- Nutrition and diet play a key role in the development of atherosclerosis, hypertension, and heart failure.
- These diseases are currently the leading cause of death in developed countries and are set to become the most common cause of death worldwide in the next 2 decades.
- The amount and the type of dietary fat consumed in the diet play a fundamental role in the development and management of atherosclerosis.
- An increased plasma concentration of low-density lipoprotein (LDL) cholesterol is a key risk factor for the development of coronary heart disease, and reducing LDL levels, by diet or by drugs, is a fundamental goal of disease prevention.
- Lifestyle changes such as dietary modification are useful adjuncts to antihypertensive drug therapy in individuals with hypertension and, if adopted on a large scale, may reduce the incidence of coronary heart disease and stroke in the population.
- Malnutrition (cancer cachexia) can be seen in up to 30% of patients with severe chronic heart failure.
- Although the results of trials using vitamin and mineral supplements have been disappointing, achieving a high intake of beneficial micronutrients through diets rich in fruit and vegetables, such as the ‘Mediterranean-style diet’, may contribute to the reduced incidence of coronary heart disease.
18.1 Introduction
Diseases of the heart and blood vessels are the most common causes of death in developed countries and over the next 20 years will become the most important cause of death worldwide. The evidence that nutritional factors are central to the aetiology of cardiovascular disorders such as atherosclerosis, hypertension, and cardiac failure is compelling. Diet therefore plays a key role in their management.
Atherosclerosis underlies the majority of vascular disorders, as illustrated in Figure 18.1. Atherosclerotic diseases, and in particular coronary heart disease, form the focus of this chapter. Knowledge of the pathobiology of atherosclerosis is vital to understanding the ways in which nutrients may be implicated in its development, and the chapter begins with a review of the processes involved.
Dietary fats are the most important nutritional determinants of coronary heart disease and the evidence for the principal role of saturated fat is discussed in depth. The evolving roles of monounsaturated and polyunsaturated fatty acids are also explored. Plasma lipoproteins are thought to be integral to the link between dietary fats and vascular disease, and a summary is provided of lipoprotein types, lipoprotein metabolism, and the common dyslipidaemias. An overview of lipid-lowering drugs is accompanied by a summary of the landmark clinical trials that support the benefits of these agents.
Recent studies highlight the importance of factors other than dietary saturated fats and plasma lipids in the prevention of coronary heart disease. The roles of antioxidants, proteins, and alcohol are discussed. In particular, the evidence for a protective effect of a Mediterranean-style diet and a diet high in fish oils is discussed.
This chapter also summarises the role of dietary factors in the aetiology of hypertension, stroke, and peripheral vascular disease and discusses the nutritional aspects of chronic heart failure, one of the most frequent sequelae of coronary heart disease. The chapter concludes with an outline of the contribution of micronutrients to cardiovascular disorders.
18.2 Atherosclerosis
Atherosclerosis is the pathological process that underlies the majority of vascular diseases. The atherosclerotic plaque is a collection of lipid, inflammatory cells, smooth-muscle cells, and fibrous tissue within the vessel wall. Atherosclerotic plaques may lead to clinical problems by two principal mechanisms. First, bulky plaques encroach upon the vessel lumen, restricting blood flow by reducing the vessel internal diameter, and lead to ischaemia in organs and tissues supplied by that vessel. Second, plaques may rupture and stimulate localised platelet aggregation and clot formation, which cause symptoms either by embolising and occluding smaller vessels downstream or by occluding the vessel at the site of the plaque and causing ischaemia or infarction of the target organ.
The arterial wall consists of three layers: the intima, media, and adventitia (see Figure 18.2). Atherosclerosis is primarily a disease of the innermost layer, the intima, which comprises smooth-muscle cells lined by a single layer of endothelial cells. The endothelium provides a barrier between the constituents of circulating blood and the remainder of the vessel wall. The importance of the endothelium, however, far exceeds its role as a physical barrier. Endothelial cells maintain vascular homeostasis by secreting a number of locally acting substances, which exert effects on neighbouring cells in the vessel wall and cells within the vessel lumen. Endothelial production of substances such as nitric oxide, which promote vasodilatation and inhibit platelet aggregation, is of vital importance in maintaining vessel health.
The presence of coronary-heart-disease risk factors leads to the loss of the protective actions of the endothelium, triggering the development of atherosclerosis. One of the earliest events in this process is the adhesion of circulating leukocytes (monocytes) to dysfunctional/damaged endothelium. This process is facilitated by the expression of binding proteins known as adhesion molecules on the surface of dysfunctional endothelial cells. Once bound, adherent monocytes migrate into the intima and transform into macrophages, and are joined by other leukocytes, such as T lymphocytes, in mediating a complex inflammatory response. The macrophages within the intima avidly take up lipid and change into lipid-laden foam cells, giving rise to a lesion known as the fatty streak. Fatty streaks are the earliest visible manifestation of atherosclerosis and are detectable in the aorta and coronary arteries in humans from a very young age.
Inflammatory cells within fatty streaks secrete cytokines and growth factors, whcih fuel the gradual progression of the fatty streak to a mature atherosclerotic plaque. Platelets adhering to adjacent endothelium provide another source of cytokines and growth factors. Smooth-muscle cells migrate from the media to the intima in response to growth factors and form the bulk of the plaque. Macrophages continue to accumulate lipid, principally in the form of cholesterol esters. The cholesterol is taken up into the vessel wall from the circulation, where it is carried by specialised proteins known as lipoproteins.
Low-density lipoprotein (LDL) is the most atherogenic circulating lipoprotein. This is most avidly taken up by macrophages in the plaque after it has been oxidised. LDL oxidation, therefore, is a crucial step in atherosclerosis, and increased ‘oxidant stress’ in the vascular wall is a feature of many pro-atherosclerotic states (such as diabetes mellitus, smoking, and hypertension).
A mature atherosclerotic plaque consists of a connective tissue cap, smooth-muscle cells, macrophages, and other inflammatory cells, and a central core of lipid-rich, necrotic debris (see Figure 18.2).
Two main types of plaque are described. ‘Stable’ plaques have a thick (often calcified) fibrous cap, which has a low propensity to rupture, and they form a chronic obstruction to blood flow. ‘Unstable’ plaques have a thin, friable cap, which is prone to rupture, exposing the potent thrombogenic lipid core to blood within the vascular lumen. When these plaques rupture, platelet aggregation and subsequent initiation of the clotting cascade leads to thrombus formation. Unstable plaques are typically less bulky and encroach less upon the vessel lumen than stable plaques, and they may be asymptomatic until plaque rupture occurs. Following rupture, however, thrombus may completely occlude the vessel and lead to infarction in the territory supplied by that vessel.
Figure 18.3 illustrates the way in which nutritional factors may interact with the multiple biological processes involved in the development of atherosclerosis.
18.3 Dietary lipids and coronary heart disease
There is compelling evidence that dietary lipids play a fundamental role in the development of atherosclerosis. These data have accrued over many years from a combination of epidemiological studies revealing an association between dietary fat consumption and the incidence of coronary heart disease, experimental studies showing that feeding lipids to animals causes atherosclerosis, and studies in which changing lipid consumption in humans reduces the risk of disease.
Before these are discussed, it is necessary to review the principal types of dietary fat and how they are classified.
Types of dietary lipid
Lipids are hydrocarbon-based organic molecules that are insoluble in water. Figure 18.4 illustrates the way in which lipids are classified as ‘simple’ or ‘complex’ depending on whether they are unmodified or have been esterified by the addition of a molecule containing an alcohol group. Simple lipids are further divided into saturated, monounsaturated, and polyunsaturated forms, depending upon the number of double bonds within their structure (see Figure 18.4). Table 18.1 shows the main dietary sources of these fats.
Biochemically, fatty acids are described by a numerical code, which defines the following:
- the number of carbon atoms within the molecule;
- the number of double bonds;
- the position of the first double bond, as counted from the terminal carbon atom.
For example, oleic acid is described as (18:1,n-9) because it contains 18 carbon atoms and 1 double bond, with the double bond situated 9 carbon atoms from the terminal end.
Table 18.1 Food sources of types of dietary fat.
Type of fat | Food source |
Saturated fats | Dairy products Beef Lamb Poultry Pork |
Monounsaturated fats | Olive oil Canola oil |
Polyunsaturated fats | Margarines Vegetable oils (corn, sunflower, safflower, soy) Fatty fish and fish oils |
Cholesterol | Egg yolks Meats Dairy products |
The link between saturated fat consumption and coronary heart disease
Epidemiology
Cross-population studies allow the identification of factors which may predispose to coronary heart disease in groups with substantially different diets and rates of coronary heart disease. The Seven Countries Study, which began in 1958, was a classic cross-population study in which the relationship between dietary composition and coronary-heart-disease mortality was assessed in more than 12 000 men (aged 40–59 years) living in Japan, Italy, Greece, the Netherlands, the former Yugoslavia, Finland, and the USA. The study demonstrated that mortality from coronary heart disease is strongly related to the mean serum cholesterol level in a population, which in turn is strongly associated with the average intake of saturated fat (Figure 18.5).
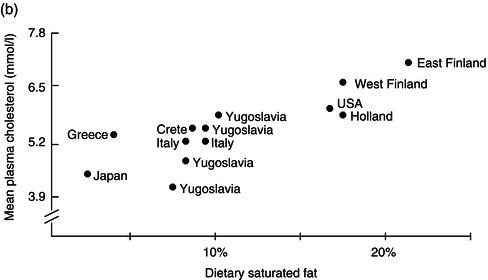
Comparison of coronary-heart-disease rates between populations suffers from a number of potential confounding effects, including genetic differences between the populations studied. This was addressed by the Ni Hon San Study, which followed migrants from Japan (Nippon) to Hawaii (Honolulu) and California (San Francisco). Japanese migrants living in Honolulu and San Francisco consumed significantly greater proportions of total fat in the diet, and had higher mean total cholesterol levels, than Japanese natives. This was associated with a significant increase in coronary-heart-disease mortality rates in the migrants (Figure 18.6).
Further studies performed within populations are consistent with cross-population studies in showing a significant correlation between consumption of total lamb and saturated fat and coronary-heart-disease mortality. Within-population studies have also been instrumental in determining which individual risk factors predispose to coronary heart disease. For example, long-term follow-up of residents of Framingham, a small town in the USA, has identified several important predictors of increased coronary-heart-disease risk. These include total cholesterol, LDL cholesterol, high-density lipoprotein (HDL) cholesterol (inversely related to coronary heart disease), hypertension, smoking, and diabetes. Framingham data have been used to construct risk-factor charts and scoring systems to predict the risk of coronary heart disease in a given individual. While caution must be employed in extrapolating data from a single population to other, potentially different populations, such tools have proved extremely useful in clinical practice.
A number of other within-population studies have strengthened the evidence of an association between plasma cholesterol levels and coronary heart disease. The Multiple Risk Factor Intervention Trial (MRFIT) is of particular relevance, as it studied over 360 000 men. The data demonstrate convincingly that there is a continuous and graded relationship between serum total cholesterol and coronary-heart-disease mortality (Figure 18.7).
Figure 18.7 The relationship between plasma cholesterol and death from coronary heart disease (the MRFIT Study). Adapted from Martin et al. (1986), copyright 1986, with permission from Elsevier.
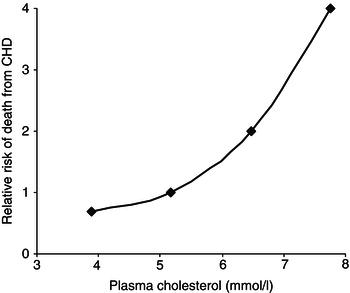
The British Regional Heart Study followed 7735 men chosen at random from general practices in 24 towns in England, Scotland, and Wales to assess the impact of variations in personal, economic, and social factors on coronary heart disease. Again, there was strong, graded association between total cholesterol and the risk of a major coronary event, even after adjustment for other risk factors.
Experimental evidence
Further support for the idea that dietary fats play a role in the development of atherosclerosis comes from studies in animals, which demonstrate a direct link between consumption of saturated fat, increased plasma cholesterol levels, and atherosclerosis. Feeding animals such as rabbits and non-human primates a diet high in saturated fat leads to predictable elevations of total and LDL cholesterol and subsequent development of vascular intimal lesions characteristic of human atherosclerosis. Furthermore, reduction of blood cholesterol levels by dietary modification leads to regression of established atherosclerosis in these animals.
Animal studies also show that certain fats, particularly the long-chain saturated fatty acid stearic acid, are able to induce platelet aggregation and thrombus formation. In contrast, diets rich in (n-3) polyunsaturated fatty acids reduce the incidence of arterial thrombosis in animals and lower the predisposition to fatal cardiac-rhythm disturbances in models of coronary artery occlusion.
The effect of reducing saturated-fat consumption on the risk of coronary heart disease
Although epidemiological and experimental studies provide persuasive evidence for a major role of saturated fat in the development of coronary heart disease, proof of its involvement requires that dietary modification, such as a reduction in the consumption of saturated fat, leads to a decrease in the incidence of coronary heart disease.
In fact, a large number of clinical trials performed over the last half century have demonstrated that diets low in saturated fat reduce the incidence of coronary events. As it is difficult in clinical trials to precisely regulate the food intake of free-living subjects in the community, many diet-intervention studies have been centred on residents living in institutions or hospitals.
In the first large-scale trial of an experimental diet, the Finnish Mental Hospital Study, over 10 000 patients in psychiatric hospitals were given a diet low in saturated fat. This led to a significant reduction in plasma cholesterol and coronary-heart-disease mortality.
The Los Angeles Veterans Administration Study randomly assigned men living in a war veterans’ home to an experimental diet low in saturated fat or to a control diet. Subjects consuming the experimental diet experienced a 31% decrease in cardiovascular events.
The Oslo Trial investigated the effects of dietary and smoking advice in 1232 healthy men aged 40–49 years. These men, who were at high risk of cardiovascular disease due to a high prevalence of hypercholesterolaemia and cigarette smoking, were randomly allocated either to an intervention group, who received dietary and antismoking advice, or to a control group, who did not receive any advice. The recommended diet was low in saturated fat and high in fibre. During the course of the study, there was a 47% reduction in the incidence of myocardial infarction and sudden death in the intervention group.
Not all intervention studies of a diet in saturated fat have yielded positive results. For example, the Minnesota Coronary Survey involved feeding 9057 men and women living in psychiatric hospitals and nursing homes in Minnesota a low-saturated fat diet. Despite a reduction of 14% in mean serum cholesterol, there were no significant differences in the rates of myocardial infarction and sudden cardiac death. It is possible that the negative results reflect a low baseline cholesterol and young age of the study population.
Moreover, saturated-fat intake in this study was replaced by n-6 polyunsaturated fatty acids. It is now recognised that increasing consumption of n-6 fatty acids without increasing n-3 fatty acids may unfavourably disrupt the balance of eicosanoid production and offset the benefits of LDL reduction (see later). Such a diet is no longer recommended.
Analysis of pooled data strongly suggests that the magnitude of benefit gained by reducing saturated fat intake is strongly dependent on the replacement nutrition. For example, while replacing saturated fat with polyunsaturated fat (preferably of both n-6 and n-3 varieties, as discussed earlier) appears to correlate with a significant reduction in the risk of developing cardiovascular disease, replacing saturated fat with a carbohydrate appears to have no impact on the same end point. Whether this effect encompasses refined carbohydrates and those deemed to have a low glycaemic index is less clear. The effect of replacing saturated fat with monounsaturated fat is unclear.
The effect of saturated fats on plasma cholesterol and atherosclerosis
The principal way in which saturated fats contribute to atherosclerosis is likely to be by increasing plasma concentrations of LDL cholesterol. This is mediated, at least in part, by a decrease in LDL-cholesterol catabolism, possibly by saturated fats inducing a reduction in LDL receptor numbers or causing decreased cell-membrane fluidity. LDL is taken up from the circulation into the vessel wall, where it makes up an important part of the atherosclerotic plaque. Macrophages within the plaque become activated after acquiring oxidised LDL and secrete a number of substances, which may further promote atherosclerosis progression.
Saturated fats vary in their ability to raise plasma LDL cholesterol. Myristic and palmitic acids have been reported to possess the greatest cholesterol-raising potential, whereas stearic acid has little effect on plasma cholesterol levels (although interestingly, it may still be atherogenic).
Dietary cholesterol and coronary heart disease
Cholesterol is a component of a normal diet and is present at high levels in foods such as eggs, dairy products, and red meats. Cholesterol esters form a fundamental part of the atherosclerotic plaque and it has been known for many years that feeding animals with a diet high in cholesterol leads to atherosclerosis. Moreover, epidemiological studies reveal a strong positive relationship between the intake of dietary cholesterol and the incidence of coronary heart disease in humans. Interpretation of these data, however, is complicated by the fact that cholesterol-rich foods are often also high in saturated fats. The strong association between dietary saturated fats and coronary heart disease has been discussed. In fact, dietary cholesterol appears to be a less potent determinant of plasma cholesterol levels than are the dietary fatty acids, particularly saturated fatty acids. The effect of lowering dietary cholesterol intake independently of changes in saturated-fat consumption on coronary-heart-disease incidence has not been studied.
Nonetheless, most dietary strategies for protection from coronary heart disease recommend a reduction in intake of both saturated fat and dietary cholesterol. It is important, however, to be aware of the distinction between a ‘cholesterol-lowering’ diet and a ‘low-cholesterol’ diet.
Dietary polyunsaturated fats and coronary heart disease
Dietary polyunsaturated fats are divided into two main families – n-6 and n-3 – according to the position of the double bond in their molecular structure. Linoleic acid (n-6) and alpha-linolenic acid (n-3) are found in plants and vegetable oils and are termed ‘essential fatty acids’ as they are not synthesised by humans. Once ingested, however, these essential fatty acids are biochemically processed (by desaturation and elongation) to form long-chain fatty acids (Figure 18.8). Long-chain polyunsaturated fatty acids are also contained in the diet, for example in fish oils.
Long-chain polyunsaturated fatty acids are important in the synthesis of eicosanoids: potent biologically active substances which act locally within cells and tissues to regulate processes such as vessel tone, inflammation, and platelet aggregation. n-6 and n-3 fatty acids compete for enzymes in each other’s metabolic pathways, so that high levels of linoleic acid, for example, may inhibit the conversion of linolenic acid to its longer-chain forms. The relative abundance of n-6 and n-3 polyunsaturated fatty acids in the diet, therefore, may determine the relative production of pro- and anti-atherosclerotic eicosanoids. The balance between dietary n-6 and n-3 fatty acids is also important, as the two families compete for incorporation into cell membranes. n-3 fatty acids exert beneficial effects on membrane fluidity and ion transport, and may lead to clinically relevant cardioprotective effects (see Section 18.6).
Polyunsaturated fatty acids have traditionally been used as substitutes for saturated fatty acids in low-saturated fat diets. When the potential health implications of reducing saturated-fat intake first became apparent, the food industry replaced many traditional sources of fat (such as lard and butter) with vegetable oils and margarines. These were principally derived from sunflower, safflower, and corn oils, which are high in linoleic acid. The n-6 fatty acid content of the UK diet, therefore, has risen substantially over the last 20 years, without a corresponding increase in n-3 fatty acids. Although such a pattern is effective in reducing plasma LDL cholesterol, there are concerns that a high ratio of dietary n-6 to n-3 fatty acids may not reduce cardiovascular risk where the overall intake of n-3 fats is low. The benefits of increasing the intake of n-3 fatty acids have become apparent in the light of recent clinical trials (see Section 18.6).
Foods rich in n-3 fatty acids include seed oils (e.g. linseed oil, rapeseed oil, soya oil, walnut oil), nuts (e.g. walnuts, peanuts), meat (e.g. beef), green leafy vegetables (e.g. spinach), and oily fish (e.g. tuna, salmon, mackerel, sardines, herring).
Dietary monounsaturated fats and coronary heart disease
Monounsaturated fatty acids contain one double bond in their molecular structure and are found in all animal products and vegetables. Rich dietary sources include olives, rapeseed, avocado, nuts, meat, and peanut oil. Early studies suggested that dietary monounsaturated fatty acids had a neutral effect on plasma lipoprotein levels. More recently, however, it has become apparent that monounsaturates, when substituted for dietary saturated fatty acids, lead to reductions in LDL cholesterol and triacylglycerols (TAGs) and small increases in HDL cholesterol.
Oleic acid is present in large amounts in olive oil, an important source of dietary lipid in Mediterranean countries. The long life expectancy and low risk of coronary heart disease in some Mediterranean countries, such as Greece, has led to the promotion of a Mediterranean-style diet as being cardioprotective. The Mediterranean-style diet is dealt with in detail in Section 18.6.
Dietary trans–fatty acids and coronary heart disease
Fatty acids exist in two molecular configurations, known as cis– and trans-isomers. Vegetable oils largely consist of cis-fatty acids, in which the hydrogen groups are on the same side of the carbon chain (Figure 18.9). In contrast, trans-isomers contain hydrogen moieties on opposite sides of the carbon chain. Trans-fatty acids are present naturally at low levels in the diet, but the majority are formed artificially by the hydrogenation of vegetable oils. Hydrogenation converts liquid vegetable oils to a semi-solid state and is widely used in the manufacture of hard margarines and other processed foods. Diets high in trans-fatty acids do not have a beneficial effect on the lipoprotein profile and may, in fact, lead to elevation of LDL- and reduction of HDL-cholesterol levels.
There is now significant evidence that trans-fatty acid consumption is linked to the development of coronary heart disease. Analysis of data obtained from the Nurses’ Health Study suggests that for every 2% increase in calories obtained from trans-fats as opposed to carbohydrates, the risk of developing coronary heart disease roughly doubles. An extrapolation of these data would suggest that trans-fats could account for between 30 000 and 100 000 excess deaths from coronary heart disease in the USA per annum.
As a result of this overwhelming evidence, many Western countries have set up guidelines suggesting significant restriction on the use of trans-fats in products. In 2003, for example, Denmark set an upper limit for artificial trans-fat levels of 2 g per 100 g of oil or fat, which has led to the virtual elimination of trans-fats in Denmark. Irrespective of guidelines placed upon them by nations, many large manufacturers and retailers have banned the use of trans-fats in their products.
It should be mentioned that while the evidence strongly supports a restriction on the use of artificially manufactured trans-fats, there is some evidence that consumption of naturally occurring trans-fats, such as those found in the meat of ruminant animals, may not have the same adverse effects and in fact may have a beneficial effect on lipid profile.
18.4 Plasma lipoproteins
An increased plasma concentration of LDL cholesterol is a key risk factor for the development of coronary heart disease, and reducing LDL levels, by diet or drugs, is a fundamental goal of disease prevention. In recent years, the importance of more subtle changes in the plasma lipid profile has emerged. Although a detailed description of plasma lipoproteins is beyond the scope of this chapter, a basic knowledge of their structure and metabolism is integral to understanding the mechanisms of cardiovascular risk reduction.
The two major lipids in plasma, cholesterol and TAG, are insoluble in an aqueous environment and therefore circulate in association with specialised proteins (apoproteins) in complexes known as lipoproteins. Lipoproteins consist of a central lipid core of cholesterol esters and TAGs, surrounded by an outer layer comprising phospholipids, free cholesterol, and apoproteins (Figure 18.10). Apoproteins play an important role in lipoprotein metabolism by interacting with cellular receptors and enzymes.
Table 18.2 Composition of plasma lipoproteins. TAG, triacylglycerol; VLDL, very low-density lipoprotein; IDL, intermediate-density lipoprotein; LDL, low-density lipoprotein; HDL, high-density lipoprotein.
Plasma lipoproteins are classified into five groups according to their density, which determines the way the lipoproteins separate when subjected to ultracentrifugation. Table 18.2 shows the ways in which lipid distribution and apoprotein composition vary between the lipoprotein classes.
- LDL cholesterol makes up approximately 70% of total serum cholesterol. It contains a single apoprotein, apo B. LDL is the major atherogenic lipoprotein, and delivers cholesterol to the vessel wall, where it is incorporated into atherosclerotic plaque. Oxidation of LDL significantly increases its atherogenicity.
- HDL cholesterol normally makes up 20–30% of the total serum cholesterol. HDL levels are inversely correlated with the risk of coronary heart disease. HDL helps to protect against atherosclerosis, mainly by carrying cholesterol away from the vessel wall to the liver, in a process known as reverse cholesterol transport.
- Very low-density lipoproteins (VLDLs) are TAG-rich lipoproteins, but contain 10–15% of serum cholesterol. VLDLs are produced by the liver and are precursors of LDLs. VLDLs may be degraded in the circulation to form VLDL remnants, which are rich in cholesterol and may contribute to atherosclerosis.
- Intermediate-density lipoproteins (IDLs) are produced by the catabolism of VLDLs. Their role in atherosclerosis is not well defined. In clinical practice, IDL is measured as part of the LDL fraction.
- Chylomicrons are TAG-rich lipoproteins. They are formed in the intestine from dietary fat and pass into the bloodstream. When partially degraded, chylomicrons (then known as chylomicron remnants) may carry some atherogenic potential.
18.5 Lipoprotein metabolism
The plasma lipoproteins transport fats within the circulation, which are either absorbed from the intestine or manufactured endogenously. Lipoprotein metabolism is characterised by complex interactions between lipoproteins of different classes, regulatory enzymes, and cellular receptors. These interactions may be simplified by considering three main areas: the exogenous pathway, the endogenous pathway, and reverse cholesterol transport. These are illustrated in schematic form in Figure 18.11.
Figure 18.11 Overview of lipoprotein metabolism. VLDL, very low-density lipoprotein; IDL, intermediate-density lipoprotein; LDL, low-density lipoprotein; HDL, high-density lipoprotein; FFA, free fatty acids.
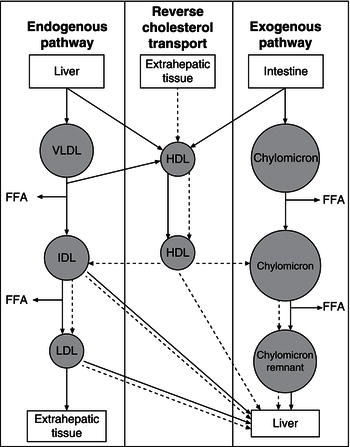
Exogenous pathways
After digestion, dietary cholesterol and fatty acids are absorbed within the intestine and re-esterified to form TAGs and cholesterol esters in intestinal mucosal cells. Here they are packaged with apoproteins, phospholipids, and cholesterol to form chylomicrons. Apolipoprotein B-48, essential for chylomicron formation, is added by the action of microsomal transfer protein. Chylomicrons are secreted into intestinal lymphatics and carried by the thoracic duct into the bloodstream.
Once in the bloodstream, TAGs within chylomicrons are rapidly hydrolysed to free fatty acids and glycerol by lipoprotein lipase, an enzyme bound to vascular endothelial cells within muscle and adipose tissue. The resulting fatty acids are either delivered to muscle as fuel or re-esterified and stored as TAGs in adipose tissue. During the process of TAG hydrolysis, chylomicrons acquire cholesterol ester from other lipoproteins, such as mature HDLs, in exchange for TAGs by the action of cholesterol ester transfer protein. The particles become progressively depleted in TAGs and enriched in cholesterol esters, and acquire apoprotein E from HDL to become chylomicron remnants. These remnants are rapidly cleared from the circulation by the liver, after binding to hepatocyte receptors which recognise apoprotein E.
Endogenous pathway
Cholesterol and TAGs are synthesised by the liver and secreted in VLDL particles for transport to peripheral tissues. Cholesterol is manufactured by a complex synthetic pathway using acetyl conenzyme A (CoA) derived from fatty acid oxidation and carbohydrate metabolism. The rate of cholesterol synthesis is determined by the enzyme hydroxymethyl glutaryl (HMG) CoA reductase. Hepatic TAGs are produced from fatty acids originating in adipose tissue or by lipogenesis from carbohydrates. Newly synthesised TAGs combine with those acquired by chylomicron remnant uptake to be secreted in VLDL. VLDL particles, like chylomicrons, are formed by the packaging together of TAGs, cholesterol esters, cholesterol, phospholipids, and apolipoproteins. Apolipoprotein B-100, the major apolipoprotein of VLDL, is added by microsomal transfer protein.
After release into the bloodstream, TAGs in VLDL are rapidly hydrolysed by lipoprotein lipase to release free fatty acids. During this process, VLDL particles exchange lipids with HDL to form cholesterol-rich IDL particles. IDL particles are either taken up by LDL receptors on the liver, or further metabolised by hepatic lipase to form LDL.
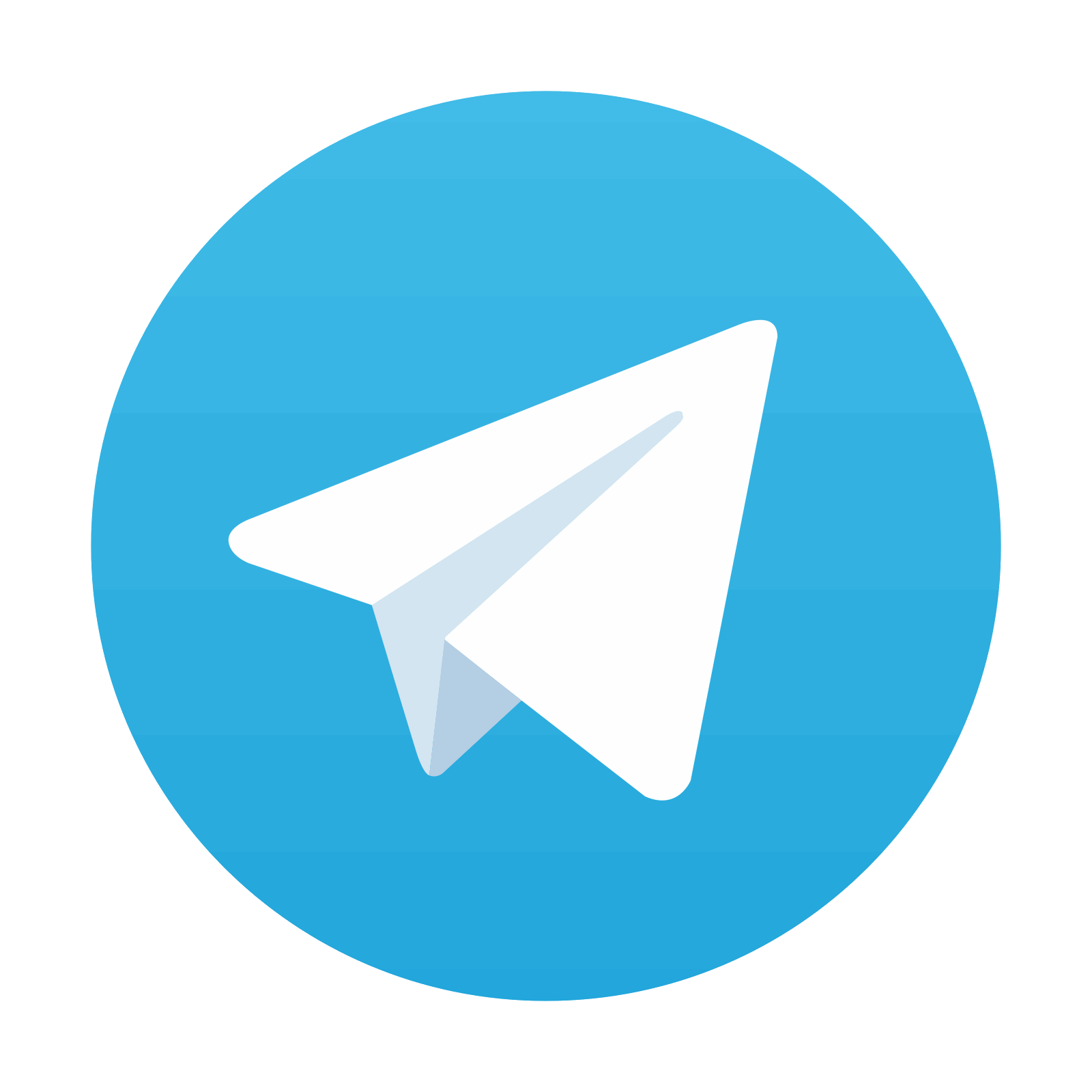
Stay updated, free articles. Join our Telegram channel

Full access? Get Clinical Tree
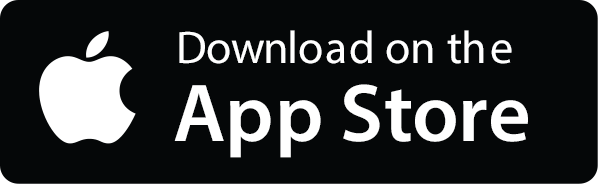
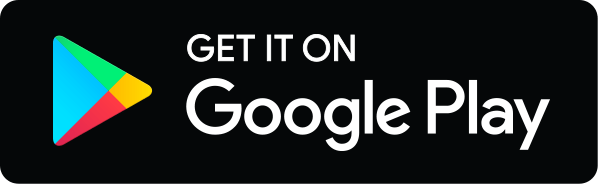