Fig. 1
Virchow’s triad. Rudolph Virchow proposed three major factors that promote abnormal blood clotting
2 Epidemiology
Currently, VTE incidence for patients under the age of 18 years is roughly 1–2 per 100,000 children year [3]. By comparison, VTE incidence in adults is up to 100–150 per 100,000 persons [4] making this disorder more common in adults by almost two orders of magnitude. Nonetheless the incidence of VTE in children has been increasing over the last several years [5, 6], making it very important that pediatricians understand VTE risk factors and presenting signs and symptoms to optimize recognition and timely management of children with VTEs [7, 8]. Many factors likely contribute to the increasing incidence of VTE in children, including better awareness and improved imaging modalities, complex therapies that increase the survival of VTE-prone children, increased use of central venous catheters and increasing prevalence of modifiable VTE risk factors (e.g. obesity, physical inactivity) [9–11]. The pediatric VTE incidence peaks in infancy, decreases in early to mid-childhood and increases again in adolescence.
3 Developmental Hemostasis
Hemostasis is a highly coordinated and regulated process aimed at stopping leaks when the continuity of the vessel wall has been compromised by injury (Fig. 2). The coagulation system is kept in balance through the activities of pro-coagulant factors on one side, anticoagulant and fibrinolytic factors on the other [12].


Fig. 2
The coagulation cascade and anticoagulation factors. The coagulation cascade consists of a series of carefully regulated sequential reactions in which serine proteases cleave pro-factors to activate the next factor’s enzymatic activity. The central enzyme in the cascade is thrombin, which cleaves fibrinogen to form fibrin but also participates in the amplification loop of factors VIII and IX as well as the termination of coagulation cascade through the protein C protein S pathway. The coagulation cascade has many “built-in” regulators that serve to prevent inappropriate or excessive clot formation. Highlighted in gray are the anti-coagulation factors that when deficient or defective are known to play a role in VTE as well as the pro-coagulants that, when in excessive amounts predispose to thrombosis
The hemostatic system undergoes constant changes over the lifespan of an individual; these changes are more pronounced during the first 6 months of life, but continue nonetheless in childhood, adolescence and adult life. Many studies have been focused on the age dependent quantitative differences of clotting and antithrombotic factors. At the time of birth the levels of most clotting and antithrombotic factors are low compared to the adult values. The vitamin K dependent factors II, VII, IX and X reach only 30–40 % of adult values [13]. This explains the physiologically prolonged activated thromboplastin time (aPTT) and prothrombin time (PT). The protein C, protein S, antithrombin, plasminogen and alpha 2 antiplasmin are lower at birth and only approach the adult values after 6 month to 1 year of age. Fibrinogen is moderately decreased at birth, reaching the adult levels after 1 month. Factors V, VIII and XIII do not change with age, while von Willebrand factor is typically elevated at birth, reaches a nadir around the age of 1 year and, thereafter, its activity increases through childhood and into adult life.
Acknowledging these variations is important in the interpretation of the hemostatic tests, in the choice and management of anticoagulant and thrombolytic therapy, especially during the first year of life
Another aspect of developmental hemostasis is the age related qualitative changes of the hemostatic proteins [14]. One example is the antithrombin, the serine protease that inhibits the thrombin and activated factor X (Xa). Antithrombin is secreted in several isoforms : native, pre-latent, cleaved and latent [15]. Latent antithrombin (LAT) has been associated with thrombosis in critically ill patients but it also has strong anti-angiogenic properties. Newborns have low levels of LAT compared to the adults; this may have to do more with the need to maintain active angiogenesis, but may be a contributing factor to the relatively low rate of thrombosis in childhood.
The overall physiologic implications of the developmental changes in the hemostatic system are not entirely understood. We do know that newborn and infants have a functional hemostatic system that offers relative protection against thrombotic events: individuals with congenital deficiencies of anti-coagulant factors (protein C, protein S, antithrombin) typically do not develop thrombotic events until the second or third decade of life, and thromboses secondary to acquired risk factors, like nephrotic syndrome or orthopedic surgeries, are much less common in young children compared to older adults.
Emerging data seems to suggest that the hemostatic proteins have more complex physiologic roles in angiogenesis and wound healing, which may partially explain their age dependent variations.
4 Risk Factors
Deep venous thrombosis occurs when the hemostatic equilibrium is tilted toward clot formation. There is a variety of factors which may contribute to this disruption, some inherited, and others aquired (Table 1). The majority of pediatric patients with VTE have at least one identifiable risk factor; the truly idiopathic VTEs are uncommon in this age group. It is very important to identify the risk factors that led to the thrombotic event and determine whether they are transient or long lasting, modifiable or not modifiable through lifestyle changes, medical or surgical therapy.
Table 1
Risk factors for VTE in children
Acquired (Environmental) factors | Inherited (Genetic) factors |
---|---|
Anti-phospholipid antibody | Antithrombin deficiency |
Autoimmune disorders (IBD) | Elevated homocysteine (MTHFR mutation) |
Central venous catheters | Elevated VIII levels |
Chemotherapy (e.g. L-asparaginase) | Factor V Leiden mutation |
Dehydration | Family history of thromboembolism |
Congenital Heart disease | Protein C deficiency |
Hyperviscosity (polycythemia) | Protein S deficiency |
Immobility | Prothrombin gene mutation (G20210A) |
Infection (sepsis, cellulitis, osteomyelitis) | Vascular/anatomic abnormalities |
Inflammation | |
Lupus | |
Malignancy | |
Nephrotic syndrome | |
Obesity | |
Oral contraceptives (estrogen containing) | |
Pregnancy | |
Shock | |
Smoking | |
Surgery | |
Total Parenteral Nutrition (TPN) | |
Trauma (especially bone fractures) | |
Ventriculo-atrial shunting |
The answer to those questions help tailor the duration and sometimes intensity of anticoagulation, as well as the lifestyle changes recommend in order to prevent a recurrence [16].
Inherited risk factors which may lead to a lifelong tendency toward hypercoagulability, are commonly referred to as inherited thrombophilias [17–19]. Inherited thrombophilias should be suspected when there is a positive family history of thrombosis (DVT, PE, myocardial infarction, stroke) or recurrent pregnancy loss, particularly if such events have occurred in multiple individuals in the family, in the same individual multiple times or at particularly young ages [20]. Inherited thrombophilias should also be suspected in a newborn or child when there is no obvious explanation for the thrombosis.
Fortunately the inherited thrombophilias with the severest phenotypes are quite rare. These include homozygous, protein C deficiency and protein S deficiency [17, 21, 22]. Each of these conditions typically presents in infancy with severe thrombosis, manifested as retinal arterial thrombosis, skin necrosis or VTE [23]. Heterozygous deficiency in these factors wherein affected individuals have low levels of antithrombin, protein C or protein S may predispose to clotting later in childhood or in adulthood [11] These deficiencies are found in less than 10 % of pediatric patients with VTE [18]. More common thrombophilic risk factors include the factor V Leiden mutation and the prothrombin gene G20210A mutation [24, 25]. Factor V Leiden mutation may affect up to 5 % of the population, and raises clotting risk by 3–7 times when only one allele is mutant. The prothrombin G20210A mutation, found in the regulatory (non-transcribed) region of the prothrombin gene, leads to elevated levels of serum prothrombin which in turn favors thrombus formation. In the heterozygous state this mutation increases the risk of a first thrombotic event by 2–3 times. Other predisposing conditions include increased levels of serum homocysteine, which can result from inherited defects in the methylenetetrahydrofolate reductase (MTHFR) gene and which promotes endothelial cell injury [26, 27]. An additional inherited VTE risk factor is elevation of basal levels of circulating Factor VIII, which presumably results in excessive Xa formation to favor thrombosis [28].
Central venous catheterization represents a major risk factor for pediatric VTE, especially in newborns. These indwelling catheters, used more and more to provide supportive care for ill children, have a relatively high risk of associated clots in and around their venous location. Other “acquired” factors include either trauma or major surgery followed by bedrest, especially orthopedic involvement [29–32]. Prolonged immobilization, dehydration, infections (e.g. cellulitis, osteomyelitis, phlebitis), medications (especially L-asparaginase chemotherapy used for acute lymphoblastic leukemia), high estrogenic states (pregnancy, use of estrogen-containing hormonal contraceptives) and obesity are all important risk factors [33]. Medical conditions that predispose to VTE include congenital heart malformations and other anatomic anomalies that predispose to venous stasis such as May-Thurner syndrome wherein the left iliac vein is compressed when crossing between the right iliac artery and the lumbar vertebrae [34]. Presence of anti-phospholipid (anti-cardiolipin antibodies, beta 2 glycoprotein 1 antibodies, lupus anticoagulant), as might occur as an isolated finding or in the context of a collagen vascular disorder, place patients at heightened risk [35, 36]. Nephrotic syndrome is a well-established medical predisposing risk factor, likely due to chronic renal loss of anticoagulant proteins such as antithrombin [37]. Inflammatory states such as Crohn’s disease or rheumatoid arthritis also favor clot formation [38].
5 Clinical Presentation
Clinical signs and symptoms of VTE range from subtle to overt, therefore it is important to have a high index of suspicion and consider the diagnosis particularly in patients who carry risk factors for VTE. Most VTEs come to medical attention because of stasis, backflow and interrupted circulation caused by the clot (Table 2). Signs/symptoms of VTE in an extremity (e.g. leg) include pain, tenderness swelling and erythema. Function of the affected limb is often compromised by pain, weakness or fatigue [39]. More proximal VTEs such as might occur in the superior vena cava may result in SVC syndrome which is typified by swelling and erythema of the head and neck, prominence of jugular venous blood vessels and headache. Intracranial thrombosis (e.g. central venous sinus thrombosis) can present with acute neurologic dysfunction or headache. Signs and symptoms of pulmonary embolism include tachypnea, pleuritic chest pain, hypoxia, shortness of breath, diaphoresis and feeling of anxiety or doom [40].
Table 2
Clinical manifestations of venous thromboembolism
Deep venous thrombosis | Pulmonary embolism |
---|---|
Swelling/edemaa | Shortness of breath |
Pain or tendernessa | Anxiety |
Erythema, hyperemiaa | Chest pain |
Visible surface veinsa | Cough |
Fatigue, weakness, loss of functiona | Hemoptysis |
Headache (sinus vein thrombosis) | Tachycardia |
6 Evaluation of Suspected Thrombosis
The most reliable and useful ways in which to test for venous thromboembolism are generally through imaging studies. The imaging modality of choice varies with the location of the suspected thrombus. For DVTs of the extremities color flow Doppler ultrasound is the preferred study because it is noninvasive, does not involve radiation, does not usually require sedation and offers reliable information about blood flow through affected extremity vessels [41, 42]. Doppler ultrasounds may also be used for the evaluation of portal and renal veins, however, given their deeper location ultrasound is less reliable in these settings and may not be useful for the evaluation of the inferior vena cava or its tributaries. The right atrium and proximal portions of the superior vena cava and inferior vena cava can be visualized through echocardiography. Computerized tomography (CT) angiography and magnetic resonance venogram MRV can be used to visualize venous blood flow in the cerebral venous sinuses and deep veins in the thorax or abdomen. The contrast venogram may image central catheter -related thrombosis. Pulmonary embolism in children is best evaluated by CT angiography a test that for the most part has replaced the ventilation perfusion nuclear medicine imaging.
7 Diagnostic Laboratory Studies
There are no definitive serologic studies that are unequivocally diagnostic of VTE. Certainly, elevated D-dimers, which are produced as a consequence of fibrinolysis, can be observed in the presence of a clot. D-dimer testing has been used in adults in the diagnostic algorithms of PE, however in children with suspected PE, normal D-dimer levels lacks the same negative predictive value as in adults because at least 15 % of children with PE have a normal D-dimers [39]. In adult patients, persistent D-dimer elevation (and factor VIII elevation) for many months after diagnosis, may predict VTE recurrence. Similar data supporting this observation is available in the pediatric age group [28, 43].
8 Evaluation of Inherited Thrombophilia
There are no clear guidelines regarding which patients should be evaluated for thrombophilia. Testing of asymptomatic children is controversial and it is not supported by clinical evidence, however many hematologists agree that a thrombophilic work-up is probably indicated for the following patients:
neonates with non-catheter related VTE
adolescents with spontaneous, unprovoked VTE
children with VTE who have a family history of thrombosis or inherited thrombophilia
children with recurrent thrombosis
Levels of antithrombin, protein C and protein S must be put into context for proper interpretation. Thus, each is physiologically lower than adult levels for the first several months of life, and each may be consumed during acute thrombotic events. For this reason, it is important to determine basal antithrombin, protein C and protein S levels after infancy and several months after the VTE diagnosis. Various medications are also known to confound natural anticoagulant levels. Thus, antithrombin levels may be influenced by heparin therapy, and protein C and S levels will be lowered by warfarin or other vitamin K antagonists. Functionally, some clot-based assays for protein C and S activity may be influenced by the factor V Leiden mutation, factor VIII levels or the presence of the lupus anticoagulant [11, 44, 45].
With the exception of uncommon homozygous deficiency for protein C or protein S, the results of the thrombophilia evaluation may not influence the immediate management of the VTE. Homozygous protein C or protein S deficiency typically presents in the newborn period with extensive thrombosis and necrotic skin lesions of purpura fulminans and warrants prompt replacement therapy in addition to anticoagulant therapy [46]. However, such cases are quite rare, and for the usual pediatric VTE patient, the thrombophilia evaluation may provide information about the risk of recurrence and guide preventative interventions.
9 Therapy
Most of the clinical data used in the treatment algorithms for children with VTE has been derived from adult clinical trials. While this approach has resulted in advances in the treatment and survival for pediatric VTE, some adult data cannot be immediately extrapolated to children because of age-related physiologic differences in the hemostatic system and differential responses to anticoagulant or thrombolytic medications [47, 48].
Goals of VTE therapy are to inhibit clot extension, prevent embolism, restore venous patency, limit long term sequelae and reduce risk of VTE recurrence [49]. These are achieved by recognizing and modifying risk factors and by pharmacologically restoring hemostatic balance with anticoagulant or thrombolytic therapies.
Anticoagulant or thrombolytic therapies carry a real risk of bleeding, therefore risks and benefits must be carefully taken into account in order to determine how aggressive to be. Several factors should be taken into account when choosing initial therapy for a newly-diagnosed VTE:
Urgency of the clinical situation. Medically severe clots such as large PEs causing hemodynamic instability or a large VTEs risking organ or limb compromise call for aggressive intervention with the goal of rapidly resolving the thrombus. In addition to anticoagulation, thrombolysis should be considered in such situations.
Bleeding risks of the intervention. Anticoagulation and thrombolysis each carry a risk of bleeding, and bleeding risk is highest for patients with evidence of bleeding such as intracranial hemorrhage, gastrointestinal hemorrhage, in patients whose vasculature has recently been challenged by surgery or trauma or in patients with preexisting bleeding tendencies (e.g. thrombocytopenia, coagulopathies, prematurity). In such patients, the risks of aggressive medical interventions such as systemic thrombolysis may outweigh the benefits for VTE resolution.
Likelihood of long-term sequelae. Large, obstructive thrombi in the lower extremities and IVC carry a high risk of post-thrombotic syndrome (PTS) which is manifested by chronic pain, swelling, and skin abnormalities [50]. Several adult and pediatric series suggest that thrombolysis may be superior to anticoagulation alone in preventing the development of PTS.
Expertise and proficiency of the medical team. Highly specialized interventions such as catheter-directed thrombolysis require the coordinated actions of interventional radiologists or cardiologists, hematologists, intensivists and nursing care. Given the relative rarity of pediatric VTE requiring such therapies and the proficiency that comes only from treating such patients in adequate volume, it may be difficult to amass such expertise in any given hospital, especially for the care of neonates and young children [51].
10 Anticoagulant Therapy
Anticoagulant therapy reduces the risk of thrombus extension and embolism while allowing the natural fibrinolytic mechanism to gradually reduce the size of the clot. Duration of anticoagulant therapy is mainly based on adult studies. If a causative factor can be identified and is reversible (e.g. removal of a central venous catheter), one may consider a shorter course of anticoagulation [49]. If, however, causative factors cannot be removed, for example in the setting of an underlying thrombophilia, then a longer course of anticoagulation may be indicated [52]. Similarly, resolution of the thrombus on imaging along with normalization of D-dimer and factor VIII levels favors a shorter course of anticoagulant therapy [28, 53]. On the other hand, if VTE recurs, then this warrants long term anticoagulation.
Guidelines from the American College of Chest Physicians (AACP), which many clinicians consider as generally-accepted standard of care for patients with VTE, call for anticoagulation with heparin or low molecular weight heparin followed by a vitamin K antagonist for three months for a first-time VTE if the causative factor can be identified and resolved. Similarly, six to twelve months of anticoagulation are recommended for an unprovoked VTE. For recurrent VTEs, the recommended duration of anticoagulation is three months for VTE when a causative factor can be identified and resolved and lifelong for idiopathic, unprovoked VTE [54].
11 Anticoagulant Medications
Unfractionated heparin (UFH) acts predominantly by enhancing the inhibitory action of antithrombin on thrombin, factors Xa, IXa and XIa. UFH may be administered subcutaneously or intravenously and has a relatively short half- life (30–60 min), particularly with IV administration, which makes anticoagulation quickly reversible should a bleeding complication, trauma or need for surgery develop. The anticoagulant activity of heparin can be monitored using the activated partial thromboplastin time (aPTT) or the anti- activated factor X activity (anti-Xa). Pediatric therapeutic ranges, for the most part, are extrapolated from adult VTE studies. Since aPTTs can be higher at baseline for infants, it is difficult to define a therapeutic range for heparin in pediatrics based solely on aPTT [55]. For this reason, and because the physiologic effects of Xa inhibition are consistent across the pediatric age range, the anti-Xa test is widely considered to be a more reliable test to monitor heparin anticoagulation level in infants and children. In general, the dose of UFH should be titrated to establish an anti-Xa therapeutic range of 0.35–0.7 U/ml. Heparin works in conjunction with antithrombin, and will therefore have greatest anticoagulant effect when antithrombin levels are normal. Newborns have physiologically lower antithrombin levels and therefore may require higher UFH doses. The starting therapeutic dose for UFH, when used as a continuous infusion is 28 units/kg for neonates and 18–20 units/kg for children older than 1 year. The dose should be titrated to the desired level of anticoagulation. Bolus infusions of 75–100 U/kg UFH shortens the time to achieving the therapeutic range but may increase risk of bleeding especially in premature newborns [56]. The main risk associated with heparin use is bleeding, with estimates of major bleeding varying between 1.5 and 24 %, depending on the population and clinical setting [57]. Heparin-induced thrombocytopenia (HIT), an acquired antibody-targeted reaction against heparin that activates platelets and paradoxically increases thrombotic risk, is one of the most serious consequences of UFH therapy [58, 59]. Nonetheless, the risk of developing HIT is relatively low (1–2.3 %). Long-term heparin use may be associated with risk of bone calcium wasting and osteoporosis, but only a few cases have been reported in children. If reversal of heparinization is required quicker than can be achieved by simply discontinuing UFH, protamine can be used. Protamine is thought to antagonize heparin’s anti-IIa activity but not its anti-Xa activity.
Low molecular weight heparin (LMWH), like UFH, acts through the antithrombin pathway, but exerts a comparatively stronger inhibitory effect on activated factor X (Xa). Enoxaparin, the most commonly used LMWH in children, can be administered subcutaneously and has a more predictable dose–response relationship than UFH [60]. LMWH also has a longer half-life than UFH, roughly 12 h, allowing less frequent dosing but at the cost of less reversibility [61]. LMWH is cleared predominantly renally, therefore it should be used with caution in individuals with renal insufficiency. The anticoagulant activity of LMWH is usually measured by anti-Xa activity 3–5 h after subcutaneous administration. The therapeutic range for anticoagulation is generally between 0.5 and 1 U/ml and less for thrombosis prophylaxis (typically 0.25–0.5 U/ml). The beginning dose for enoxaparin is usually 1 mg/kg administered subcutaneously every 12 h. Because of enhanced clearance of the drug, newborns generally require larger doses per kilogram, so for infants the starting enoxaparin dose is typically 1.5 mg/kg administered subcutaneously every 12 h. Obese children may require less enoxaparin per kg. of body weight to achieve targeted anticoagulation levels [62]. Anti-Xa levels should be checked after the second or third dose, and LMWH dosing titrated to the targeted anti-Xa level [57]. Once therapeutic levels are achieved, periodic monitoring of anti-Xa activity is recommended, especially in young infants, who experience rapid weight gain and changing LMWH requirements per kilogram of body weight. The main risk of LMWH therapy is bleeding, with different authors reporting rates of major bleeding between 2.9 and 5 %. The anticoagulant activity of LMWH, like that of UFH, can be partially reversed using protamine. Osteoporosis and HIT are thought to be less frequent complications of LMWH than of UFH, but their incidence and severity in children remain uncharacterized [60].
Vitamin K antagonists antagonize the post-translational γ-carboxylation reaction needed for the activation of coagulation factors II, VII, IX and X. Importantly, γ-carboxylation is also needed for the synthesis of functional protein C and S which have a shorter half-life than that of II, IX or X [63]. Thus, vitamin K antagonists are administered along with heparin or LMWH for 4–6 days until a therapeutic INR is achieved; this approach prevents the development of a transient hypercoagulable state through the inhibition of protein C and protein S activity [57]. The most commonly used vitamin K antagonist is warfarin (Coumadin©). In children, starting doses of warfarin are usually in the range of 0.1 mg/kg. day, but therapeutic doses must be titrated to desired effect. Since warfarin antagonizes vitamin K activity, its anticoagulant effects are susceptible to dietary vitamin K intake. For this reason, patients should be advised not to alter their dietary intake of vitamin K rich foods (e.g. greens, leafy vegetables) once an appropriate therapeutic level of warfarin has been achieved [64]. Anticoagulant activity is measured by the prothrombin time (PT) / international normalized ration (INR), which is standardized between laboratories. The therapeutic range for most patients, extrapolated from adult studies, is an INR between 2 and 3, higher in certain high risk situations like artificial heart valves. In general, warfarin is not used in newborns and young infants because of difficulty in keeping such patients in the therapeutic range. Newborns have physiologically low levels of vitamin K dependent factors, they may have variable intake of vitamin K (low in breast milk, high in formula and in total parenteral infusions), and blood sampling for INR monitoring is often difficult for premature babies and low birth weight infants. Furthermore, there are no liquid formulations for warfarin, which challenges administration for very young patients. As with other anticoagulants, the main risk of warfarin therapy is bleeding, with major bleeding risk estimated at around 3 % per patient per year. Proper and continued education of the patient and family in managing of this medication and activity restrictions are important ways to minimize bleeding risk. Given the variations in the vitamin K levels, it is important to monitor INRs at regular intervals) while patients are on warfarin. Should bleeding or trauma occur, warfarin’s anticoagulant effects may be reversed using vitamin K, fresh frozen plasma infusions, prothrombin protein concentrates or recombinant activated factor VII. Lastly, warfarin is well-known teratogen and is contraindicated in pregnancy. For this reason, teenage girls that must be on warfarin should receive appropriate counseling and ideally be on contraceptive therapy.
12 Newer Anticoagulants
Because of the challenges that come with heparin and warfarin therapy, the use of other anticoagulants has become more prevalent in children. Argatroban is a direct thrombin inhibitor approved for pediatric use in the United States for treatment of patients with heparin induced thrombocytopenia (HIT). It is administered as a continuous IV infusion and its anticoagulant activity monitored with the aPTT (therapeutic target aPTT 1.5–3 times normal); the steady state is reached after 2 h. of intravenous infusion. Argatroban is cleared by the liver and therefore should be used with caution in patients with hepatic dysfunction [65].
The direct thrombin inhibitor dabigatran is approved for adult use, and the pediatric experience is limited to small case series. A phase I clinical trial (NCT00844415) done in adolescents showed that drug was well tolerated, with no major bleeding events; there are ongoing phase 2 and phase 3 pediatric clinical trials [66, 67].
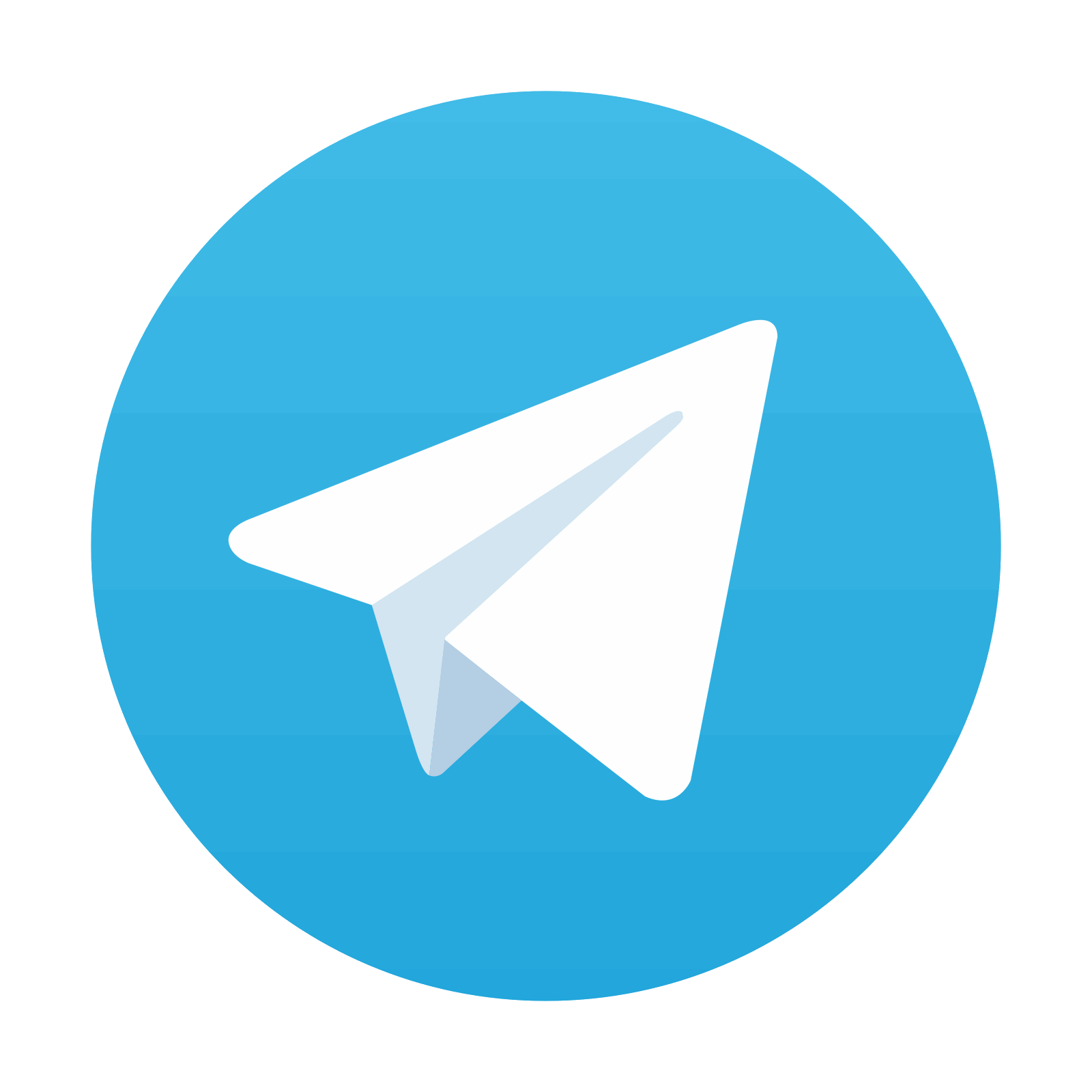
Stay updated, free articles. Join our Telegram channel

Full access? Get Clinical Tree
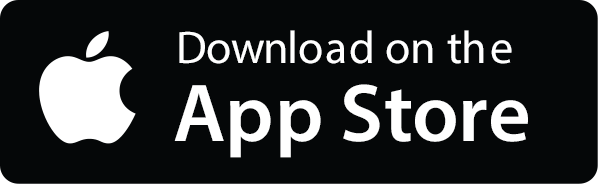
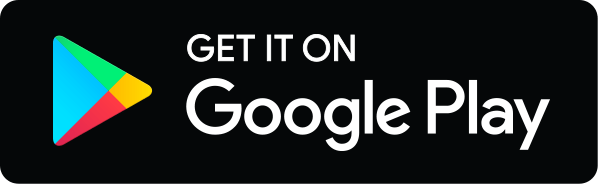