3 The care of people with cancer requires careful deliberation and consultation with the patient. The appropriate care will depend upon the prognosis, the effectiveness and toxicity of any therapy and finally, most importantly, on the patient’s wishes. To empower patients to participate in this decision-making process requires them to be fully informed and the clear delivery of this information is essential. A number of resources are available to supplement the information divulged by clinicians to their patients. These include many web-based resources as well as patient information leaflets published by charities including Macmillan and Cancer Research UK (CRUK) as well as individual tumour-type patient groups such as Breast Cancer Breakthrough and Prostate Cancer UK. It is increasingly appreciated that the management of patients with cancer requires a multidisciplinary approach involving a team of professionals including surgeons, clinical and medical oncologists, palliative care physicians, radiologists, histopathologists, specialist oncology and palliative care nurses, clinical psychologists, counsellors, dieticians, occupational therapists, physiotherapists, social workers and clinical geneticists. The aims of therapy should be clearly identified before embarking on a course of treatment. Treatment may either be curative, aiming to prolong the quantity of life or palliative, aiming to improve the quality of life. When considering the management of individual tumour types, the maxim that prevention is better than cure should be recalled. Cancer prevention and screening are essential if the global burden of malignancy is to be minimized. During the last quarter of the 20th century, the role of chemotherapy, radiotherapy and endocrine therapy after primary surgery for localized breast cancer was recognized. These additional treatments are defined as adjuvant therapies. Thus, adjuvant therapy is treatment after the primary tumour has been removed surgically and in the absence of detectable residual disease. Whilst large clinical trials demonstrated the advantages of adjuvant therapy to a population of women with breast cancer, the benefits for each individual cannot be specified; the statistics relate to the group as a whole. Oncologists observing the success of adjuvant treatments have progressed to the investigation of the role of primary or neoadjuvant treatments. Neoadjuvant therapy, usually chemotherapy or endocrine therapy, is delivered prior to surgery or radiotherapy to downsize the tumour, thus demonstrating the sensitivity of the tumour and potentially reducing the extent of the surgical resection or radiation field. In order to work out what treatments are feasible for a person with cancer, the extent of spread of the cancer needs to be established as this is one of the most important influences on survival. In addition to the histological grade of a tumour, an important criterion in treatment decisions and the major determinant of outcome is the extent of spread or stage of a cancer (Figure 3.1). Staging a tumour is essentially an anatomical exercise that uses a combination of clinical examination and radiology. A uniform staging system is employed for most tumour sites that is based upon the size of the primary Tumour, the presence of regional lymph Nodes and of distant Metastases. The details of this TNM classification vary between different tumour sites. As always, there are exceptions, including the staging system for lymphomas that was originally set out following a conference at the University of Michigan in Ann Arbour. It is known as the Ann Arbour Staging System, and most radiologists assume that it is named after a person rather than a town, so this is a chance to score points at X-ray meetings. Figure 3.1 T-stage classification for bladder cancer and influence on survival. CIS, carcinoma in situ; Ta, non-invasive papillary tumour; T1, tumour invades subepithelial connective tissue; T2, tumour invades superficial muscle; T3, tumour invades deep muscle or perivesical fat; T4, tumour invades prostate, uterus, vagina, pelvic wall or abdominal wall. Staging depends to a large extent upon radiology, and this is the most commonly used tool to evaluate the response of cancers to therapies. Anatomical imaging by plain films, computed tomography (CT), ultrasound and magnetic resonance imaging (MRI) are the standard methods. Using the correct terms impresses other clinicians and may make a trip to the radiology department less daunting for junior doctors requesting an investigation. X-rays measure radiodensity (radiolucency and radio-opacity) and ultrasound measures echogenicity and echoreflectivity, whilst CT scans report attenuation values measured in Hounsfield units and MRI reports signal intensity. CT scanning is the production of three-dimensional images using X-rays that have been directed through tissues and the images produced depend on the density of the tissues. CT was developed in the 1970s by Sir Godfrey Hounsfield and Allan McLeod Cormack who shared the Nobel Prize in 1979. The first CT scanner built at EMI Central Research Laboratories is said to have been funded by the success of the Beatles who were signed to the EMI label. CT measures the attenuation of different tissues to ionizing radiation and calculates a mean value for a volume of tissue known as a voxel. This is displayed on a two-dimensional image as a single pixel. The attenuation is calculated relative to water, which has a Hounsfield unit (HU) value of 0, so high attenuation tissues have a positive HU value (e.g. bone +400 HU) and low attenuation tissues a negative HU value (e.g. fat −120 HU). Different window settings are used to look at different ranges of the Hounsfield greyscale. For example, in the bone windows setting, the lungs will look uniformly black, whilst in the lung windows, the bones look uniformly white. Intravenous iodinated contrast agents improve the sensitivity and specificity of CT but are contraindicated in patients with asthma, allergies to contrast or poor renal function. Figure 3.2 T1 (left) and T2 (right) MRI scans demonstrating a large right basal ganglia glioma with midline shift, compression of the right lateral ventricle and peritumoural oedema. Unlike CT, MRI does not use ionizing radiation but instead a powerful magnetic field aligns the spin of protons, especially hydrogen atom protons, in water and fat. A radiofrequency pulse then energizes the protons, and the gradual release of this energy from the protons as they relax back to their original magnetic alignment may be detected as radiofrequency signals. The signal intensity relates to the concentration of mobile hydrogen nuclei in tissues. T1 (longitudinal relaxation or spin–lattice) and T2 (transverse relaxation or spin–spin) relaxation time constants depend on the physical properties of the tissues. If you want to impress the neuroradiologists (not always a useful ploy in the authors’ experience), water such as cerebrospinal fluid is black (low signal intensity) on T1 images and white (high signal intensity) on T2 images (Figure 3.2). Whilst CT is a good tool to examine tissues composed of high atomic weight elements such as bone, MRI is better suited to non-calcified tissues. For similar reasons, CT contrast agents usually are composed of high atomic number atoms such as iodine or barium, whilst MRI contrast agents such as gadolinium are paramagnets that have magnetic properties only in the presence of an externally applied magnetic field. MRI is generally superior for imaging the brain, whilst CT is better for solid tumours of the chest and abdomen, as it is faster and generates fewer motion artefacts. MRI is also better suited to patients who may require many examinations because it does not carry the risks of ionizing radiation. MRI is, however, contraindicated in patients with metallic objects such as pacemakers in situ and it is also quite claustrophobic and noisy in the scanner. Positron emission tomography (PET) is a functional imaging modality that detects γ-rays emitted by positrons (positively charged electrons) emitting radionuclide tracers. Positrons have a short half-life and are generated by cyclotrons. Common positron-labelled radionuclides include fluorine (18F), carbon (11C), oxygen (15O) and nitrogen (13N). In oncology, the most frequently used tracer is 18F-fluorodeoxyglucose (FDG), a short half-life glucose analogue that is taken up into actively metabolizing cells including cancer cells and following intracellular phosphorylation is trapped in these cells. Hence, the distribution in the body of FDG reflects glucose uptake within the body. This means that PET scanning may differentiate between residual masses and active disease in lymphoma. As a consequence, FDG-PET scanning is used in both staging and monitoring cancer treatment (Figure 3.3). PET scanning techniques continue to progress through the use of novel tracers, such as choline in prostate cancer, which has greater specificity for the cancer than FDG. Figure 3.3 (a) T2-MRI and FDG-PET scan showing an FDG avid parietal primary cerebral lymphoma. (b) CT and FDG-PET scans demonstrating a huge right axillary and anterior chest wall mass due to Burkitt lymphoma. The FDG-PET also demonstrates extensive involvement of other nodal groups, bones and the right kidney upper pole (stage 4B). FDG, Fluorodeoxyglucose; PET, positron emission tomography. Table 3.1 Commonly used isotopes in nuclear imaging in oncology In addition to PET scanning, other functional images may be used in the diagnosis and staging of specific cancers, using isotope-labelled radionuclide tracer elements (Table 3.1 and Figure 3.4). The isotope-labelled tracers that are used diagnostically may also be used therapeutically. Bone scintigraphy uses bisphosphonates labelled with 99Tc and is more sensitive than X-rays for detecting metastases, though surprisingly the tracer reflects blood flow at the site of the supposed metastases and so there is a differential diagnosis for tracer uptake, which may include Paget’s disease and osteoarthritis. In addition to the histological grade and the stage of a cancer, the general health of patients will determine how long they survive and may influence treatment decisions. Scales that measure the performance status or functional capacity of patients include: the Eastern Co-operative Oncology Group (ECOG) grading system, which is also known as the WHO or Zubrod score and the Karnofsky scale (Table 3.2). The performance status, however estimated, is an important prognostic indicator for almost all tumour types. Figure 3.4 Plain pelvic radiograph (above) and corresponding area of technetium pyrophosphate bone scan (below) of a patient with sclerotic bone metastases from prostate cancer. Table 3.2 Functional capacity grading (ECOG) and Karnofsky performance scales Table 3.3 Survival rates for various diseases Although a very significant stigma is attached to the diagnosis of cancer, for most of the general population the fear outweighs the reality and comparison with other more palatable illnesses yield results that are not always expected (Table 3.3). Surgery has six major roles in the management of people with cancer: Surgical oncology is the oldest discipline for the management of cancer and originates with attempts at curative resections. Surgical oncology enjoyed a golden era at the end of the 19th century and early 20th century prior to the World War I (Table 3.4). Table 3.4 Landmarks in radical surgical oncology Subsequent advances in surgical oncology included the development of endocrine surgery for metastatic disease. Surgical hormone ablation for the management of breast cancer was pioneered over 100 years ago by George Beatson, a Glaswegian surgeon who gave his name to Scotland’s largest cancer centre (Table 3.5). The prevention of cancer by surgery has expanded greatly in recent years with the identification of individuals at high risk of developing malignancies because they have inherited germline genetic mutations associated with cancer predisposition. However, perhaps the most widespread example of surgical cancer prevention is the role of orchiopexy in the management of undescended testes. Undescended testes are the most common birth defect of the male genitalia, affecting up to 3% of live births. Although in many cases the testes will descend to the scrotum during the first year of life, undescended testes are associated with a 4–40-fold increased risk of malignancy, especially testicular seminoma. Orchiopexy, surgery to move the undescended testis into the scrotum, has been shown to reduce infertility, although it remains controversial whether it also reduces the risk of malignancy. Nevertheless, it certainly makes the detection of testicular tumours easier to recognize and diagnose at an earlier stage. Table 3.5 Landmarks in endocrine surgery for advanced cancer Familial adenomatous polyposis (FAP) is an autosomal dominant disorder characterized by the development of multiple polyps in the colon, which may undergo transformation to malignant tumours. Most patients with FAP will develop a colonic cancer by the age of 40 years, so prophylactic colectomy is generally recommended before the age of 25 years. Similarly, risk-reducing prophylactic mastectomy may be advocated for women with inherited mutations of BRCA genes (Table 3.6). Oncological diagnosis hinges on histopathology and surgeons play a major role along with interventional radiologists in obtaining tissue specimens. Whilst aspiration cytology and fine-needle biopsies can be undertaken radiologically or endoscopically, more extensive incision or excision biopsies require surgical involvement. Careful specimen orientation and inking prior to histological sectioning may be required to assess the status of tumour margins. The optimal surgical approach and biopsy technique for tumour sampling must take into account concerns about contaminating new tissue planes with cancer cells that could jeopardize subsequent therapy. For example, thoracoscopic pleural biopsy of mesothelioma may result in needle-track metastases along the path of surgical instrumentation. The risk of this surgical spread of the cancer is reduced in mesothelioma by postoperative radiotherapy to the biopsy track. Surgery had a major role in the staging of tumours prior to the development of improved radiological techniques and a staging laparotomy was routine care in the management of Hodgkin’s disease until the late 1980s. Surgical staging retains a role in the management of ovarian epithelial cancer and the surgical placement of radio-opaque titanium clips (that are non-ferrous and thus safe in the MRI scanner) may guide postoperative radiotherapy in some tumours. Table 3.6 Prophylactic surgery BRCA, breast cancer; MEN, multiple endocrine neoplasia. The surgical treatment of cancer includes definitive curative surgery (with or without adjuvant treatments), debulking operations, metastasectomy and endocrine ablation surgery for advanced diseases, although the latter is largely historical as pharmacological hormone manipulations have taken over most of this role. It is really important to avoid unnecessary surgery in patients with extensive unresectable cancer whilst ensuring that patients with potentially curative tumours are not denied surgery. The treatment of cancer by multidisciplinary teams including radiologists, pathologists, physicians and surgeons is designed to ensure the right patients have the right operations at the right time. For example, this approach aims to guarantee that patients who are candidates for neoadjuvant down-staging therapy prior to surgery are not wheeled straight into the operating theatre. Most early curative surgery aimed to remove tumours en bloc, that is, with the draining lymph nodes. In recent times, surgeons have developed the use of more conservative function-preserving operations. Examples of the latter include wide local excision rather than mastectomy and partial nephron-sparing nephrectomy rather than radical nephrectomy for small renal tumours. A further development in surgery for skin tumours was introduced by Dr Frederic Mohs in the 1930s when he was still a medical student at the University of Wisconsin-Madison. Mohs’ surgery for skin tumours involves sectioning the tumour and mapping its surgical margins during the operation to ensure completeness of tumour resection. Instead of using a breadknife and the pathologist looking at each slice, the Mohs’ technique is more like a vegetable peeler with the pathologist examining each peeling for residual cancer involvement during the surgery. Whilst this is a more laborious technique, it is especially valuable for tumours at specific anatomical sites such as the eyelids and in recurrent disease (see Fig. 32.6, p. 262). Debulking operations that do not result in complete surgical removal of the tumour are not always futile. They can provide important clinical benefits in selected tumour types, including ovarian cancer and primary brain tumours, and are usually followed by either chemotherapy or radiotherapy. Debulking surgery forms part of the treatment algorithm in several uncommon tumours such as thymomas and pseudomyxoma peritonei. Mucin secretion into the abdominal cavity by mucinous adenocarcinomas most commonly arising in the appendix is the usual cause of pseudomyxoma peritonei. The term “myxoma” is derived from the Greek for mucin, but the etymology of the medical terms myxoma and pseudomyxoma seem to have got mixed up. Myxomas are benign pedunculated connective tissue tumours usually arising in the atria of the heart and are not mucinous, whilst pseudomyxoma peritonei fills the abdominal cavity with true jelly-like mucin. The surgical resection of secondary deposits may seem perverse since the presence of metastases implies systemic dissemination of the cancer. Nevertheless, surgical oncologists have embraced this approach enthusiastically, mainly on the basis of relatively weak evidence from uncontrolled retrospective series that have been interpreted as demonstrating a survival benefit. The resection of lung, liver and brain metastases has become a part of the routine treatment strategy for a number of types of cancer. The most common indication for surgical metastasectomy is for hepatic secondaries from colorectal cancer. The rationale for this approach is based upon reported 5-year survivals of around 30% in patients undergoing surgery compared to around 10% in those who received chemotherapy. However, there are no randomized controlled studies that support hepatic metastasectomy and the case series are inevitably confounded by selection and reporting bias. Similarly, pulmonary metastasectomy has been widely adopted for osteogenic sarcomas, soft tissue sarcomas, renal cell tumours and melanomas, and cerebral metastasectomy has also been advocated in a similar spectrum of malignancies. In contrast to metastasectomy, the surgical resection of residual masses following the completion of chemotherapy forms part of the multidisciplinary treatment of advanced non-seminomatous germ cell tumours to remove residual differentiated teratoma that could relapse at a later date. Finally, surgical endocrine ablation is nowadays rarely indicated for metastatic cancer although surgical castration is occasionally performed for advanced prostate cancer. Surgery has an important role in the optimal management of oncological emergencies, in particular, metastatic spinal cord compression where rapid surgical decompression reduces neurological disability (see Cord compression in Chapter 46). Surgical decompression and spinal column stabilization should be offered to patients with a good prognosis and needs to be undertaken as swiftly as possible, preferably before patients lose the ability to walk. The optimal neurosurgical approach will depend upon the anatomical site of compression and the stability of the spine. If spinal metastases involve the vertebral body or threaten spinal stability, posterior decompression should always be accompanied by internal fixation with or without bone grafting. The palliation of tumour-associated obstruction, fluid accumulation and bleeding may require surgical intervention, although the placement of shunts and stents is now more frequently undertaken by endoscopists and interventional radiologists. Plastic or metal stents are used to relieve obstruction of the bowel, oesophagus, bronchial tree, biliary ducts and ureters and even patients with advanced malignancy benefit (Figure 3.5). Diathermy or laser coagulation of tumour-related haemorrhage similarly is a valuable palliative intervention that can often be undertaken endoscopically. Surgical relief of bowel obstruction may be indicated when stenting is not feasible but usually requires either colostomy or ileostomy depending upon the level of the obstruction. Fistulae are abnormal passageways connecting two epithelial-lined organs not normally connected and include rectovaginal, enterovaginal, colovesical and vesicovaginal or combinations of these. Fistulae may be either related to locally advanced disease or may be a consequence of radiotherapy. Fistula surgery is complex and demanding, requiring surgical expertise and often prolonged recovery, so it is usually reserved for cancer patients in remission. The surgical placement of shunts to prevent the reaccumulation of ascites (usually peritoneal–venous shunts, e.g. LeVeen shunt) and pleural effusions (usually pleuroperitoneal shunts, e.g. Denver shunt) may be indicated for symptom palliation. Surgical oncologists may also be called upon to perform surgery for ulcerating and necrotic locally advanced cancers such as toilet mastectomy for fungating breast tumours. Orthopaedic surgeons frequently operate on pathological bone metastases either as a form of secondary prevention or following pathological fractures (Figure 3.6). Figure 3.5 Oesophageal stent to palliate dysphagia. Figure 3.6 Pathological spiral fracture through right femoral shaft metastasis from prostate cancer repaired with a distal femoral plate. Internal fixation of bones with lytic metastases are especially important in weight-bearing bones with large deposits occupying more than half the bone cortex that are at a high risk of fracturing. Surgeons thus have an important role in the palliation of symptoms in advanced malignancy, and their input into the multidisciplinary team should not be seen as just to establish the diagnosis and surgically resect curable cancers. The aggressive treatment of bulky tumours often leaves major residual defects and in combination with plastic surgeons, the discipline of surgical oncology has developed reconstructive surgery to reduce some of the effects of tumour resections. Plastic surgeons have developed a reconstructive ladder of increasingly complex wound management to deal with some of these residual deficits (Table 3.7). Reconstructive surgery is not, however, the exclusive responsibility of plastic surgeons. For example, orthopaedic surgeons have developed sophisticated procedures for limb reconstruction following sarcoma surgery using bone grafts and prostheses. Maxillofacial surgeons reconstruct mandibles with free-flap fibula transplants following surgery for oral cavity cancers. ENT surgeons medialize vocal cords with silicon injection to overcome the hoarse voice associated with recurrent laryngeal nerve palsy caused by mediastinal tumours. Breast reconstruction following mastectomy often involves the insertion of a tissue expander that is progressively injected with saline over the ensuing weeks until a suitable size and shape has been achieved, when it may be replaced by a more permanent implant. The second most common breast reconstruction procedure involves flaps of tissues from other parts of the body such as the back, abdomen, buttocks or thigh. These flaps may be pedicled, leaving the original blood supply or may be free flaps with vascular microsurgery to connect to a new blood supply. A latissimus dorsi muscle flap can be performed without significant loss of function and retaining its original blood supply. Abdominal flaps usually take tissue from the lower abdomen, for example, the transverse rectus abdominis myocutaneous (TRAM) flap that leaves the abdominal wall weakened. More recent abdominal flaps attempt to retain abdominal wall strength by using a muscle-sparing deep inferior epigastric perforator (DIEP) flap or superficial inferior epigastric artery (SIEA) flap. These require greater microsurgical skill. Other autologous tissue donor sites for breast reconstruction include superior gluteal artery perforator (SGAP) and inferior gluteal artery perforator (IGAP) flaps from the buttocks. Table 3.7 Reconstructive ladder of increasingly complex wound management coined by plastic surgeons Radiotherapy involves the use of high-energy ionizing radiation to cause DNA damage and ultimately cell death. The damage induced by ionizing radiation may be lethal or sub-lethal to the tumour cells. In sub-lethal cell injury, damage to cellular proteins and organelles causes microscopic changes in the cell characterized down the microscope by swelling of mitochondria and endoplasmic reticulum and cloudiness of the cytoplasm known as hydropic degeneration. Cells may repair sub-lethal damage by removing damaged proteins and organelles by a cell stress response and autophagy and replace them with newly synthesized components. In contrast, lethal damage results in either cell necrosis or apoptosis. Ionizing radiation acts by ejecting an electron from an atom to yield an ion pair. This may lead to direct damage to DNA via molecular excitation or indirectly via the hydrolysis of water into free radicals with an open electron shell configuration characterized by the presence of an unpaired electron (Figure 3.7). These free radicals are highly reactive, usually short-lived chemicals such as the neutrally charged hydroxyl radical (OH·) derived from water, which has an in vivo half-life of about 10−9 seconds. The other common free radical formed from water is superoxide (O−2) with one unpaired electron, which is responsible for the “oxidative burst” or oxygen-dependent intracellular killing of ingested bacteria by phagocytes and is detoxified by the enzyme superoxide dismutase. Figure 3.7 How radiation damages DNA. Radiation is like rain: The dose of radiotherapy is defined as the amount of energy deposited in tissues and is measured in Grays (Gy) after Hal Gray, a British pioneer of radiation biology and physics who also established the Gray Laboratories at Mount Vernon Hospital. One Gray is the dose absorbed when 1 J (joule) is deposited in 1 kg of tissue. Each Gray per cell causes approximately 10,000 damaged DNA bases, 1000 damaged deoxyribose sugars, 1000 single strand breaks, 40 double strand breaks, 150 DNA–protein cross-links and 30 DNA–DNA cross-links. Radiation can have an effect at any point in the cell cycle, although it is only at the time of mitosis that cell death occurs. Therefore, there can be a time lag of days, weeks or even months between the radiotherapy and the full effects of the treatment becoming manifest. Typical radiotherapy doses for solid epithelial tumours are 60–80 Gy. This is delivered as multiple fractions over time for several reasons. Dose fractionation allows normal cells to recover from sub-lethal damage between fractions, whilst tumour cells are less efficient at repairing damage. Dose fractionation also ensures that tumour cells in different phases of the cell cycle are exposed to radiation since it causes greatest damage in the G2 and M phases. The exact scheduling and fractionation of radiotherapy varies but in general doses of around 2 Gy are delivered on a daily basis 5 days a week. In some circumstances, more frequent dosing has been shown to be more efficacious but is of course more demanding on resources. For example, continuous hyperfractionated accelerated radiotherapy (CHART) involves radiotherapy delivered three times a day, every day of the week, usually for a fortnight. Radiotherapy utilizes X-rays, electron beams and β- or γ-radiation produced by radioactive isotopes. X-rays are produced when a high-energy electron beam that is produced by heating an electrode in a vacuum, strikes matter. The energy of X-rays can be changed by altering the voltage input to the cathode of the X-ray tube that accelerates the electrons. Diagnostic radiology uses low-voltage equipment (e.g. 50 kV), producing X-rays of longer wavelength that are less penetrating. Therapeutic X-rays are produced by higher-voltage machines (30–50 MeV) producing shorter wavelength, more penetrating X-rays. Radiotherapy is delivered in three ways: external beam radiotherapy, brachytherapy and radioisotope therapy. External beam radiotherapy involves the use of isotope sources or linear accelerators to deliver radiation from a distance. In the case of brachytherapy, the radioactive source is a solid radioactive nuclide emitting γ-rays, which is placed within the tumour or closely applied to the tumour. Finally, radioactive isotopes that are preferentially taken up in the target organ may be administered orally or intravenously; for example, oral iodine-131 is given for the treatment of thyroid tumours and intravenous strontium-89 in palliative treatment of bone metastasis. Superficial voltage machines operate at 50–150 kV, and their energy does not penetrate more than 1 cm below the surface of the skin. They are used chiefly to treat superficial skin cancers. Orthovoltage machines that yield X-rays of 200–300 kV of energy penetrate to a depth of 3 cm. Metastases in bones close to the skin surface (ribs, sacrum) are frequently treated on these machines. Megavoltage radiotherapy machines usually use a cobalt-60 source that produces X-rays of 1.25 MeV on decaying to nickel-60. The cobalt-60 sources are contained within a protective lead shield and an adjustable window in this shield allows regulation of the γ-ray beam. However, there is considerable scatter of the beam, limiting the focus and the relatively short half-life of the cobalt source means that it needs to be replaced every 3–4 years and that treatment times may become prolonged as the cobalt nears the replacement date. It is speculated that cobalt-60 sources could be used by terrorists to produce a “dirty” bomb, a conventional explosive device to which radioactive material has been added. Megavoltage machines have been replaced by linear accelerators that produce a high-energy electron beam by accelerating electrons down a cylindrical waveguide before they bombard a fixed target, resulting in a high-intensity electron beam (4–20 MeV) with greater penetration and less scatter. The advantages of this electron beam over X-rays lie in the penetration and decay characteristics that allow an electron beam to deliver its high energy to deep-seated tumours whilst sparing normal tissues in its pathway (Figure 3.9). The accurate shaping of the radiation field to encompass the cancer but minimize damage to normal tissues really began with the introduction of the multileaf collimator composed of over 100 metal leaves, 5 mm thick and each aligned parallel to the radiation field. As each leaf may be moved independently to block part of the field, the resulting radiation field may be shaped and sculpted to suit the target. A further refinement of this process, known as intensity-modulated radiotherapy (IMRT), involves moving the multileaf collimator during the dose so that another level of fine tuning of the field can be achieved (Figure 3.10). One more recent development is image-guided radiation therapy (IGRT) that links the carefully shaped field with a continuous image of the patient. This process can overcome, for example, movement artefacts generated by the patient (hopefully still) breathing. Brachytherapy employs sealed radionuclide sources that are implanted directly into a tumour or body cavity to deliver localized radiotherapy (Table 3.8). Examples of brachytherapy include radioactive iridium-192 needles or wires implanted into tumours of the breast, tongue and floor of the mouth. Sealed caesium-137 radioactive sources may also be placed into the vagina or rectum to treat cancers of the vagina, cervix, lower uterus, rectum or anus. Brachytherapy seeds are increasingly being used to treat localized prostate cancer (Figure 3.11). The major disadvantage of brachytherapy is the risk to staff handling the radioactive sources and caring for the patients. The radioactivity exposure of all staff involved with brachytherapy must be monitored. Another method used to reduce exposure is to place inactive source holders while the patient is anaesthetized and once they have been correctly located (as determined by X-ray), the patient is allowed to recover from the procedure. With the patient in a shielded room, the live radioactive source is then introduced, either manually or by remote control using a selectron. This routine is termed manual or remote after-loading and is frequently used for tumours of the upper vagina, cervix and endometrium. Radioactive isotopes can be given by mouth or injection and are taken up by a particular tissue where they remain. Radioisotope therapy can only be used where a tumour is in a tissue that will preferentially accumulate a specific isotope, leaving other tissues unaffected (Figure 3.12). Examples are the thyroid, which will take up radioactive iodine and bone that naturally accumulates phosphorus or will take up bone-seeking radiochemicals such as rhenium-186 hydroxyethylidene diphosphate (186Re-HEDP) (Table 3.9). A disadvantage with this approach is that the source cannot be recovered, limiting the degree of control over the total exposure to radiation. Figure 3.9 (a) Squamous cell cancer of the oral cavity. (b) Radiological verification of the radiotherapy fields. (c) The planned radiotherapy fields. Figure 3.10 Comparison of conventional and intensity-modulated radiotherapy planning. Table 3.8 Radionuclides used for brachytherapy Figure 3.11 Prostate brachytherapy seeds in situ seen on pelvic X-ray. Figure 3.12 Selective internal radiation therapy (SIRT) using microspheres loaded with radioisotopes (in this case Yttrium-90) injected into the hepatic arteries to treat inoperable liver cancer. The microspheres are about one-third the size of a human hair width. Table 3.9 Table of systemically administered radionuclides used in oncology Gamma: 364 keV Half-life: 8 days β- and γ-emitter Half-life: 14 days β-emitter Half-life: 17 hours β-emitter Gamma: 103 keV Half-life: 47 hours β- and γ-emitter Half-life: 50 days β-emitter External beam radiotherapy is usually given as repeated daily dose fractions rather than as a single large dose of radiotherapy, which would lead to severe damage to the normal tissues. Even with fractionation, normal tissues have a maximum tolerated dose as indicated in Table 3.10. The area to be irradiated is referred to as the radiation field. This is marked out on the skin before treatment, and such markings often persist after treatment as tattooed dots. These radiotherapy tattoos are assiduously sought by clinical medical exam candidates, but in real life, most patients will tell you that they have had radiotherapy if you ask them nicely. In general, radiation-related side effects occur within the field of treatment although a few systemic manifestations such as fatigue and nausea may occur. The toxicity of radiotherapy increases with both the volume of tissue irradiated and the dose given. The transient side effects that develop during treatment tend to reflect the acute damage to normal healthy tissue. Careful planning of the beam size and shielding of surrounding tissue, ensuring that radiation fields give effective tumour eradication with an acceptable level of toxicity, is, therefore, a prerequisite of successful therapy. Table 3.10 Table of normal tissue tolerance of radiotherapy Radiotherapy-related side effects can be usefully divided into early and late toxicities (Table 3.11). Early toxicity occurs in hours to weeks and includes both systemic effects such as nausea, lethargy and myelosuppression (when a large volume of bone marrow is within the treated area; e.g. whole femur or pelvis radiation). Localized skin toxicity is a common early side effect that may be local erythema progressing to ulceration and desquamation in the more severe cases. Other early side effects depend on the anatomy of the radiotherapy field, for example, alopecia with cranial irradiation (Figure 3.13), oropharyngeal mucositis with head and neck radiotherapy and diarrhoea, proctitis and cystitis with pelvic fields. These early reactions occur in tissues that are rapidly dividing and are usually present during or shortly after the course of radiotherapy and in most cases are reversible. Late side effects may take months or years to manifest themselves and once again depend upon the site being irradiated. These late tissue reactions occur when slowly dividing cells attempt division and are less frequently reversible. In some cases, the effects are believed to be mediated by fibrosis of the vascular endothelium. Examples of late reactions include necrosis in the central nervous system leading to transverse myelitis and paralysis with spinal cord radiation fields, radiation-induced pneumonitis (Figure 3.14), nephritis and osteomyelitis. Finally, radiotherapy is carcinogenic and may induce secondary tumours. Table 3.11 Table of adverse early and late reactions to radiotherapy Figure 3.13 Clearly demarcated scalp alopecia due to radiotherapy. Figure 3.14 Radiation pneumonitis with alveolar infiltrates and basal consolidation following irradiation of right breast cancer. Tumour resistance to radiotherapy appears to be an intrinsic property of that cancer, rather than an acquired attribute selected for by treatment, as in the case of chemotherapy. Indeed, the radiation sensitivities of many types of tumours are relatively predictable. The response of tissues both malignant and normal to fractionated radiation depends upon the “5 Rs”: In this context, repair is recovery from sub-lethal damage and is dependent on DNA repair mechanisms. Reassortment refers to the cell cycle phase of the tumour cells. Cells in G2 and M phases are most susceptible to radiotherapy, so after a first dose, cells in G1 and S will make up a greater proportion of the live tumour cells. Depending on the timing of the subsequent fraction of radiotherapy, these cells may have progressed or “reassorted” to G2 and M phases with increased sensitivity. Repopulation is the ability of tumour cells to grow and divide between doses of radiotherapy; this is a particular problem with prolonged fractionation and delayed fractions. Hypoxic cells are relatively radioresistant, and after the first fractions of radiotherapy, the death of sensitive cells reduces the competition for oxygen in the tumour, and cells that were hypoxic previously become reoxygenated and hence more susceptible to radiation. Different cell lineages are more or less radiosensitive, and these differences are in part intrinsic and independent of environmental factors. Amongst the factors that influence the radiosensitivity of tumours are the DNA repair genes, the production of free radical scavenging molecules (e.g. glutathione-S-transferases, superoxide dismutases, glutathione peroxidase), genes controlling apoptosis and cell cycle regulatory genes. The origins of chemotherapy for cancer lie in the use of biological warfare during the First World War, most hauntingly described in Wilfred Owen’s poem “Dulce et decorum est”. Following the extensive use of chlorine gas in trench warfare, the German Army first released mustard gas at Ypres on the night of 12–13 July 1917. Mustard gas had been synthesized in 1854 by Victor Meyer and was noted to be a vesicant in 1887. As a weapon of mass destruction, mustard gas, or Yperite as it was then known, had the advantages over chlorine of requiring smaller doses, being almost odourless and remaining active in the soil for weeks. The British gas casualties from 1914–1918 reveal the greater fatalities with mustard gas. Mustard gas exposure causes a severe blistering rash and conjunctivitis followed by myelosuppression after around 4 days. During the Second World War, the only use of mustard gas resulted in an own goal when the Luftwaffe sunk the USS John Harvey off Bari harbour in southern Italy in 1943. The ship was carrying 2000 M47A1 bombs containing a total of 100 tonnes of mustard gas, and the American sailors who survived developed conjunctivitis and skin blistering followed by a steep fall in their white cell counts, as documented by the naval surgeon Colonel Stewart Alexander. Meanwhile at Yale University, Alfred Gilman and Louis Goodman were using the closely related nitrogen mustard (mechlorethamine) initially in murine lymphoma models. In 1944, the first patient with lymphosarcoma (high-grade non-Hodgkin’s lymphoma) was treated, and although Mr J. D., a 48-year-old silversmith, achieved a temporary remission of his tumour, he later died of bone marrow failure. The subsequent development of chemotherapy following this fortuitous finding as a by-product of biological warfare, owes much to luck and trial and error rather than design. One serendipitous discovery was made by Barnett Rosenberg, a physicist at Michigan State University in 1965. He studied the effects of electric currents on Escherichia coli by using platinum electrodes in a water bath and found that they stopped dividing but not growing, leading to bacteria up to 300 times longer than normal. This was found to be due to cisplatin, a product from the platinum electrodes, which was interfering with DNA replication. By the end of the 1960s, a number of cytotoxic drugs from natural sources had been identified. In 1971, President Nixon, losing the war in Vietnam, declared war on cancer, signing the Cancer Act and establishing a drug discovery programme at the National Cancer Institute (NCI). This project trawled though thousands of natural chemicals in search of potential cytotoxic agents. It was not until the 1990s that rational drug design targeting known tumour-related features emerged. Examples of this include trastuzumab, a monoclonal antibody raised against erbB2/neu/Her-2 in breast cancer and imatinib, which inhibits the adenosine triphosphate (ATP) binding site of brc-abl fusion protein kinase in chronic myeloid leukaemia. In 2013, over 125,000 cancer patients in England were treated with systemic anticancer therapy receiving over 538,000 cycles of treatment. Amongst the many classifications of cytotoxic agents is a functional classification of cytotoxics (Table 3.12). Alkylating agents transfer an alkyl group to purine (adenine and guanine) bases of DNA. Bifunctional alkylating agents form covalent bonds between two different bases, resulting in interstand or intrastrand cross-links, whilst monofunctional alkylating agents cannot form cross-links but cause adducts. Both forms of DNA alteration inhibit DNA synthesis, so alkylating agents act chiefly during the S phase of the cell cycle. Bifunctional agents can act on more than one base and are more cytotoxic, whilst monofunctional agents are more mutagenic and carcinogenic. One of the mechanisms of tumour resistance to alkylating agents is enzymatic removal of alkyl groups from purine bases and enhanced repair of cross-links. Antimetabolites are structurally similar to natural compounds and in general interfere with cellular enzymes. These agents inhibit the metabolism (usually synthesis) of compounds necessary for DNA, RNA or protein synthesis. They include (1) purine analogues, (2) pyrimidine analogues, (3) folic acid analogues and (4) others, for example, hydroxyurea. Most antimetabolites have their greatest activity during the S phase. Intercalating agents disrupt the steric integrity of the DNA double helix. The exact mechanisms of this action remain uncertain, although anthracycline antibiotics intercalate into the DNA major groove between base pairs of the DNA double helix and this action is non-covalent with no base sequence specificity. Platinum agents also intercalate and form intrastrand links similar to those formed by alkylating agents. Antimicrotubule drugs can be divided into two groups, those that stabilize microtubules by inhibiting depolymerization (e.g. taxanes) and those that are depolymerizing agents that inhibit polymerization of tubulin (e.g. vinca alkaloids). Spindle poisons inhibit the mitotic spindle function and therefore act in the M phase of the cell cycle. Tubulin exists as α-tubulin and β-tubulin monomers in dynamic equilibrium with tubulin polymers or microtubules. Resistance to spindle poisons may occur by mutations of β-tubulin, and these point mutations do not confer cross-resistance between taxanes and vincas. An early spindle cell poison included colchicine used for acute gout, familial Mediterranean fever and rarely psoriasis. Although colchicine, like vincas causes depolymerization, it binds to a distinct site and is not used as a cytotoxic. Table 3.12 A functional classification of cytotoxics Cyclophosphamide Melphalan CCNU (lomustine) Streptozotocin Temozolomide Thiotepa 6-Thioguanine Gemcitabine Ralitexed Carboplatin Oxaliplatin Anthracyclins Daunorubicin Mitomycin C Actinomycin D Vinblastine Vinorelbine Docetaxel Irinotecan Teniposide Topoisomerases prevent DNA strands from becoming tangled by cutting DNA and allowing it to wind or unwind. There are two mammalian classes of topoisomerases: topoisomerase I breaks single strands of DNA, whilst topoisomerase II breaks both strands of DNA. Topoisomerase I inhibitors act by inhibiting the religation step of the nicking–closing reaction, trapping topoisomerase I in a covalent complex with DNA. Topoisomerase I inhibitors act in the S phase and belong to the camptothecin group. Camptothecin was discovered by the NCI screening of plant-derived compounds and was isolated from a Chinese small tree Camptotheca acuminata. Topoisomerase II is inhibited both by DNA intercalators (e.g. anthracyclines) and by non-intercalators (e.g. epipodophyllotoxins). The major obstacle to successful cures with chemotherapy is the development of drug resistance by tumours. Indeed, the intrinsic resistance of some tumour cell types accounts, in part, for the variable sensitivity of different cancers to chemotherapy (Table 3.13). In some circumstances drug resistance is to a single drug only, whilst in other cases there is cross-resistance between different drugs. The latter mechanism is due to the expression of molecular efflux pumps in tumour cell membranes. The most commonly found pump in multiresistant tumour cells is P-glycoprotein (Pgp) or the multidrug resistance protein (MDR). This transmembrane protein pumps natural toxins out of cells (including most chemotherapy agents) and is normally present in selected cells of the body such as renal proximal tubule cells, the apical mucosal cells of the colon and the canilicular surface of hepatocytes. Overexpression of Pgp/MDR by cancer cells confers a survival advantage in the presence of chemotherapy by inducing tumour resistance. Table 3.13 Sensitivity and curability of selected cancers treated with chemotherapy Lymphomas Germ cell tumours Childhood tumours Myeloma Colorectal cancer Ovarian cancer Bladder cancer Melanoma Adult brain tumours Prostate cancer Cytotoxic-specific drug resistance can be achieved by a number of mechanisms, including efficient repair of DNA, reduced drug uptake, increased drug efflux, decreased intracellular activation of the drug, increased intracellular inactivation of the drug, activation of biochemical pathways that bypass the pathway being blocked by the cytotoxic drug and finally compensation for blocked enzyme pathways by increased enzyme production. An example of the last form of drug-specific resistance occurs with methotrexate, an antifolate antimetabolite that inhibits dihydrofolate reductase (DHFR). The first ever cancer cures with chemotherapy alone were reported with methotrexate for choriocarcinoma in 1963. In resistant tumour cells, there is amplification of the DHFR gene, with many thousands of copies of the gene leading to higher levels of DHFR to overcome the inhibitory actions of methotrexate. Cytotoxic drugs are rarely used as single agents but are usually administered in combinations in an attempt to improve treatment efficacy by reducing the development of drug resistance, based on similar principles in the management of infectious diseases such as tuberculosis. A number of considerations are applied to the design of chemotherapy combinations. Only drugs that have proven activity as single agents should be included and preference should be given to drugs with non-overlapping toxicities and different modes of action. Cycles or pulses of chemotherapy given intermittently are designed to allow for recovery of normal tissues between doses without enabling the tumour cells to repopulate. Although this goal is frequently desirable, in recent years a number of continuous infusion chemotherapy regimens have been developed. The importance of a good acronym for a chemotherapy regimen should not be underestimated. No single regimen has remained the gold standard of care for as long as the CHOP regimen for non-Hodgkin’s lymphoma, easily seeing off competition from the likes of ProMACE-CytaBOM. With greater experience of the benefits and disadvantages of chemotherapy, its safety has improved and the indications for its use have expanded. As with radiotherapy and endocrine treatments, chemotherapy is increasingly used in an adjuvant context (Table 3.14). In some circumstances chemotherapy, resistance may be overcome by escalating the dose of cytotoxic drugs. In many circumstances, the dose-limiting toxicity (DLT) of chemotherapy is myelosuppression, and if this can be avoided, doses may be doubled or more before reaching the next DLT, which is often mucosal damage. Autologous (from the patient him/herself) and allogeneic (from a donor) bone marrow transplantation (BMT) was developed to this end. Prior to high-dose chemotherapy, progenitor stem cells are harvested either from multiple bone marrow aspirations (BMT) or now more often from peripheral blood following growth factor stimulation (peripheral blood stem cell transplant (PBSCT)). These stem cells are immature haematopoietic cells capable of repopulating the bone marrow. The patient then receives the conditioning high-dose chemotherapy and/or radiotherapy, and subsequently the stem cells are reinfused as a transfusion. This approach has an appreciable mortality of 20–50% in the case of allogeneic BMT and of 5% with autologous PBSCT. However, stem cell transplantation has a defined role in the management of a number of malignancies (Table 3.15). Table 3.14 Cancers effectively treated by neoadjuvant and adjuvant chemotherapy Osteosarcoma Locally advanced breast cancer Osteosarcoma Breast cancer Colorectal cancer Table 3.15 Cancers effectively treated by high-dose chemotherapy and stem cell transplantation ALL, acute lymphoblastic leukaemia; AML, acute myeloid leukaemia; CML, chronic myeloid leukaemia. The main actions of chemotherapy are focused on killing rapidly dividing cancer cells and hence many of their toxicities arise because of the effects on normal cells with high rates of turnover. Indeed, the side effects of chemotherapy may be divided into the predictable toxicities that are common, often dose related and usually related to the mechanism of action of the drug. In contrast, idiosyncratic side effects are usually rarer, unrelated to dose or mechanism of action but tend to be drug specific. The predictable effects of chemotherapy on fast dividing normal cells (bone marrow, gastrointestinal tract epithelium, hair follicles, spermatogonia) will be a consequence of inhibition of cell division and are especially found with cell cycle phase-specific cytotoxics. In contrast, the side effects on slow-growing cell types will occur most frequently with drugs that are not cell cycle specific, such as the alkylating agents that introduce DNA mutations into these cells, resulting in secondary leukaemias and other tumours. The side effects of chemotherapy may be divided into three time groups: (1) immediate effects that occur within hours, (2) delayed effects that occur within days, weeks or months but are generally manifested whilst the full course of chemotherapy treatment is ongoing and (3) late effects that occur months, years or decades after the chemotherapy has ceased. The top five side effects ranked by patients according to severity are nausea, fatigue, hair loss, concern about the effects on friends and family and finally vomiting. The immediate toxicities include nausea and vomiting, anaphylaxis, extravasation and tumour lysis. The delayed side effects are the most abundant and include alopecia, myelosuppression, stomatitis and the majority of the unpredictable toxicities. The late effects of chemotherapy include infertility and secondary malignancies. Vomiting is a central reflex initiated in the vomiting centre of the medulla that coordinates the contraction of the diaphragm and abdominal muscles with relaxation of the cardiac sphincter and the muscles of the throat. There are four inputs into the vomiting centre: the labyrinths (e.g. motion sickness), the higher cortical centres (e.g. fear, anticipation), the vagus nerve sensory input from the gastrointestinal tract, particularly the small bowel, and finally the chemoreceptor trigger zone (CTZ). The CTZ is located in the area postrema adjacent to the fourth ventricle where the blood–brain barrier is relatively deficient and chemicals in the blood and cerebrospinal fluid are sensed, stimulating the vomiting centre. The different inputs to the vomiting centre rely on different neurotransmitters, and this can be exploited pharmacologically in the control of symptoms (Figure 3.15). Chemotherapy chiefly acts on the gastrointestinal tract, causing serotonin (5-hydroxytryptamine (5HT)) release and acting via the afferent vagus nerve. It also stimulates the CTZ, which employs dopaminergic and muscarinic pathways. Occasionally, anticipatory vomiting is problematic, and this acts through the higher cortical centres using γ-aminobutyric acid (GABA) neurotransmission. In contrast, the labyrinthine pathways utilize histamine-1 receptors, and motion sickness is often successfully controlled with antihistamines. Figure 3.15 Neural pathways in vomiting. The likelihood of being sick with chemotherapy depends upon the emetogenicity of the cytotoxics used as well as host-related factors. Cisplatin and mustine are amongst the most emetogenic, whilst vinca alkaloids rarely cause nausea. Younger age, women, patients who have been sick previously with chemotherapy and patients with a low alcohol intake are all more likely to suffer with chemotherapy-induced vomiting. Acute vomiting within 6 hours of chemotherapy is best controlled by a combination of steroids and 5HT-3 receptor antagonists. Delayed vomiting occurring up to 5 days after the chemotherapy is best treated with steroids and dopamine antagonists. Anticipatory vomiting that occurs prior to receiving chemotherapy is treated with benzodiazepines. As with all medicines, anaphylaxis may occur with chemotherapy and the most common culprits are taxanes and asparaginase. The incidence of hypersensitivity with paclitaxel is so high that routine prophylaxis with steroids and antihistamines (H1 and H2) is administered to all patients receiving paclitaxel. Extravasation is the inadvertent administration of chemotherapy into the subcutaneous tissue. This leads to pain, erythema, inflammation and discomfort, which if unrecognized and untreated can lead to tissue necrosis with the possibility of serious sequelae. The position, size and age of the cannulation site are the factors that have the greatest bearing on the likelihood of problems occurring (Figures 3.16, 3.17, 3.18 and 3.19) and the experience of the specialist administering the chemotherapy is crucial in this aspect. The likelihood of damage occurring is determined by the cytotoxic drug, with anthracyclines being especially likely to cause severe injury. Figure 3.16 Extravasation of carboplatin chemotherapy showing swollen and red skin which was cold. The rapid cytolysis of a large volume of cancer cells at the start of chemotherapy occasionally results in the tumour lysis syndrome or metabolic chaos. The destruction of tumour DNA leads to hyperuricaemia from the breakdown of nucleotide bases. The cytolysis causes hyperkalaemia by releasing intracellular potassium, and the breakdown of proteins and DNA causes hyperphosphataemia and secondary hypocalcaemia. Acute renal failure may be a consequence of the high levels of urate and phosphate, whilst the high levels of potassium may lead to cardiac arrhythmias. Tumour lysis only really occurs to a significant extent with acute leukaemias and high-grade lymphomas including Burkitt’s lymphoma. Bulky tumours, poor renal function and high levels of urate before chemotherapy increase the risk of tumour lysis. Uric acid is soluble at physiological pH but precipitates in the acidic environment of the renal tubules, leading to crystallization in the collecting ducts and ureters, leading to obstructive uropathy. Similarly, calcium phosphate is precipitated in the renal tubules and microvasculature producing nephrocalcinosis. The most important issue in the management of tumour lysis is its prevention by a combination of hyperhydration, allopurinol and urinary alkalinization to pH >7 with sodium bicarbonate to reduce urate precipitation in the renal tubules. Allopurinol is an inhibitor of xanthine oxidase, the enzyme that catalyzes the conversion of soluble xanthine (a product of purine catabolism) to uric acid. The treatment of established tumour lysis is an oncological emergency. The majority of patients who develop tumour lysis have chemosensitive tumours and are receiving a potentially curative treatment. These patients should be considered candidates for urgent haemodialysis. A relatively new addition to the treatment is recombinant urate oxidase (rasburicase), which converts insoluble urate to soluble allantoin (see Chapter 40). Figure 3.17 Peripherally inserted central catheter (PICC). Figure 3.18 A patient with a Hickman line, a skin-tunnelled, long-term silicon catheter with a Dacron cuff about 2 cm above the exit site that acts as a barrier to micro-organisms and prevents catheter dislodgment. Hickman lines are used for continuous infusional chemotherapy or for patients with poor venous access. Hickman lines are placed in the radiology department using ultrasound guidance or in theatres under general anaesthetic. Figure 3.19 Portacath for the delivery of chemotherapy. The main predictable delayed side effects of chemotherapy are alopecia, bone marrow suppres- sion and gastrointestinal mucositis. Hair loss with chemotherapy is both drug and dose dependent and is related to the frequency of cycle repetition. Long-term therapy may result in the loss of pubic, axillary and facial hair in addition to scalp hair. The loss of scalp hair often occurs in an acute episode while washing, usually 2–6 weeks after starting chemotherapy. It should be emphasized to patients that alopecia from chemotherapy is reversible, with hair regrowth beginning 1–2 months after completing chemotherapy. The hair may regrow a lighter or darker colour and is often curlier initially. Doxorubicin and cyclophosphamide are the commonest culprits. Patients should be offered wigs, which are available from the National Health Service (NHS). Scalp cooling (below 22°C) may reduce alopecia by causing vasoconstriction and reducing circulation to hair follicles. The pharmacokinetic profiles of cytotoxics dictate that scalp cooling is only effective for anthracyclines. Concerns have been raised over the potential risk of developing scalp and cerebral metastases due to reduced drug circulation to these sites with scalp cooling. Along with alopecia, a frequent complication of chemotherapy is onychodystrophy or nail changes other than colour changes that usually make the nails brittle and prone to shedding (onycholysis) as well as fungal infection (onychomycosis). A common physical sign in patients who have received cyclical chemotherapy are Beau’s lines, horizontal grooves or lines on the nail plate that indicate cycles of arrested nail growth with chemotherapy cycles (Figure 3.20). Figure 3.20 Beau lines. Horizontal lines in nails represent arrest of nail growth due to cycles of chemotherapy. The myelosuppressive effects of chemotherapy may affect the circulating red cells, white cells and platelets, and the manifestations in these three series are in part related to their circulatory lifespans. In circulation, the half-life of an erythrocyte is 120 days, of a leucocyte is 6–8 hours and of a platelet is 7 days. There is a significant risk of severe myelosuppression if chemotherapy is initiated when the total white cell count is <3.0 × 109/L (or the neutrophil count is <1.5 × 109/L) and/or the platelet count is <150 × 109/L. These values are the usual cut-offs for administering a cycle of chemotherapy; however, it may be given at lower values in patients with haematological malignancies or if supportive therapy is anticipated and when non-myelosuppressive regimens are employed. Myelosuppression is the DLT for many cytotoxic agents; the main exceptions are vincristine, bleomycin, streptozotocin and asparaginase, which do not cause myelosuppression. Thus, anaemia is rarely a DLT but is generally cumulative over successive cycles of chemotherapy. Anaemia is most troublesome with cisplatin because the nephrotoxicity of this agent may decrease erythropoietin production from the kidneys in response to anaemia. The symptoms of anaemia include fatigue, lethargy and exertional dyspnoea with haemoglobin levels in the range 80–100 g/L. Reduced exercise capacity progresses to dyspnoea and tachycardia at rest and complications including cerebrovascular (e.g. transient ischaemic attacks) and cardiovascular (e.g. angina) ischaemia as the haemoglobin falls below 80 g/L. The management of chemotherapy-induced anaemia is with transfusion, and in the case of cisplatin-induced anaemia, at least, erythropoietin may be beneficial (Box 3.3).
The principles of cancer treatment
Appropriate care
Cancer staging
How to stage a tumour
Radiology techniques
Computed tomography
Magnetic resonance imaging
Positron emission tomography
Isotope
Half-life
Tracer
Oncological use
99Tc (technetium)
6 hours
Methylene diphosphonate (MDP)
Bone scan
111In (indium)
67 hours
Octreotide
Neuroendocrine tumours
131I (iodine)
8 days
Sodium iodide
Thyroid cancer
131I (iodine)
8 days
Meta-iodobenzylguanidine (MIBG)
Phaeochromocytoma & neuroblastoma
67Ga (gallium)
68 hours
Gallium citrate
Lymphoma
Radio-isotope scanning
Performance status
ECOG functional capacity grading
0
Asymptomatic
1
Symptomatic but fully ambulant
2
Symptomatic; ambulant >50% waking hours
3
Symptomatic; confined to bed >50% waking hours
4
Symptomatic; bed bound
Karnofsky performance status score (%)
100
Normal; no complaints; no evidence of disease
90
Able to carry on normal activity; minor signs or symptoms
80
Normal activity with effort; some signs or symptoms
70
Care for self; unable to carry on normal activity or do active work
60
Requires occasional assistance, but able to care for most of needs
50
Requires considerable assistance and frequent medical care
40
Disabled; requires special care and assistance
30
Severely disabled; hospitalization indicated but death not imminent
20
Very sick; hospitalization necessary; active supportive treatment necessary
10
Moribund; fatal processes progressing rapidly
Stroke
Heart failure
Prostate cancer
Breast cancer
5-year survival rate
50%
62%
50–99%
73–89%
Prognosis: it is not cancer, is it doc?
Surgical oncology
Year
Surgeon
Operation
1881
Albert Billroth
Subtotal gastrectomy
1890
William Halsted
Radical mastectomy
1897
Carl Schlatter
Total gastrectomy
1898
Johann von Mikulicz
Oesophagogastrectomy
1900
Ernest Wertheim
Radical hysterectomy
1906
W. Ernest Miles
Abdominoperineal excision of rectum
1913
Franz Torek
Oesophagectomy
1913
Wilfred Trotter
Partial pharyngectomy
1933
Evarts Graham
Pneumonectomy
1935
A. O. Whipple
Pancreaticoduodenectomy
Surgical cancer prophylaxis
Year
Surgeon
Operation
1896
George Beatson
Oophrectomy for breast cancer
1941
Charles Huggins and Clarence Hodges
Orchidectomy for prostate cancer
1951
Rolf Luft and Herbert Olivecrona
Hypophysectomy for breast cancer
1952
Charles Huggins and D. M. Bergenstal
Adrenalectomy for breast cancer
Surgical diagnosis and staging of cancer
Indication
Prophylactic operation
Cryptorchidism
Orchiopexy
Polyposis coli/chronic ulcerative colitis
Colectomy
Familial medullary thyroid cancer (MEN 2 and 3)
Thyroidectomy
Familial breast cancer (BRCA 1 and 2)
Mastectomy
Familial ovarian cancer (BRCA 1 and 2)
Oophrectomy
Surgical treatment of cancer
Surgery for oncological emergencies
Surgical palliation of cancer
Surgical reconstruction following cancer therapy
1. Healing by secondary intent
2. Primary closure
3. Delayed primary closure
4. Split-thickness graft
5. Full-thickness skin graft
6. Tissue expansion
7. Random pattern graft
8. Pedicled flap
9. Free flap with vascular microsurgery
Radiotherapy
The measurement of radiation
External beam radiotherapy
Brachytherapy
Radioisotope therapy
Source
Half-life
Mean X-ray energy
Form
Cobalt-60
5.3 years
1.25 MeV
Pellets (beads, tubes, needles)
Caesium-137
30 years
0.66 MeV
Tubes, needles
Iridium-92
74 days
0.37 MeV
Wires, hairpins, cylinders
Iodine-125
60 days
0.03 MeV
Grains, seeds
Radioisotope
Decay
Uses in oncology
Iodine-131
Beta: 192 keV
Used in treating thyroid cancer and in imaging the thyroid gland
Phosphorus-32
1.71 MeV
Used in the treatment of polycythemia vera
Rhenium-188
2.12 MeV
Used to irradiate coronary arteries via an angioplasty balloon and in relieving the pain of bone metastases
Samarium-153
Beta: 825 keV
Used in relieving the pain of bone metastases
Strontium-89
1.481 MeV
Used in relieving the pain of bone metastases
Toxicity of radiotherapy
Tissue
Radiation effect
Dosage (Gy)
Testis
Sterility
0.2
Eye
Cataract
10
Lung
Pneumonitis
20
Kidney
Nephritis
25
Liver
Hepatitis
30
Central nervous system
Necrosis
50
Gastrointestinal tract
Ulceration, haemorrhage
60
Timing
Tissue
Reaction
Early reactions
Skin
Dermatitis
Oral mucosa
Stomatitis
Bladder
Cystitis
Oesophagus
Oesophagitis
Bowel
Diarrhoea, ulceration
Bone marrow
Myelosuppression
Late reactions
Central nervous system
Necrosis
Kidney
Nephritis
Liver
Hepatitis
Lung
Pneumonitis and fibrosis
Vascular endothelium
Fibrosis
Radiosensitivity and radioresistance
Chemotherapy
Drug discovery
Mechanisms of cytotoxic drugs
Alkylating agents
Antimetabolites
Intercalating agents
Spindle poisons
Functional group
Chemical group
Examples
Alkylating agents
Nitrogen mustards
Chlorambucil
Nitrosoureas
BCNU (carmustine)
Tetrazine compounds
Dacarbazine
Aziridines
Mitomycin C
Methane sulphonic esters
Busulphan
Antimetabolites
Purine analogues
6-Mercaptopurine
Pyrimidine analogues
Cytarabine
Dihydrofolate reductase inhibitors
Methotrexate
Thymidylate synthetase inhibitors
5-Fluorouracil
Ribonucleotide reductase inhibitors
Hydroxyurea
Intercalating agents
Platins
Cisplatin
Antibiotics
Doxorubicin
Anthraquinones
Mitoxantrone
Others
Bleomycin
Spindle cell poisons
Vinca alkaloids
Vincristine
Taxanes
Paclitaxel
Topoisomerase inhibitors
Topoisomerase I inhibitors: camptothecins
Topotecan
Topoisomerase II inhibitors: epipodophyllotoxins
Etoposide
Topoisomerase inhibitors
Chemotherapy resistance
Chemosensitivity
Tumour
Sensitive and curable
Leukaemias
Sensitive and normally incurable (radical palliation)
Small cell lung cancer
Moderately sensitive (palliation or adjuvant treatments)
Breast cancer
Low sensitivity (chemotherapy of limited use)
Kidney cancer
How chemotherapy is used
Therapy
Tumour
Cancers effectively treated by neoadjuvant chemotherapy
Soft tissue sarcoma
Cancers effectively treated by adjuvant chemotherapy
Wilms’ tumour
Disease
Stage
Transplant
Approx. 5-year disease-free survival
CML
Stable phase
Allogeneic
30%
ALL
Second remission
Allogeneic/autologous
40%
AML
First remission
Allogeneic/autologous
50%
High-grade non-Hodgkin’s lymphoma
Responsive relapse
Autologous
45%
Hodgkin’s disease
Responsive relapse
Autologous
45%
Neuroblastoma
High-risk first line
Allogeneic/autologous
50%
Neuroblastoma
Relapsed
Allogeneic/autologous
25%
Non-seminomatous germ cell tumour
Responsive relapse
Autologous
50%
Myeloma
First line
Allogeneic/autologous
30%
Side effects of chemotherapy
Early side effects
Nausea and vomiting
Anaphylaxis
Extravasation
Tumour lysis
Delayed side effects (predictable)
Alopecia and onychodystrophy
Myelotoxicity of chemotherapy
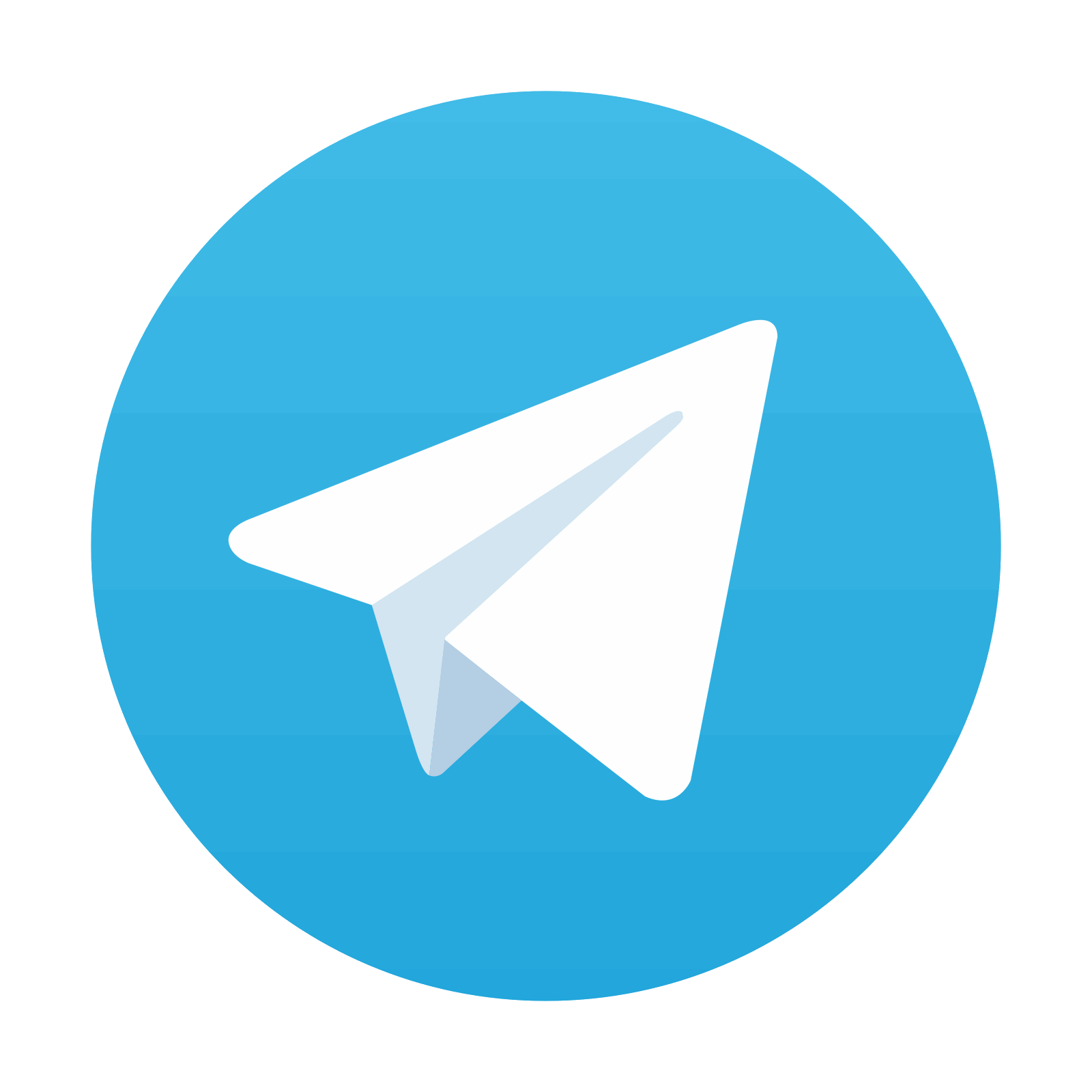
Stay updated, free articles. Join our Telegram channel

Full access? Get Clinical Tree
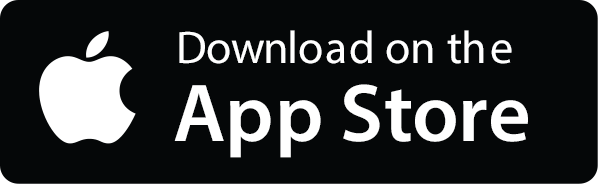
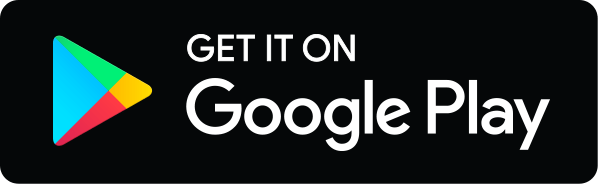