Fig. 6.1
The effect of elevated progesterone on conceptus elongation. Transient elevation of peripheral progesterone concentrations by exogenous administration results in the acceleration of conceptus development. Photographs illustrate elongating bovine conceptuses from the uterus of a recipient heifer with elevated (top) or low (bottom) serum progesterone, recovered at slaughter on day 14 post-estrus following the transfer of 10 in vitro-produced blastocysts on day 7. Such elongation is entirely maternally driven, as evidenced by the failure of embryos to elongate appropriately in vitro or in vivo in the absence of uterine glands. Note, however, the variation in conceptus length, despite being recovered from the same uterus, suggesting an intrinsic component to the embryo in the elongation process (Modified from Clemente et al. 2009).
6.2 Importance of Progesterone in the Establishment of Pregnancy
Circulating concentrations of P4 represent a balance between the production of P4 by the corpus luteum (CL) and the metabolism of P4, primarily by the liver. The production of P4 is regulated by the development of the CL after the preovulatory surge of luteinizing hormone, the number of granulosa cells that luteinize into large luteal cells, and the constitutive production of P4 by these cells. The metabolism of P4 is primarily related to the rate of blood flow to the liver (Sangsritavong et al. 2002). Therefore, practical strategies aimed at the manipulation of circulating concentrations of P4 will be most productive by focusing on increasing luteal tissue volume to increase P4 production and/or limiting P4 metabolism (Wiltbank et al. 2014).
While fertilization success following natural or artificial insemination is high (~90 %), a significant proportion of the resulting embryos fail to develop to term. The majority of embryos are lost between fertilization and maternal recognition of pregnancy, which in cattle occurs around day 16 post-estrus (Diskin and Morris 2008), and this loss significantly contributes to reproductive inefficiency in cattle. While poor embryonic survival can be attributed to a variety of factors such as poor follicle and oocyte quality and poor embryo quality and/or poor reproductive tract environment, circulating concentrations of P4 undoubtedly play a role in establishing uterine receptivity and low concentrations, for example, in high-producing dairy cows, may partly contribute to lower fertility in such animals.
Results of several retrospective studies have indicated a positive relationship between circulating concentrations of P4 in the week after breeding and subsequent pregnancy rate (Stronge et al. 2005; Diskin et al. 2006; Parr et al. 2012). Interestingly, there is both a linear and quadratic component to this relationship; that is, too much P4 may lead to a decline in pregnancy rate. Thus, both suboptimal and supraoptimal concentrations of P4 from days 4–7 after AI or a suboptimal rate of increase in the concentration of P4 during this interval is negatively associated with embryonic survival. Using a novel model of high- and low-fertility Holstein-Friesian cows, Cummins et al. (2012) reported that circulating concentrations of P4 were 34 % greater in cows with similar genetic merit for milk production traits, but with extremes of good (Fert+) or poor (Fert-) genetic merit for fertility traits. In a follow-up study, Moore et al. (2014) investigated the factors affecting circulating concentrations of P4 in those cows. Concentrations of P4 were measured from days 1–13. Corpus luteum volume was 41 % greater and mean circulating concentrations of P4 were 79 % greater in Fert + cows compared with Fert- cows. The results indicate that greater circulating concentrations of P4 were primarily due to a greater capacity of CL to secreted P4 rather than differences in the clearance rate of P4 in this lactating cow genetic model of fertility.
6.3 Effect of Progesterone on the Pre-hatching Embryo
As discussed elsewhere in this chapter, the effect of P4 on conceptus development is likely a result of downstream effects of P4-induced changes in gene expression in cells of the uterus (Satterfield et al. 2006; Forde et al. 2009, 2011a) resulting in changes in the composition of uterine lumen fluid or histotroph to which the developing embryo is exposed. Whether any of the effects of P4 are directly on the embryo has been assessed by experiments in which P4 was added to medium during the in vitro culture of embryos. Results of such studies have been varied and contradictory with some authors reporting positive effects of P4 (Merlo et al. 2007; Ferguson et al. 2011), while others have reported no effect (Reggio et al. 1997; Goff and Smith 1998). Overall, however, despite the presence of progesterone receptor (PGR) mRNA on embryos (Clemente et al. 2009), there is little convincing evidence that P4 has a direct effect on the early embryo. In our own laboratory, culture of embryos in vitro in the presence of P4 did not affect the proportion developing to the blastocyst stage in the presence or absence of oviductal epithelial cells (Clemente et al. 2009). This is consistent with the observations of Larson et al. (2011) who failed to observe a direct effect of P4 either from days 1–3 or 4–7 after fertilization. Furthermore, the addition of P4 to culture medium had no effect on conceptus elongation after transfer to synchronized recipients (Clemente et al. 2009). In two other in vivo studies, we failed to demonstrate the effect of elevated P4 on blastocyst development. In the study of Carter et al. (2008), no differences in embryonic development on day 5 or day 7 were observed when beef heifers were supplemented with exogenous P4 from day 3, despite dramatic effects on post-hatching elongation between days 13 and 16 of pregnancy. In a follow-up study, multiple in vitro-produced embryos were transferred to the oviduct of beef heifers that did or did not receive a P4 insert on day 3 after the onset of estrus. There was no effect of P4 on the proportion of embryos that developed to the blastocyst stage by day 7 (Carter et al. 2010).
6.4 Effect of Progesterone on the Post-hatching Blastocyst
In contrast to the lack of a requirement by the embryo for interactions with the female reproductive tract up to the blastocyst stage, the development of the post-hatching and preimplantation conceptus is entirely driven by the uterine environment. The protracted period of implantation characteristic of ruminants involves rapid proliferation of the trophectoderm cells, which is dependent on substances in the uterine lumen fluid (or histotroph), that are derived from the endometrium, particularly the uterine glands, for growth and development.
Earlier studies in ewes (Wilmut and Sales 1981; Lawson and Cahill 1983) and cows (Garrett et al. 1988b) suggested that maternal P4 regulates early conceptus growth and development. More recent studies confirmed those findings and have begun to unravel the underlying biology (e.g., Satterfield et al. 2006; Forde et al. 2009, 2011a, 2012). In particular, significant progress has been made in clarifying the role of luteal P4 in the successful establishment of pregnancy in sheep and cattle, with particular emphasis on how P4 affects endometrial gene expression and conceptus elongation.
The effects of elevated P4 shortly after conception on the advancement of conceptus elongation have been convincingly demonstrated in cattle and sheep. Garrett et al. (1988b) administered 100 mg P4 on days 1, 2, 3, and 4 of pregnancy which increased concentrations of P4 in peripheral plasma on days 2–5 and significantly larger conceptuses on day 14. Using a P4 implant on day 3 of pregnancy, Carter et al. (2008) significantly elevated concentrations of P4 in plasma until day 8, and this was associated with larger conceptuses recovered at slaughter on day 16. Similarly, when ewes received daily injections of 25 mg P4 from 36 h postmating, blastocyst diameter increased by 220 % on day 9 and at the time of initiation of elongation of blastocysts to a filamentous conceptus on day 12 was advanced (Satterfield et al. 2006); these effects of P4 treatment on blastocyst development were blocked by the administration of RU486, a PGR antagonist.
Using a combination of in vitro embryo production and in vivo embryo transfer techniques, we have shown that the effect of P4 on conceptus development is mediated exclusively via the endometrium (Clemente et al. 2009). The addition of P4 to culture medium had no effect on blastocyst formation (Clemente et al. 2009; Larson et al. 2011) or elongation after transfer to synchronized beef recipients (Clemente et al. 2009). Exposure of the uterus to elevated P4 prior to embryo transfer resulted in an advancement in conceptus elongation (Clemente et al. 2009). Most convincingly, the embryo did not need to be present in the uterus during the period of P4 elevation in order to benefit from it (Clemente et al. 2009), strongly suggesting that the effect of P4 is via advancement of the normal temporal changes that occur in the endometrial transcriptome (Forde et al. 2009) resulting in advanced conceptus elongation (Carter et al. 2008; Clemente et al. 2009). In addition, reducing the output of P4 from the CL, for example, by treatment with prostaglandin F2α (PGF) (Beltman et al. 2009; Forde et al. 2012) or by aspirating the contents of the preovulatory follicle just before the expected time of ovulation (O’Hara et al. 2012), results in a delay in the temporal changes in the endometrial transcriptome, causing delayed conceptus elongation in vivo (Forde et al. 2011b, 2012; O’Hara et al. 2012).
6.5 Effects of Progesterone on the Endometrium and Uterine Environment
A prerequisite for establishing uterine receptivity to implantation in all species studied thus far is loss of expression of PGR from uterine LE and then GE (Bazer et al. 2010). Paradoxically, it is sustained exposure of the endometrium to circulating concentrations of P4 that leads to this downregulation of PGR as the luteal phase of the estrous cycle progresses. The concentrations of P4 in circulation modify the loss of expression of PGR in the endometrium such that in animals in which P4 is high, there is early loss of the PGR (Okumu et al. 2010), i.e., uterine receptivity to implantation is established earlier, while conversely, low or suboptimal concentrations of P4 delay loss of the PGR and thus delay establishing uterine receptivity to implantation (Forde et al. 2011a). Thus, in simple terms, it would appear that elevating P4 simply advances the changes in endometrial gene expression which normally occur (Forde et al. 2009) (Fig. 6.2).
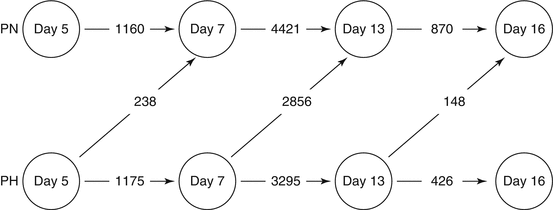
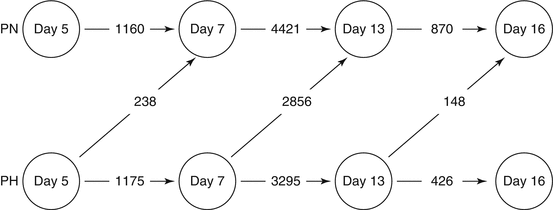
Fig. 6.2
Numbers of differentially expressed genes that are temporally regulated in the endometrium of pregnant heifers with unmanipulated (“normal,” PN) or artificially elevated (“high,” PH) progesterone concentrations between day 5 and day 16. Note that a day 5 endometrium exposed to elevated progesterone is more similar to a “normal” day 7 endometrium than the corresponding day 5 endometrium. The same applies for day 7 and day 13, indicating that P4 supplementation advances the temporal changes in gene expression which normally occur in the endometrium and which contribute to advanced conceptus development observed on day 13 and day 16 (From Forde et al. 2009).
The continued exposure of the endometrium to P4 during the luteal phase of the estrous cycle significantly modifies the endometrial transcriptome (Forde et al. 2009, 2011b, 2012) with a large divergence in gene expression in the endometrium (and histotroph composition) between when a zona-enclosed blastocyst (when PGRs are expressed in uterine LE and GE) andan elongated conceptus (when PGR expression is lost from uterine LE and GE) is present. Manipulating circulating concentrations of P4 in vivo significantly alters the expression of genes that encode for secreted proteins in the endometrium, e.g., apolipoprotein A1 (APOA1), connective tissue growth factor (CTGF), lecithin-cholesterol acetyltransferase (LCAT), lipoprotein lipase (LPL), insulin-like growth factor binding proteins 1 (IGFBP1) and 3 (IGFBP3), fatty acid binding protein 3 (FABP3), fibroblast growth factor 10 (FGF10), gastrin-releasing peptide (GRP), mephrin 1B (MEP1B), matrix Gla protein (MGP), neuromedin N (NMN), nephronectin (NPNT), periliphin 2 (PLIN2), and tubulointerstitial nephritis antigen-like 1 (TINAGL1) (Satterfield et al. 2009; Forde et al. 2010, 2013), which are also detectable in the uterine luminal fluid during elongation of the conceptus (Forde et al. 2013) and the pregnancy recognition period (Forde et al. 2014a). Thus, P4, during the luteal phase of the cycle, modifies the uterine environment in a stage-specific manner to provide the optimum environment for the developing conceptus. The differences in the capacity of the uterus to induce elongation of the conceptus are dependent on circulating concentrations of P4 and effects of P4 on the endometrial transcriptome and subsequent modification of the uterine luminal fluid, resulting in either an advance or a delay in conceptus elongation. As a consequence of the different elongation trajectories driven by different concentrations of P4 in circulation, there is a difference in IFNT secretion (Kerbler et al. 1997; Rizos et al. 2012) and thus effects on the pregnancy recognition response in the endometrium. Results from studies of both cattle and sheep demonstrated that stimulation of classical interferon-stimulated genes in uterine GE and stromal cells, but not uterine LE, is greater in those animals in which P4 advanced the pregnancy recognition response in the endometrium.
In addition to proteins, the uterine lumen fluid of ruminants is composed of molecules that are actively transported from the endometrium into the uterine lumen and include glucose, ions, fatty acids, and amino acids (Gao et al. 2009c; Groebner et al. 2011; Meier et al. 2011). In both sheep and cattle, a significant increase in total amino acid content occurs between the post-hatching period of embryo development and the pregnancy recognition period (Gao et al. 2009c; Forde et al. 2014b). The expression of the various cationic, acidic, and neutral amino acid transporters responsible for the transport of these amino acids into the uterine lumen is altered in a temporal and cell-specific manner in both the endometrium and conceptus of sheep during early pregnancy (Gao et al. 2009a, b, c) and is modulated by P4 and/or conceptus IFNT, prostaglandins, and cortisol in vivo (Dorniak et al. 2011, 2012, 2013) with additional data from cattle indicating pregnancy recognition also modifies the expression of these genes in the endometrium (Forde et al. 2014b). The abundance of individual amino acids in the uterine lumen fluid is also modified by circulating concentrations of P4, specifically alanine, arginine, asparagine, and lysine in sheep (Satterfield et al. 2010) and valine (Hugentobler et al. 2010), histidine, and asparagine in cattle (Mullen et al. 2014). These differences are likely due to the modification in endometrial gene expression of their transporters (the solute carrier superfamily of genes, SLCs) by which altering P4 in circulation modifies the expression of acidic (SLC1A1, SLC1A4, SLC1A5), cationic (SLC7A1, SLC7A5, and SLC7A7), as well as neutral (SLC38A2, SLC38A4, SLC38A7, SLC43A2, SLC6A14) amino acid transporters in the endometrium (Forde et al. 2014b).
6.6 Asynchronous Embryo Transfer
The dramatic regulatory effect of the uterus on bovine conceptus development, and the role played by P4, is nicely illustrated in studies comparing the outcome of synchronous and asynchronous embryo transfer. Such synchrony between the needs of the developing embryo and uterine secretions has long been recognized as being critical to the successful establishment of pregnancy (reviewed by Pope 1988). Indeed, embryo transfer studies in sheep and cattle have clearly demonstrated a need for close synchrony between the embryo and the uterine environment of the recipient. Previous studies have established that pregnancy rates are reduced when embryos are greater than 48 h from synchrony with the recipient’s uterine environment (Moore and Shelton 1964; Rowson and Moor 1966; Rowson et al. 1972).
Asynchronous transfer of day 7 bovine blastocysts to the uteri of day 5 or day 9 recipients resulted in retarded (5.4 ± 0.4 mm) or advanced (50.4 ± 5.2 mm) conceptuses on day 14, respectively, compared to synchronous controls (day 7 to day 7: 15.7 ± 1.5 mm) or conceptuses derived from AI (12.0 ± 3.3 mm) (Ledgard et al. 2012). Consistent with these observations, Geisert et al. (1991) reported that only 1 of 21 (4.8 %) day 8 bovine blastocysts transferred to a day 5 uterus established pregnancy compared to 50 % in synchronous controls.
The administration of P4 early in the estrous cycle of the recipient can effectively advance uterine receptivity for the transfer of older asynchronous embryos. In sheep, day 6 recipients after early exposure to exogenous P4 supported the development of transferred day 10 blastocysts (Lawson and Cahill 1983). In cattle, embryo transfer to P4-treated recipients (100 mg/day from day 1 to day 4) which showed estrus 72 h after the donor cows (i.e., day 8 blastocysts transferred into a day 5 uterus) resulted in pregnancy rates at day 35 similar to those of synchronous (±12 h) recipients (42.1 vs. 50 %), while, as mentioned above, only approximately 5 % of day 5 asynchronous recipients became pregnant (Geisert et al. 1991).
Similar data have been reported recently by Randi et al. (2015) who transferred multiple day 7 bovine blastocysts to synchronous (day 7) or asynchronous (day 5 or day 9) recipients (n = 10 per recipient). The transfer of day 7 blastocysts to a day 5 uterus resulted in fewer conceptuses surviving (20 %) and delayed elongation in those that were recovered. In contrast, transfer to an advanced day 9 uterine environment resulted in the same level of survival as synchronous controls (~50 %), but a dramatic advancement in conceptus elongation, in agreement with the observations of Ledgard et al. (2012). Supplementation of day 5 recipients with P4 from day 3 increased circulating concentrations of P4 and increased conceptus length compared to day 5 controls; however, supplementation with P4 reduced the length of estrous cycles in approximately 50 % of heifers (see below for further discussion).
Together, these studies indicate that P4 stimulates changes within the uterine environment which regulate receptivity and promote embryo survival and conceptus elongation. Manipulating P4 may be one way of strategically regulating the temporal changes that normally occur in the uterine environment in order to allow flexibility in the timing of embryo transfer. Given the above results indicating that transfer to an advanced uterus (i.e., uterus ahead of the embryo), which has had longer exposure to P4, results in an advancement in conceptus elongation and that such advanced conceptuses produce more IFNT (Kerbler et al. 1997; Rizos et al. 2012), one could reasonably hypothesize that transfer to an advanced uterus would result in improved pregnancy rates. However, interrogation of data from commercial embryo transfer operations does not support that hypothesis (Wright 1981; Donaldson 1985; Hasler et al. 1987; Heyman 1988; Hasler 2001; Rodrigues et al. 2003; Randi et al. 2015). For example, in the study of Randi et al. (2015), 4749 recipients received a single in vitro-produced fresh blastocyst. The overall pregnancy rate was 43.5 %, which is about the norm in such commercial IVF operations. The transfer of a day 7 blastocyst to a synchronous day 7 uterus resulted in a pregnancy rate of 47.3 %. Transfer to a uterus 1 day behind (day 6: 46.6 %) did not affect pregnancy rate. However, transfer to a day 5 (40.8 %) or a day 8 (41.3 %) uterus moderately impacted pregnancy rate, while transfer to a uterus 2 days in advance (day 9: 24.4 %) or 3 days behind (day 4: 27.0 %) dramatically reduced pregnancy rates compared to results from synchronous transfer of blastocysts. Taking the results of all of these studies together, it is clear that the accelerated conceptus elongation associated with the transfer of a blastocyst to an advanced uterus does not translate into an improved pregnancy rate; rather, once synchrony is exceeded by approximately 48 h, pregnancy rates decline appreciably.
6.7 Strategies to Increase Concentrations of Progesterone
Potential beneficial effects of exogenous P4 supplementation on fertility have been acknowledged for a long time (see reviews by Inskeep 2004; Lonergan 2011; Wiltbank et al. 2014). Given the significant volume of data indicating that elevating P4 results in an advancement in conceptus elongation (Garrett et al. 1988b; Carter et al. 2008; O’Hara et al. 2012; O’Hara et al. 2014a, c) and that such advanced conceptuses produce more IFNT (Kerbler et al. 1997; Rizos et al. 2012), one could reasonably hypothesize that such advanced conceptuses would be more likely to establish pregnancy. However, data on the impact of post-insemination supplementation of P4 on pregnancy rate are conflicting and, at best, indicate a modest positive response (Nascimento et al. 2013; Wiltbank et al. 2014).
Several approaches can be taken to increase concentrations of P4 in peripheral blood after AI, including those that (1) increase endogenous function of the existing CL (e.g., strategies which promote growth of the dominant follicle before ovulation, resulting in a larger CL, or luteotrophic treatments which stimulate CL development); (2) induce ovulation of a dominant follicle and formation of accessory CL (e.g., hCG or GnRH administration); or (3) those which supplement P4 directly (e.g., via injection or intravaginal devices). However, results in terms of pregnancy rate are often conflicting or inconclusive and may reflect (1) the timing of treatment, (2) that only a proportion of animals with inherently low P4 benefit from such treatment, (3) that P4 supplementation is less effective in high-producing dairy cows due to increased liver metabolism, or (4) the lack of sufficient animal numbers and statistical power in many studies to detect effects of treatments.
Dominant follicle size is associated with subsequent CL size (Vasconcelos et al. 2001). Larger CL generally secretes more P4 and this has, in some studies, been associated with improved pregnancy rates. Therefore, strategies which promote growth of the dominant follicle before ovulation and/or stimulate CL development are likely to increase pregnancy rate (Baruselli et al. 2010). Equine chorionic hormone (eCG) incorporated into synchronization protocols has been reported to improve pregnancy rates following fixed-time artificial insemination/embryo transfer, although results for treatment of lactating dairy cows have been less promising than those for heifers or beef cows (Bo et al. 2011).
Human chorionic gonadotrophin (hCG) administration to ovulate a dominant follicle and form an accessory CL has been used widely in an attempt to improve pregnancy rates, albeit with variable results (see Lonergan 2011). In a recent large study, Nascimento et al. (2013) reported the results of two separate analyses that evaluated the effect of hCG treatment post-AI on fertility in lactating dairy cows. The first was a meta-analysis of the combined results of 10 different published studies that used hCG treatment between days 4 and 9 post-AI in lactating dairy cows. Overall, hCG administration increased pregnancies per artificial insemination by 3 percentage points [34 % (752/2,213) vs. 37 % (808/2,184)]. In a subsequent field trial, lactating Holstein cows (n = 2,979) from six commercial dairy herds received hCG or not on day 5 after a timed AI; pregnancies per AI were greater in cows treated with hCG (40.8 %; 596/1,460) than control (37.3 %; 566/1,519) cows. Surprisingly, the positive effect of hCG was restricted to first-lactation cows.
6.8 Effect of Exogenous Progesterone on Corpus Luteum Life Span
Although the use of exogenous P4 to improve synchrony of embryo transfer and/or advance conceptus elongation as described previously is encouraging, caution is warranted. Paradoxically, depending on the timing of administration, exogenous P4 can have a negative effect on CL life span, resulting in shortened interestrous intervals due to premature CL regression (Ginther 1970; Garrett et al. 1988a; Burke et al. 1994; Pope et al. 1995; O’Hara et al. 2014a; Pugliesi et al. 2014; Randi et al. 2015) while at the same time advancing conceptus development due to the changes induced in the endometrium by P4 (O’Hara et al. 2014a). This situation is clearly not compatible with successful maintenance of pregnancy. In one recent study, Parr et al. (2014) supplemented lactating cows on seven farms with a P4 device from day 4 to day 10; in each farm, pregnancy rate was depressed, presumably due to animals short-cycling.
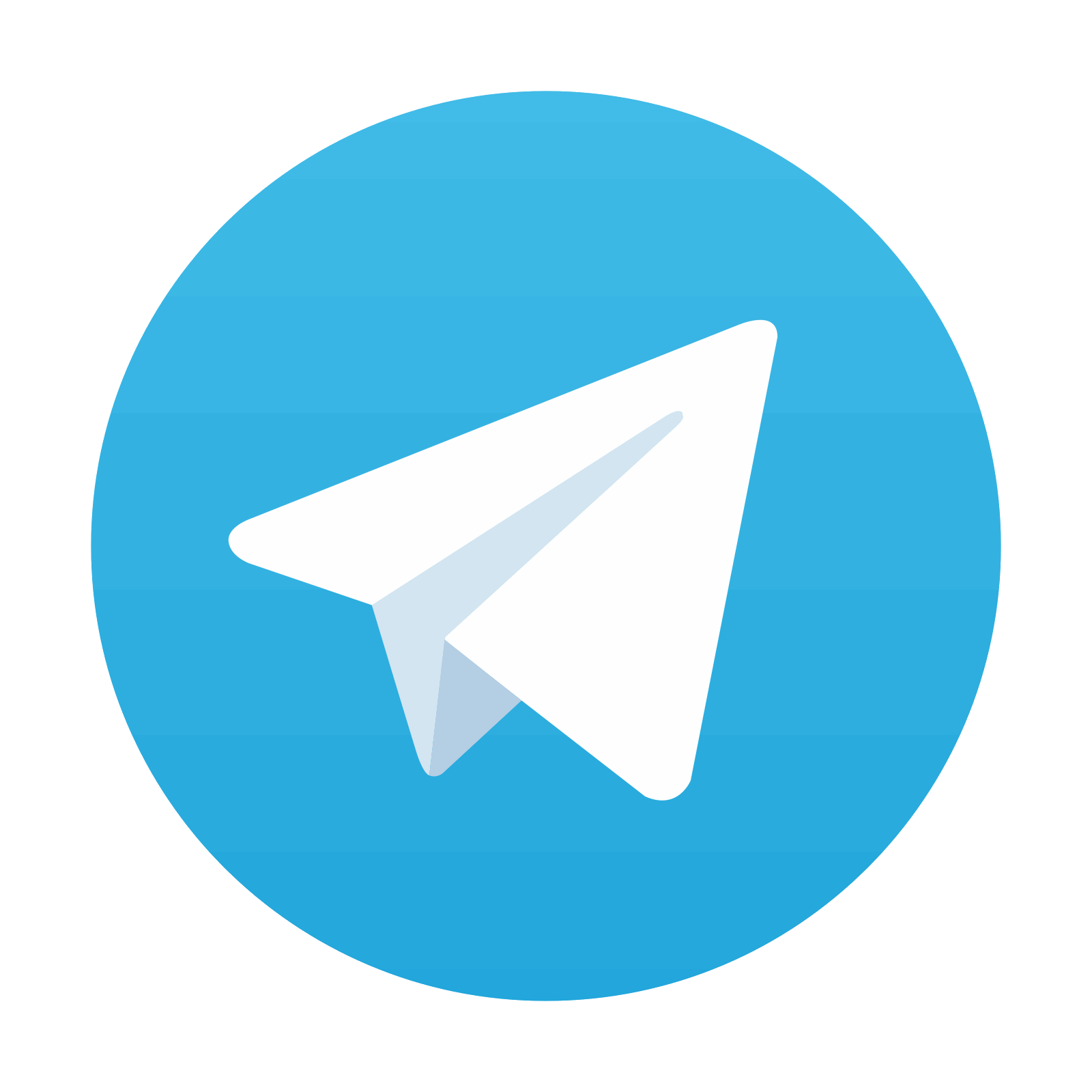
Stay updated, free articles. Join our Telegram channel

Full access? Get Clinical Tree
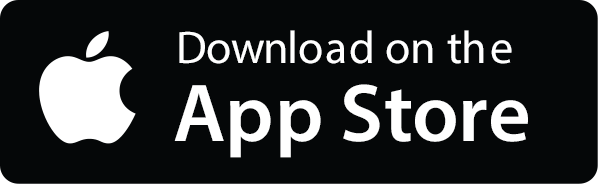
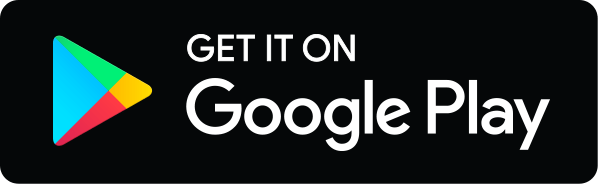