Changes in BCT
The inconvenience of protracted BCT radiation schedules has led several investigators to explore whether it is possible to deliver the needed radiation treatment over a shorter period of time. Whelan [16] and the Start B Trial [6] have demonstrated that 3 weeks of EBRT, with or without a boost, is equivalent to 5 weeks of EBRT for women with moderate-sized breasts (<1,800 ml for the radiation planning volume) in terms of both local control and cosmesis. This 3-week EBRT approach is gradually replacing the standard 5-week EBRT regimen for women who qualify for BCT and are ≥35 years old and who have tumor stage ≤ T2 and nodal status ≤ N1.
Since 3 weeks of EBRT can still be a hardship for many women, researchers are investigating whether the radiation treatment for some women might be reduced even further. Based upon the observation that 80–85 % of breast cancer recurrences happen within the index quadrant in postmenopausal women, a number of investigators are exploring whether partial breast irradiation (PBI) can further shorten the course of radiotherapy. The concept behind PBI is that treating the entire breast (whole breast irradiation or WBI) may result in overtreating some women, thereby increasing toxicity. If equivalent local control in these women can be achieved by irradiating a smaller volume of tissue, the dose to the normal tissues, and thus the toxicities of the treatments, will be reduced.
These efforts have resulted in a variety of different techniques for PBI that employ different geometries in treatment delivery, irradiate different volumes, and have different fractionation schedules. Most of the current PBI approaches are delivered postsurgically, as is the case with standard WBI and hypofractionated treatment, but reduce the overall time of the radiation treatment to a week or less. Due to a shorter course of treatment, these PBI techniques are usually referred to as accelerated PBI or APBI. With IORT APBI, all of the radiation treatment is delivered in one treatment during the lumpectomy.
Non-IORT APBI in Early-Stage Breast Cancer
The oldest APBI method, and one that has the longest follow-up, is multi-plane high-dose-rate (HDR) brachytherapy, pioneered by Polgar in Europe [17] and several centers in the United States [18–21]. This approach involves inserting 14–20 catheters at 1–1.5 cm spacing to cover the lumpectomy cavity in more than one plane. Patients receive between 30 and 36 Gy given over 4–5 days in seven to ten fractions. In highly selected women, in small single-institutional studies, this APBI approach has shown comparable results to WBI, with a 5-year local recurrence rate of 4–5 %. For example, in a single-institution, nonrandomized study of 45 consecutively treated patients, Polgar et al. [22] showed 5- and 10-year local recurrence rates of 4.4 and 9.3 %, respectively, with an overall survival at 12 years of 88.9 %. Of note, all patients had T1 tumors and were node negative. In another randomized trial, Polgar et al. [23] assigned low-risk women (median age 59 years, T ≤ 2.0 cm, pN0, G1 or G2, ER/PR+, negative margins ≥2 mm, DCIS and lobular carcinoma excluded) to either APBI (128 women) or conventional BCT (130 women). The study was stopped prematurely in 2004, before it accrued the necessary 570 patients to determine non-inferiority of this APBI approach with BCT, in order to participate in the larger randomized GEC-ESTRO study [24] of 1,170 women treated with either brachytherapy PBI or conventional BCT. Though underpowered when it was stopped, the difference in LR in the arms proved non-significant (4.7 % for PBI vs. 3.1 % for BCT) with a median follow-up of over 5 years,
Multi-plane brachytherapy requires a high level of skill to deliver, and it is also uncomfortable for many patients. For these reasons, this approach to APBI has not gained as wide an overall acceptance as originally anticipated. A simpler HDR brachytherapy technique, based on a double-lumen balloon catheter that can be inserted into excision cavity, was developed by Mammosite® (Hologic, Marlborough, MA). The balloon catheter technique is discussed in detail elsewhere in this book (Beitsch, “Partial Breast Radiation Therapy”). The ease of use of this device and its relatively high reimbursement led to a rapid adoption of its use in the United States, despite limited evidence of clinical efficacy. While balloon brachytherapy is technically easier, its radiation technique has significantly less volume coverage than either multi-plane brachytherapy or 3D conformal APBI. Holland et al. [25] and Faverly et al. [26] have shown that disease extends up to 2 cm beyond the tumor in more than 20 % of patients. If the APBI volume of breast tissue irradiated is reduced too severely, it is arguable whether limiting the radiation coverage to the excision cavity plus less than 2 cm of adjacent tissue will prove effective in the long term.
Smith et al. [27] analyzed Medicare billing claims for women ≥67 years for IDC diagnosed between 2000 and 2007, treated with BCS, and followed by either Mammosite brachytherapy or WBI. Subsequent mastectomy was used as a surrogate for recurrence and was compared between the two groups. Risks of acute complications (hospitalization or infection within 120 days of treatment) and long-term toxicities (rib fractures, necrosis, breast pain, and pneumonitis) were also compared. A total of 7,291 women received APBI brachytherapy, vs. 123,244 who had WBI. The median FU was 3.84 years for the Mammosite group, and the median age was 75. At 5 years, the cumulative incidence of subsequent mastectomy was 4.0 % in the Mammosite group vs. 2.2 % in the WBI group (p < 0.001). In addition, Mammosite therapy generated more acute complications and toxicities, including hospitalizations (9.6 % vs. 5.7 %, p < 0.001), infections (8.1 % vs. 4.5 %, p < 0.001), rib fractures (4.2 % vs. 3.6 %, p < 0.0010), fat necrosis (9.1 % vs. 3.7 %, p < 0.001), and breast pain (14.9 vs. 11.7 %, p < 0.001). The incidence of radiation pneumonitis was lower in the Mammosite group (0.1 % vs. 0.8 %, p < 0.001). It is possible that the Smith study indicates that inadequate coverage of residual breast disease by the smaller target volume used in balloon brachytherapy can have consequences, but it is also useful because it points out the pitfalls of the rapid acceptance of a breast technique in the absence of adequate long-term clinical data. This is especially true since most women today routinely receive adjuvant hormonal therapy, which will delay recurrences rather than eliminate them.
A third nonoperative APBI approach involves twice daily treatment of the breast using either 3D conformal radiation or intensity-modulated radiation therapy (IMRT). A total of 38.5 Gy is the usual dose prescription delivered twice a day over a 5-day period, though other dose formulations are also being tested. This schedule was deemed equivalent to the conventional approach because it used the linear-quadratic formula, which called for waiting 6 h between fractions. This is an attractive method to explore, because most radiotherapy centers have the ability to provide this APBI approach. However, two recent publications have indicated high levels of unacceptable moderate-to-severe late toxicity (pronounced subcutaneous fibrosis, retraction, telangiectasia) with relatively short median follow-ups of 15 and 30 months, respectively, Jagsi et al. [28] and Hepel et al. [29], but these complications have not been universally reported by other centers [30]. This discrepancy has not yet been reconciled, with further investigation into this approach with an ongoing randomized trial.
In 2005, the NSABP initiated a prospective, randomized trial in the United States to comparatively evaluate these three methods of APBI delivery against conventional WBI [31]. In addition to the NSABP B-39 trial, there is the GEC-ESTRO trial for APBI with multi-plane brachytherapy and two randomized trials in Europe testing 3D conformal ABPI with WBI, called the RAPID trial [32] and the IMPORT trial [33]. These began in 2006 and 2007, respectively. These trials are not yet closed to accrual, and it will be several more years before the data matures sufficiently to evaluate them. Even though the target volumes differ in these studies, they should be very helpful in defining the role of APBI in the management of early-stage breast cancer, further identifying the most suitable patients for these nonsurgical APBI approaches.
Treatment guidelines for radiation oncologists in the United States and Europe have been published by ASTRO [34] and ESTRO [35] in an attempt to identify which patients may be best suited to treat with APBI. However, neither ASTRO nor ESTRO has considered including a single dose of IORT when formulating their guidelines, as the clinical results from trials of IORT were immature and insufficient at the time of their respective publications.
Intraoperative Radiation Therapy Use in Breast Conservation Treatment
IORT using electron beams of radiation (IOERT) was first utilized for breast cancer treatment in the early 1990s attempting to replace the traditional EBRT boost. EBRT boost, given in five to eight fractions, is delivered after 5 weeks of WBI and several months after the lumpectomy. The EBRT boost needs to irradiate the excision cavity, but there can be some uncertainty as to exactly where that cavity is located. There is a potential of the radiation boost missing all or part of the cavity. The rationale for using an IOERT boost is that direct visualization of the tumor bed during IOERT best eliminates any possibility of this geometric miss. Previously, boosting the tumor bed postsurgically was difficult to do well, because it was hard for the radiation oncologist to direct the radiation boost beam to the excision cavity using the less sophisticated imaging systems available. This often required the surgeon to place titanium clips outlining the periphery of the lumpectomy site to aid the radiation oncologist in viewing the excision cavity. In addition to better targeting of the excision cavity, IOERT irradiation delivers a very high biological dose at the time of the surgery, coinciding with the time that residual tumor cells are more rapidly proliferating. IORT also reduces the skin dose (as the radiation is delivered subcutaneously) and saves about 1 week of fractionated EBRT treatments. Finally, with an EBRT boost, the radiation fields used must be larger than with IOERT to account for patient motion (breathing) and for positioning errors that might occur during the multiple patient setups needed to deliver the EBRT boost. The IOERT boost volume is significantly smaller than the EBRT boost volume, resulting in less dose to normal tissues, and, theoretically, should result in less normal tissue toxicity. Table 28.2 compares the advantages of an IOERT boost over that of an EBRT boost.
Table 28.2
Comparison of IORT/IOERT boost vs. EBRT boost
Feature | IOERT/IORT boost | EBRT boost | Advantages of IOERT boost |
---|---|---|---|
Skin dose | None with IOERT; negligible with IORT if 1 cm spacing from applicator to skin | Substantial | Subcutaneous delivery of IORT/IOERT eliminates skin boost dose |
Size of boost volume | Typically 25 mm3 for a 1.5 cm tumor with IOERTa | Typically 50 mm3 for a 1.5 cm tumor | Smaller CTV should result in less toxicity |
# of fractions | 1 treatment; IOERT adds 15–20 min to the surgical time. IORT adds about 40–60 min | 5–8 treatments; does not impact surgical time | IOERT/IORT eliminates 1+ week of treatment |
Accuracy of radiation delivery | No chance for geometric miss of CTVb | Depends on imaging and varies center to center | Direct visualization of target makes IOERT/IORT superior method |
Time of radiation boost | During surgery | 9–40 weeks postsurgeryc | IOERT boost provides 1–2 log cell kill, reducing tumor burden for the postoperative radiation |
Breast size | Irrelevant with IOERT, as delivered directly to tumor bed; IORT challenging for small-breasted women | Large-breasted women more challenging to boost | If volume to boost is deep-seated, more normal tissue must be irradiated, compromising cosmesis |
Dose uniformity | Excellent with IOERTd, poor with IORT | Excellent | Excellent |
Ipsilateral recurrence | IOERT: <0.5 % at 6 years; <1 % at 10 years | Typically 3–4 % at 5 years | Both IOERT and IORT have lower recurrence rate than EBRT boost |
IORT: 1.73 % at 5 years | Typically 7–8 % at 10 years | ||
Cosmesis | 90 % good to excellent at 5 years+ | 70–85 % long-term good to excellent cosmesis | 30 %+ of EBRT women unhappy with breast appearance after BCT |
Despite numerous advantages, IOERT boost for breast cancer was impractical prior to the development of mobile IORT technology. Patients had to either be transported from the operating room to the radiation oncology department, adding an hour or more to the surgical time, or a shielded bunker had to be constructed within the operating room. Such cost was often prohibitively expensive for most hospitals. Mobile IORT technology, using either electron beams or 50 kV X-rays, has now made IOERT boost practical, with more than 10,000 women (mostly in Europe) with breast cancer already receiving IOERT boost. More recently, thousands of women each year now receive IOERT boost, with several more centers realizing the advantages of such technology for the breast cancer patient. Even though improvements in EBRT boost technology and technique have occurred, IORT boost still has advantages as it is now widely recognized that the size and shape of the excision cavity changes over time [36–39]. In fact, the excision cavity imaged many weeks later at the time of the EBRT boost can be quite different from the excision cavity created by the surgeon at the time of the tumor removal. This begs the question of which is the “correct” volume to boost in the first place. Additionally, the increasing trend in oncoplastic reconstruction at the time of BCS compromises the ability of the radiation oncologist to target the lumpectomy cavity, even when surgical clips have been added to guide the boost delivery.
The data collected worldwide utilizing IOERT points to the likely most accurate method of boosting the tumor bed, appearing to be synergistic with the modern surgical and medical oncology approaches for the treatment of breast cancer. As we will see when we discuss clinical results, for patients eligible for BCT, IOERT boost results in 10-year local control rates of 99 %, a figure that is just not achievable with EBRT boost approaches. This higher local control rate should result in improved long-term survival rates [11], with the added advantage of higher rates of breast preservation.
While extending the principles of a radiation boost to IORT, APBI was conceived and initiated before IORT boost results were sufficiently mature. If IORT APBI were proven safe and effective, all of the purported benefits of IORT boost could be achieved with the added bonus of a 1-day treatment. Results to date for IORT APBI have not been an unequivocal success. Nevertheless, IORT APBI will likely continue to be explored in an effort to optimize the technique and to find the select low-risk patients for whom the benefits of a single-day treatment might be appropriate.
Technical Aspects of IORT
Before we discuss the clinical results of IORT, it is important to understand the technical differences in the various approaches that have been used, because they can influence the outcome. There are three IORT techniques that provide APBI at the time of surgery: A single HDR treatment using a specially developed HDR breast applicator (HDR-IORT); 50 kV low-energy X-rays delivered through spherical plastic applicators or balloon catheters of varying diameters from 1 to 5 cm; and intraoperative electron beam treatments from linear accelerators delivered through cylindrical applicator tubes varying in diameter from 3 to 10 cm and ranging in energy from 4 to 12 MeV. Electron IORT is often designated as IOERT to distinguish it from 50 kV IORT approaches. Both 50 kV IORT and IOERT have been used to boost the tumor bed during surgery and also for single-fraction APBI treatment. One 50 kV device that uses a double-lumen balloon applicator has also been used to replace the Ir192 source used in balloon APBI, delivering the radiation treatment postsurgically in ten fractions over 1 week.
It is important to understand that each IORT technique generates different energies and utilizes a different applicator system, thus is associated with very different radiation target volumes and dose distributions. It is therefore not possible to extrapolate the results from one IORT technique to another. Required surgical techniques for performing a lumpectomy differ among surgeons with several technical restrictions, such as margin positivity, size of the tumor, and receptor analysis. It therefore may not even be appropriate to extrapolate the results from the same IORT technique and assume it still applies if the surgical approach and restrictions differ widely.
HDR-IORT [40]
Applicator:
HDR-IORT uses a modified quadrangular Silastic breast applicator (HAM applicator, Mick Radio-Nuclear Instruments, Mt, Vernon, NY). The HAM applicator for breast IORT is a 2 cm thick, rectangular block of tissue-equivalent material with catheters running the length of the block through its center, spaced 1 cm apart. The catheters accept Ir192 sources from an HDR afterloader (Fig. 28.1b). The width of the HDR applicator and the number of catheters can be varied depending on tumor size. The length of the HDR applicator block varies from 4 to 6 cm, which is sufficient to provide radiation down to the level of the muscular fascia.
Fig. 28.1
HDR-IORT Technique: (a) Skin retraction; (b) use of blue plastic simulation applicator to obtain best applicator fit; (c) HAM applicator placed in the surgical cavity reaching the pectoralis major muscle. Note that the breast parenchyma is flush with the applicator and that a tungsten shield protects the skin; (d) cross-sectional display of dose distribution, optimized by computerization of dwell times of the Ir192 source in each of the catheters (Photos courtesy of G. Cohen, Memorial Sloan Kettering Cancer Center, New York City)
Surgical Approach:
The radiation from this approach has very limited penetration beyond the lumpectomy cavity, so it is especially important to achieve negative margins upon final pathologic analysis. The tumor is excised with the objective of achieving a grossly free margin of at least 15 mm. The surgical specimen is examined radiographically and then sent to the pathologist for further gross margin analysis. Immediate re-excision is then performed for a margin that appears positive or even close. All patients receive a sentinel lymph node biopsy (SNLB). The breast parenchyma must be detached from the skin and the skin edges retracted to avoid excessive radiation exposure (Fig. 28.1a). After the tumor is removed, the applicator is inserted into the excision cavity.
Radiation Treatment:
The catheters are attached to a remote afterloader. An Ir192 source is sequentially inserted into each catheter of the applicator and moved over its length under computer guidance. The computer then determines the dwell time of the radioactive source at each point along the catheter in an attempt to achieve a homogeneous distribution of radiation. The resultant radiation distribution is in the form of an elliptic cylinder, with the maximum dose delivered at the surface of the cylinder. The dose rapidly decreases as the distance from the applicator surface increases. Figure 28.1c shows the special HDR applicator and its placement in the lumpectomy cavity. It is important that the breast tissue to be irradiated is in direct contact with the applicator. This is typically not an issue, since any space between the applicator and the surrounding breast parenchyma is filled with fluid. A single fraction of 20 Gy was initially prescribed 1 cm from the surface of the applicator; however, after the first 18 patients had significant acute toxicity, the dose prescription was reduced to 18 Gy to the lateral margin of the surgical cavity.
Other Factors:
A shielded operating room (OR) is required for the treatment. The placement of the applicator and the treatment planning takes about 10 min, and irradiation times are approximately 40 min, for a total additional operative time of about 1 h. All personnel must leave the OR during the treatment, so provisions for remote anesthesia monitoring and patient observation are required. Subsequent to the initial published trial, the HAM breast applicator was modified to have a curved surface on the short side of the applicator to promote better conformation of the breast tissue to the applicator. There is also now a tungsten shield at the top of the applicator to provide greater protection to the skin.
50 kV IORT
There are currently two 50 kV systems being used for IORT breast cancer treatment: Intrabeam (Carl Zeiss, Saarbrucken Germany) (Fig. 28.2) and Xoft (iCad, Burlington, MA) (Fig. 28.3). Both systems use miniature X-ray generators that attach to X-ray tubes with diameters of approximately 3.2 mm (Zeiss) or 2.5 mm (iCad). The target of these X-ray tubes, located at the distal end of the tube, generates low-energy X-rays of approximately 25 kV energy. The X-ray tubes are inserted into applicators that can be sterilized and then used for treatment in the OR. Prior to the introduction of these devices, there was little clinical experience with X-rays of such low energy. ASTRO has issued an Emerging Technology Committee Report on “electronic brachytherapy” devices [41] and the American Association of Physicists in Medicine (AAPM) established Task Group 146 to provide a standard for calibration and quality assurance of these low-energy devices. The AAPM was not charged with addressing the radiobiological equivalence (RBE) issues associated with these low-energy devices. This is an important, unresolved issue in the clinical use of these devices, since the effectiveness of this low-energy radiation changes as a function of applicator size and distance from the applicator surface, resulting in different equivalent biological doses delivered at a 1 cm distance from the applicator. Clinically, this means that because the same dose is applied to the applicator surface, patients with smaller tumors might have different clinical outcomes than those with slightly larger tumors, since they are treated with different-sized applicators and thus receive different biological doses.



Fig. 28.2
Intrabeam System (Carl Zeiss, Saarbrucken, Germany): (a) Intrabeam System; (b) X-ray generator with applicator inserted over X-ray tube; (c) applicator in excision cavity and being purse-string sutured into place

Fig. 28.3
Xoft Axxent System: (a) Xoft Controller; (b) balloon catheter insertion; (c) IORT delivery. Note the operator is standing behind a shielding screen (Photos courtesy of Xoft, Inc., an iCad company, Burlington, Massachusetts, USA)
Applicator – Intrabeam: The Intrabeam applicator consists of different-sized plastic spheres ranging in diameters from 1.5 up to 5 cm, with 5 mm increments in size that fit over a rigid X-ray tube.
Applicator – Xoft: The Xoft applicator is the double-lumen balloon used with the Mammosite system. Four balloons are currently available, depending on the tumor size and shape: 3–4 cm, 4–5 cm, 5–6 cm, and a 5 × 7 cm elliptical balloon. The Xoft X-ray tube is on a flexible cable that can be inserted into the catheter of the double-lumen balloon.
Surgical Approach – Intrabeam: [42–46]. The tumor is excised with an attempt to achieve negative margins of at least 10 mm. Radiographic examination of the tumor specimen and additional excision is recommended until the margins achieve this clearance. It is important to achieve complete hemostasis since the radiation treatment time can range from 25 to 50 min, and even a small amount of bleeding can cause inaccurate dose delivery. After tumor removal, the various applicator sizes are positioned within the lumpectomy cavity until there appears to be a snug fit of surrounding tissue around the applicator. The breast parenchyma is sutured with a purse string to hold the breast tissues snugly against the applicator. It is important to insure that the skin is not brought closer than 1 cm from the applicator surface. This can be achieved by undermining the skin edges and retracting the skin away from the applicator. When this is not possible, radiopaque, tungsten-filled polyurethane protector sheets can be inserted under the skin surface. Alternatively, saline-soaked surgical gauze, 5–9 mm thick, can be inserted deep to the skin to lift the dermis off the applicator in order to protect the surrounding skin. The radiopaque tungsten-filled polyurethane protector sheets can also be fitted like a cap on the applicator to protect the chest wall, sometimes necessary to reduce the dose to the thoracic structures by 95 %. It is also possible to deliver the IORT radiation during a second surgical procedure, several weeks after the original operation once the final pathology is available.
The surgical approach does not differ markedly from that used in postoperative balloon catheter placement, except that with IORT, the balloon is implanted during the lumpectomy procedure, the radiation is delivered, and then the balloon is removed before closure. All patients undergo SLNB for node assessment, which must be negative for IORT to be administered. Margins also need to be negative, though no special assessments during surgery have been reported, other than selecting low-risk women, using preoperative MRI to eliminate multicentric or multifocal disease, and careful attention to procedure. (Note: If final pathology reveals positive margins, the patients are re-excised. If the re-excision is clear, no further treatment is given. If the re-excision is still positive, the patient is referred for WBI.)
The minimum skin spacing must be at least 1 cm. If the skin spacing is between 7 mm and 1 cm, the patient is not a candidate for IORT but can still receive 5 days of postoperative APBI. If the skin spacing is less than 7 mm, the patient is not a candidate for either technique. The breast tissue is dissected to the level of the superficial pectoralis fascia so that a flexible lead shield can be inserted to protect the chest wall. A cavity evaluation device (CED) is inserted into the lumpectomy cavity through a small incision made within the lateral aspect of the breast and filled with saline solution until the correct size balloon for treatment is determined. The CED is replaced with the appropriately sized balloon for the procedure. Temporary retention sutures are used to hold the balloon into place and to build up subcutaneous tissue to increase the skin spacing from the balloon. Ultrasound is used to assure conformance of the surgical cavity to the balloon surface and to assess the balloon to skin distance.
Radiation Approach – Intrabeam [42–46]: A dose of 20 Gy to the surface of the sphere is prescribed, falling to 5 Gy at 1 cm from the surface of the sphere. Typical irradiation times using this approach are 30–50 min. The advocates of 50 kV treatments maintain that, despite the sharp falloff in dose, there is an increase in RBE as a function of distance from the sphere as the energy decreases. Even if one were to accept this concept, the equivalent dose at 1 cm could at most be only 18 Gy, with the dose at 2 cm only 6.3 Gy [49]. The rapid falloff in dose, even with an increase in RBE, may not adequately irradiate the microscopic disease that is known to extend at least 2 cm in some cases beyond the tumor proper. If the IORT is delivered in a second procedure, the applicator is reinserted into the excision site. However, the diameter of the applicator used in a second procedure is considerably smaller than if the IORT was given at the time of the tumor excision, e.g., 2.5 cm vs. 4.5 cm [42]. The reduction in applicator size and the delay in delivery will irradiate a different volume to a different dose and at a different time with respect to the microscopic tumor environment, than if the IORT was delivered during the initial surgery. This difference in timing of IORT delivery apparently impacts clinical outcomes for APBI [50, 51], but has not yet shown to affect the outcomes for IORT kV boost.
Radiation Treatment – Xoft [47, 48]: While the surgeon is inserting and checking the placement of the balloon, the physicist calibrates the Xoft X-ray source using a modified well chamber, similar to those used in the calibration of brachytherapy sources. During calibration, the X-rays are turned on for about 15 s and the entire calibration process takes less than 15 min. A flexible lead-equivalent shield is draped over the breast to protect the patient, with the anesthesiologist, physicist, and radiation oncologist standing behind protective shields. The rest of the OR staff leaves the room for the radiation delivery, which lasts 22 min and varies only slightly with balloon diameter (20–24 min). A dose of 20 Gy is delivered to the surface of the balloon falling to about 5 Gy at 1 cm. Due to the design of the Xoft balloon applicators, it can also be used to provide postoperatively APBI in ten fractions over 5 days.
Other Factors: Before each day’s treatment, the physicist must calibrate and conduct quality assurance (QA) testing on the Intrabeam system to assure proper performance. It takes about 2 h to perform calibration and QA [46] using the Intrabeam supplied equipment calibration and QA equipment. With the Xoft system, calibration is performed concurrently with the surgery and takes less than 15 min. During the radiation procedure, for both systems, everyone leaves the OR, except the radiation oncologist, physicist, and anesthesiologist who stay behind protective shields. It is recommended that patients receive prophylactic antibiotics to reduce the risk of infection. Both systems recommend that the patient be draped with protective radiation shields. Utilization of the Intrabeam adds about one additional hour to the surgical procedure, excluding the 2 h of physics calibration. Total time for a Xoft IORT procedure, including the lumpectomy, SLNB, balloon placement, radiation treatment and closure, is about 2 h.
IOERT [52–56]
There are currently two types of electron generators that can deliver IOERT: conventional radiotherapy accelerators operating in the electron mode and mobile IOERT units, Mobetron® (Intraop Medical, Sunnyvale, California USA), Liac (Sordina, Padova, Italy), and Novac (NRT, Aprilla, Italy). Conventional accelerators have higher electron energy ranges (4–20 MeV) compared to the mobile units (3–12 MeV), but for breast IOERT, 12 MeV is sufficient penetration. A conventional accelerator weighs 8–10 tons and requires substantial radiation shielding of 100 tons or more in order to limit stray radiation to the surrounding areas. The mobile units can be used in unshielded ORs, though some require about 1 ton of mobile shielding be positioned around the surgical bed before treatment to protect surrounding areas from excessive stray radiation. Figure 28.4 shows the IOERT units currently available.


Fig. 28.4
Mobile IOERT linacs: (a) Novac 7; (b) Liac; (c) Mobetron; and (d) conventional linac being used for IOERT. Conventional linacs used in IOERT require shielded bunker. Mobile linacs can be used in ORs with little or no additional shielding. Novac and Liac usually require about 1 ton of mobile shielding to be placed around and under surgical bed for radiation protection of surrounding areas
Applicators: All IOERT units use cylindrical applicators for breast treatment. Both acrylic applicators and metallic applicators are in use. Metallic applicators can be steam sterilized quickly using an autoclave, while acrylic applicators need to be gas sterilized or sterilized with a liquid process, both of which take a day or more to complete. The sterilization process can be an issue if multiple IOERT cases are planned for the same day or for consecutive days. Acrylic applicators have diameters that range from 3 to 10 cm in 1 cm increments, with a wall thickness of 5 mm. Metallic applicators have the same range of diameters, but with 5 mm increments and 2 mm wall thickness. IOERT applicators must be in direct contact with the breast parenchyma surface before irradiation. Acrylic applicators used with the Liac and Novac are 60–100 cm in length and are directly connected to the head of the accelerator for treatment. The metallic applicators used with the Mobetron are held in place by a special clamp that attaches to the rails of the surgical bed.
Conventional accelerators use both acrylic and metallic applicators of varying lengths, depending on the attachment mechanism they employ. The Mobetron also provides 5 and 10 mm acrylic bolus disks that can be used either to increase the surface dose or to provide protection to deep-seated tissues. To facilitate contact for tumors located over the curvature of the CW, applicators come with bevel angles of 0°, 15°, 30°, and 45°. The smaller the bevel angle, the more uniform the radiation is across the applicator diameter. It is inadvisable to use 45° bevels, as the homogeneity across the field is inadequate to provide for sufficient dose coverage of the tissue at risk, especially at the applicator perimeter. Typical energy and field size use for a mobile IOERT unit used in breast cancer treatment is shown in Fig. 28.5.
Fig. 28.5
Typical energy (a) and field size (b) use for breast IOERT. Median field size used was 5.5 cm; bolus was used in 40 % of patients to either increase the surface dose or reduce the depth of penetration; beveled applicators were used in 45 % of patients, and ½ cm rather than integral field size applicators were used in 40 % of patients (Data from March 2011 customer survey involving 2,200 breast IOERT boost and IOERT APBI patients, courtesy of Intraop Medical Corporation, Sunnyvale, California)
Surgical Approach: The surgical approach is identical, irrespective of which electron generator is used, and is similar whether boost or APBI is planned, with the exception that the chest wall (CW) protector is always used in IOERT APBI and is not usually employed for IOERT boost. The tumor is removed as usual with an attempt to achieve free margins. If oncoplasty is planned for the patient, an oncoplastic approach suitable for the breast in question is used to remove the tumor. There is no consistently reported method of margin clearance, with some centers relying only on good technique and wide excision surgery and others on the wider surgery of quandrantectomy. In some centers, intraoperative frozen section is performed of the margins in order to assess margin clearance. After the tumor is excised, in IOERT boost, the breast parenchyma is loosely approximated so that the sides of the excision cavity can be temporarily sutured to form a flat surface for the IOERT radiation. In IOERT APBI, the breast parenchyma is also lifted off the CW and is remodeled. The remodeling of the breast parenchyma, together with the temporary re-approximation, morphologically transforms the breast tissue at risk, irrespective of the tumor shape, so that it is compactly positioned directly beneath the electron applicator (Fig. 28.6). This surgical approach positions all of the tumor margins at the center of the radiation field and irradiates several centimeters of tissue from the center in all directions. The amount of peripheral circumferential extension of the radiation field depends upon the size of the applicator chosen. The clinical treatment volume (CTV) irradiated with IOERT is comparable to that used in 3D conformal APBI, but can be slightly smaller while covering the same tissue at risk, since no additional tissue volume needs to be included to allow for patient motion and errors in patient setup. Furthermore, because more normal tissue can be excluded from the radiation field with IOERT, the dose-volume histograms (a measure of homogeneity of tumor coverage and normal tissue exposure) with IOERT APBI appears to be more precise compared to those generated with any of the EBRT APBI techniques.
Fig. 28.6
Volumes treated and dose distributions resulting from the different IORT approaches. (a) HDR-IORT: The volume irradiated is an elliptic cylindrical shell extending down to the CW, with 18 Gy delivered at the inside surface of the cylinder, dropping to about 4 Gy at 1 cm from the inside surface. (b) Intrabeam: The volume irradiated is a spherical shell, with 20 Gy at the applicator surface (inside shell) dropping to 5 Gy at 1 cm. (c) IOERT: The surgical approach morphologically transforms the breast tissue, irrespective of the tumor shape, so that several centimeters of tissue originally adjacent to the tumor is compactly positioned under the applicator (see inset). Radiation is uniform across the applicator and extends to the CW. (d) Dose-volume histograms from [57] showing uniformity of tumor volume coverage of the various approaches used in IORT
For IOERT APBI, a shielding disk must be inserted between the remodeled breast parenchyma and the CW (Fig. 28.7c). Shielding disks are usually comprised of a lead and aluminum sandwich of 6–9 mm in thickness, though disks with other shielding materials are in use, such as acrylic disks with and without metal inserts. It is important to select a CW shield with a diameter at least 1 cm circumferentially larger than the applicator for the treatment. Validation of the correct placement of the shielding disk by U/S or a physical probe should be made prior to radiation delivery. For IOERT boost, a CW shield is generally not required, providing the dose to the rib from the IOERT boost will be <5 Gy. If acrylic applicators are used, the skin must be retracted, so it is not in contact with the applicator (Fig. 28.7a). This is not necessary with metallic applicators, but provision must be made so that the skin cannot fall into the radiation field. This is usually accomplished by tying sutures from the skin to the clamping system holding the metallic applicator in place (Fig. 28.7b). After the IOERT treatment, the temporary sutures and the protective CW plate, if used, are removed. The breast parenchyma is remodeled, and closure is performed in the usual fashion. If an oncoplasty was planned, the breast remodeling needed for the IOERT will have already accomplished a large portion of that procedure.
Fig. 28.7
IOERT Technical Aspects-Part I: (a) skin retraction needed with acrylic applicators; (b) suturing skin to applicator clamp to prevent from falling into radiation field with metal applicators; (c) visualizing and imaging the treatment field to assure proper coverage of boost or APBI; (d) inserting shielding disk to protect CW
If the final pathology reveals positive margins, IOERT boost patients are re-excised and receive EBRT after their breast has healed (typically 3–4 weeks). If the nodes are positive, EBRT is delayed so that the patient can receive chemotherapy first, if necessary. For IOERT APBI patients with positive margins on final pathology, there is no standard consensus for margin treatment. Some centers do nothing, reasoning that because of the re-approximation technique, all the margins in IOERT APBI patients have already received a minimum dose of at least 18 Gy (i.e., surface dose for 21 Gy) for APBI and that margin positivity is less of a factor. This may be especially true in the older, low-risk group of women being treated with IOERT APBI [58]. Other centers re-excise and give no further radiation treatment.
Radiation Treatment: The radiation oncologist selects the applicator that is needed to cover the tissue at risk and positions and centers it on the re-approximated tumor bed. The applicator should be chosen with a diameter 1.0–1.5 cm larger than the maximum tumor dimension for IOERT boost and 1.5–2.0 cm larger for IOERT APBI. With IOERT boost, additional radiation is delivered through EBRT to adequately treat the microscopic disease that might extend a few cm beyond the tumor. For APBI, it is important to choose an applicator large enough to adequately treat this tissue at risk, because there is a “cold” radiation gap at the walls of the applicator (Fig. 28.6c). The 90 % radiation level – the usual prescription dose for IOERT – reaches only to within 4–5 mm of the inside wall of the applicator, depending on the design of the accelerator and applicator system. This “cold” gap is amplified when beveled applicators have to be used. Fortunately, the use of IOERT applicators that are sufficiently sized to adequately treat the tissue at risk does not appear to clinically result in increased fibrosis or poorer scar healing.
The 0° bevel applicator results in the best radiation coverage for all applicator sizes. Figure 28.8a shows a technique that allows a 0° bevel applicator to be used even when on a CW curvature, improving the homogeneity of the treatment. An acrylic bolus disk holds the remodeled breast parenchyma, and sterile gauze is wedged between the CW and the disk to present a 0° bevel angle for the IOERT. The depth from the surface to the shield plate or CW can be determined by utilizing intraoperative ultrasound or a mechanical probe (Fig. 28.8b). The depth measurement determines the energy of the treatment, with the 90 % depth having a variation from 11.0 to 35.0 mm for energies ranging from 4 to 12 MeV.
Fig. 28.8
IOERT Technical Aspects-Part II: (a) Flat surface created by wedging sterile gauze between the CW and an inserted bolus plate, allowing 0° bevel applicator to be used on sloping CW surface; (b) measuring the depth of the gland, either with a mechanical probe or with U/S; (c) soft docking; (d) hard docking
The applicator, now positioned in the patient, and the accelerator that is used to deliver the IOERT, must be aligned before treatment. For units that require physical connection of the applicator to the head of the treatment unit (“hard docking”), either the patient bed is moved under the treatment unit (e.g., in all conventional units) or the mobile accelerator is moved to the surgical bed. The head of the treatment unit is rotated and positioned so that the mechanical connection can be made. Care must be used to ensure that the applicator that is being handheld in the patient by one of the surgeons or radiation oncologists does not shift position during the attachment process (Fig. 28.8c).
In systems that do not directly connect the applicator to the treatment head (“soft docking”), alignment is achieved through the use of lasers to guide the orientation (Fig. 28.8d). Both docking systems are relatively quick, once some experience is gained. For units that use “soft docking,” it is possible to document the tumor bed site using a small handheld TV camera (Fig. 28.7d). If the surgical bed needs to be moved for docking, the anesthesia equipment must also be moved or needs hoses long enough to accommodate the move. For some mobile units (the Liac and Novac), mobile shields must be positioned around the table and under the treatment bed for radiation protection to the surrounding areas.
The physicist calculates the amount of radiation units needed to deliver the prescription dose. Some centers use in vivo dosimetry to validate and/or monitor the IOERT dose (Fig. 28.7a). The Novac and Liac generally divide the treatment into two parts and adjust the dose delivered in the second half to improve the accuracy of dose delivery. This is not necessary with conventional IOERT units or the Mobetron. For IOERT boost, the dose prescription is usually 10 Gy to the 90 % depth. For IOERT APBI, it is predominantly 21 Gy to the 90 % depth.
Everyone leaves the room during the time that the radiation is being delivered. The patient and anesthesia must be monitored by video or remotely. Treatment typically takes 1–5 min, depending on whether the treatment is a boost or APBI. Mobile IOERT units have treatment times of only 1–2 min, as the dose rate for mobile units is at least a factor of two higher than that of conventional units. Total time added to the surgical procedure for breast IOERT is 15–25 min.
Other Factors: The large dose of radiation delivered by IOERT in a single treatment makes the calibration and QA of each unit critically important to assuring proper treatment. Calibration and QA procedures for IOERT for conventional accelerator units was developed by the American Association of Physicists in Medicine (AAPM) and published in their Task Group 48 report [59]. Similar procedures for mobile IOERT units are published in Task Group 72 report [60]. The latter report recommends daily validation before treatment of both output and energy. For the Mobetron, these daily QA measurements can be made with 12 Gy exposure for all energies and outputs (about the amount of one IOERT boost treatment). With the Novac and Liac units, due to their unique method of generating the radiation, 60–100 Gy of exposure is required for output measurements.
This high level of exposure dose generated by these units for QA may require that the QA be taken when the adjacent areas are unoccupied, since the ORs for mobile units are unshielded. Alternatively, some of their users now use online in vivo dosimetry to validate output during the treatment, dividing the treatment into two halves and adjusting the remaining dose based on the output of the first [61–63]. Radiation QA is an important element for any multi-institutional study. The HIOB protocol (see Future applications) has developed QA procedures that allow all IOERT units to participate in multi-institutional studies with a high level of confidence.
The main technical difference between the IORT and IOERT approach is shown in Table 28.3. Nairz et al. [57] has evaluated the dose distributions from Intrabeam, IOERT, and brachytherapy and devised a “dose inhomogeneity index,” or “Dii,” to evaluate the mean deviation of the dose inside the PTV from the prescribed dose. A Dii of 0 indicates completely homogeneous irradiation. For IOERT, the Nairz Dii = 0.047, but rises to 0.505 for 50 kV treatments (Fig. 28.6d).
Table 28.3
IORT vs. IOERT
Method | Meets TV concept of Holland | Homogeneity of radiation distribution | Can treat asymmetric PTV | Treatment time | Added OR time | Shielded OR needed |
---|---|---|---|---|---|---|
IORT | No | Poora | Nob | 25–50 minc | ~1 h | No |
IOERT | Yes | Excellent | Yes | 1–3 mind | 15–30 min | Noe |
IORT Clinical Results: IORT as a Boost
IORT kV boost (when delivered at the time of the tumor removal) and IOERT boost have several similarities, despite very different radiation distributions and volumes irradiated by the two approaches. In both IORT approaches, radiation is given under direct visualization, eliminating the possibility of a geometric miss that is possible with an EBRT boost. Both the IORT and IOERT boosts can be used in wide variety of BCT eligible patients, delivering the dose subcutaneously, which should result in lower skin toxicity. They both eliminate approximately 1 week of EBRT boost treatment and start the radiation treatment at the time during the surgery when residual tumor cells are hypothesized to be rapidly proliferating. Because both IORT boosts are combined with several weeks of WBI to complete the BCT treatment, the differences in the volumes and dose distributions of the two approaches may be less important in IORT boost than in IORT APBI, since the WBI can partially compensate for the differences in the approaches.
Boost Clinical Results: 50 kV
Vaidya et al. [64] reports on long-term results of IORT boost with 50 kV using the Intrabeam system. Three hundred cancers (299 patients) underwent BCS and 20 Gy as a boost to the tumor bed. After wide excision of the breast cancer, the Intrabeam applicator that best fit the excision cavity was inserted into the cavity, and a purse-string suture was made to adapt the breast tissue to the applicator surface. A dose of 20 Gy to the surface of the applicator was administered, delivering 5–7 Gy of radiation 1 cm from the applicator surface. In this study, all tumors were unifocal on mammography, and none of the tumors exceeded 4 cm in diameter. There was no restriction on tumor type, tumor grade, receptor status, or axillary node involvement. The median patient age was 57 (range 28–83 years), 79 % of the tumors were 2 cm or less, and 29.9 % had Grade 3 tumors. Of the 242 patients in whom systemic therapy was analyzed, 94 patients (31 %) required adjuvant chemotherapy, and 195 patients (81 %) received adjuvant hormonal therapy. Patients with positive margins on final pathology that required re-excision either went on to receive a completion mastectomy or were excluded from the analysis. In one cohort of patients, IORT was delivered postoperatively in a second operation, after a median time of 4.9 weeks from the initial operative removal of the tumor. The additional time required for IORT setup and delivery was 30–50 min. All patients received 45–50 Gy of EBRT in 25 fractions over 5 weeks. If adjuvant chemotherapy was required, EBRT was delivered at its completion. With a median follow-up of 60.5 months (range 10–122 months), eight patients have had an ipsilateral recurrence. The 5-year Kaplan-Meier estimate for recurrence is 1.73 %, with five of eighth recurrences identified within the tumor bed, thus reducing the true local recurrence (TLR) rate at 5 years to 1.04 %.
Wenz et al. [65] reports on his own Intrabeam boost experience of 155 breast cancers in 154 women treated with Intrabeam as an anticipated boost. The median age was 63 years (range 30–83 years), 65 % of the patients had T1 tumors, and 35 % had T2 tumors. The median applicator size used was 4.5 cm (range 2.5–5 cm), resulting in treatment times of 7.5–51.1 min (median 36.6 min). A complete axillary lymph node dissection (CALND) was performed if the SNB was positive; 70 % of patients were N0, 22 % N1, and 8 % N2 or N3. EBRT was initiated after either wound healing or completion of chemotherapy (median 40 days, range 13–226 days). Adjuvant chemotherapy was given to 46 (29.9 %) patients and adjuvant hormonal therapy to 129 (83.8 %) patients. WBI using standard tangential treatment portals was delivered using a 6 MeV linear accelerator to a dose of 46 Gy in 23 fractions. The EBRT dose was increased to 50 Gy to the breast and to supra- or infraclavicular fossa in 26 patients with involved nodal areas.
With a median follow-up of 34 months (maximum 80 months), there were a total of ten deaths – eight due to distant metastases – and two ipsilateral breast tumor recurrences (IBTR), resulting in a 5-year Kaplan-Meier OS of 87 %, and a relapse-free survival rate of 98.5 %. Chronic toxicity after 3 years was mild, with two-thirds of patients experiencing none or barely palpable fibrosis. Five patients had a marked increase in breast density, with one patient with Grade 3 fibrosis requiring a mastectomy. Breast edema was seen in 8 % of patients, and skin toxicity was mild, with only 6 % experiencing telangiectasia and 6 % hyperpigmentation.
Wenz et al. [66] cautions that starting EBRT too soon after IORT could lead to increased toxicity. The toxicity analysis involved 48 patients with a median follow-up of 36 months (range 30–56 months). The median time between IORT delivery and the initiation of EBRT was 36 days. In general, the toxicity was mild, with 30/48 (63 %) experiencing either no change or only minor changes. However, a statistically significant and clinically relevant tendency for late toxicity occurred when the time interval between IORT and EBRT was too short: 8/12 higher-grade fibroses, 5/6 retractions, and 4/5 breast pains occurred in patients with an IORT-EBRT interval less than the median of 36 days. The recommended 5–6-week interval between IORT boost and initiating EBRT is 1–2 weeks longer than is needed with an IOERT boost or standard BCT treatment. While Wenz cautions that the impact of this longer gap between IORT and EBRT on recurrences is not known, it may not be significant, as longer time gaps occur with both IOERT and Intrabeam boost patients who have EBRT delayed due to adjuvant chemotherapy [56, 64, 67–70].
Most of the studies to date show that IORT boost with Intrabeam appears to provide an acceptable local control rate when compared with standard BCT. The treatment toxicity is also considered acceptable, providing that attention is paid to proper technique and patient selection when using this device. For optimal cosmetic results, maintaining proper skin spacing and avoiding the Intrabeam’s use in larger T2 tumors and small-breasted women, as well as waiting 5–6 weeks to initiate the EBRT, seem to be important factors. Long-term data, requiring further follow-up, is still needed in order to adequately assess cosmetic results. There is currently no long-term data or follow-up from the iCad Xoft system when used as a boost.
Boost Clinical Results: IOERT
The first reported use of IOERT for early-stage breast cancer was a combined study of the Medical College of Ohio and the Centre Regional de Lutte Contre le Cancer (Montpellier, France) [71]. In this study, 72 patients (Stage I – 43 %, Stage II – 57 %), between the age of 33 and 81 years, were given a 10 Gy IOERT boost following segmental mastectomy, with temporary re-approximation of the breast parenchyma to bring the margins of the cavity together. All patients underwent an axillary lymph node dissection. The field size was chosen to encompass the tumor bed with at least a 10 mm margin. After wound healing (typically 1–3 weeks), patients received an additional 45–50 Gy of radiation over 5–6 weeks with external X-ray radiation (6 MV or cobalt therapy). With follow-up of 2–17 years, only one patient developed a recurrence, and cosmesis was reported as excellent.
In 2006, Montpelier [72] updated results for the 50 patients it treated. Margins were assessed during the operation by frozen section, and all patients underwent a CALND. The median dose delivered was 10 Gy to the 90 % line (range 9–20). After IOERT, the temporary retaining sutures were removed, and the tumor cavity was remodeled. The EBRT dose was 50 Gy in 25 fractions, delivered with cobalt radiation. Nodal irradiation was given as required. For the two patients who required chemotherapy, EBRT began 3 weeks after its completion, with the others undergoing EBRT typically 4 weeks postsurgery. With a median follow-up of 9.1 years (range 5–15 years), they observed two local recurrences, one at 8 years and one at 14 years. Six additional patients had distant metastases, and 45 patients are alive, 1 with disease, giving a 10-year OS of 94 %. All patients in their series had good to excellent cosmesis, despite the fact that all had a CALND rather than SNB for nodal assessment. The high level of good cosmesis reported in this study might be a result of the skin and normal tissue sparing that occurs when using the IOERT boost as compared with the conventional EBRT external beam boost, as well as to the breast remodeling they employed at the time of surgery.
The University of Salzburg treated two consecutive series of patients with Stages I and II breast cancer [68]. Group I consisted of 188 patients treated with breast-conserving surgery and postoperative irradiation to the whole breast, followed by a postoperative external beam boost to the tumor bed. Group 2 was 190 patients treated with breast-conserving surgery, IOERT boost directly to the tumor bed, and postoperative irradiation to the whole breast. The groups were comparable in regard to age, menopausal status, tumor size, histological type, grading, and axillary lymph node status. Exclusion criteria were neoadjuvant chemotherapy, tumors > pT2, multicentricity, and ductal carcinoma in situ. For the IOERT boost patients, if margins were not clear with a minimum of 3–5 mm in the intraoperative pathologic assessment, re-excision was performed in the same surgical procedure prior to IORT. If margins were not clear in the final pathological report (minimum 3 mm), a secondary re-excision was performed before the patients had their EBRT. Margins were negative in all patients included in this study. Axillary surgery in Group 2 was confined to sentinel lymph node biopsy only, if sentinel lymph nodes were negative. Complete axillary clearance of levels I and II was performed, if sentinel lymph nodes were positive. After complete tumor resection and axillary surgery, the tissue surrounding the tumor bed was mobilized and temporarily approximated by sutures. Ultrasound was used to determine the depth dose, which ranged from 0.6 cm to 3.9 cm (median 1.9 cm). Applicators with diameters of 50–60 mm were used, and a dose of 9 Gy was delivered to the 90 % reference isodose, using the appropriate energy for the tumor thickness.
After wound healing, 51 Gy of EBRT for patients with IDC and 56.1 Gy for patients with ILC were delivered in daily fractions of 1.7 Gy to the whole breast. For the IOERT boost patients, 18.9 % received adjuvant chemotherapy, and an additional 4.7 % received combined chemotherapy and endocrine therapy. For these patients, EBRT was sequenced after completion of chemotherapy, with a time delay after IORT of up to 20 weeks. After median follow-ups of 81.0 months and 51.1 months in Group 1 and Group 2, respectively, no IBTR was observed in the IOERT boost patients, while 12 IBTRs (6.4 %) occurred in Group 1. The 5-year actuarial rates of IBTR were 4.3 and 0.0 %, respectively (p = 0.0018). Distant metastases occurred in 24 patients (12.8 %) and 8 patients (4.2 %) in Group 1 and Group 2, respectively, for 5-year actuarial rates of distant recurrence of 8.6 % and 4.2 % (p = 0.08). The 5-year disease-free survival in Group 1 was 90.9 % and was 95.8 % in Group 2 (p = 0.064). The only serious complications reported were three rib fractures that occurred early on in the IOERT group. This complication was eliminated when the IOERT dose to the ribs was limited to 5 Gy or less.
Salzburg has recently updated this “matched-pair” analysis [67] with a very mature median follow-up period of 12.6 years for the patients treated with conventional BCT (Group 1), and 10.7 years for those receiving the IOERT boost (Group 2). Figure 28.9 shows the results of this study for IBTR, True Local Recurrence (TLR), distant metastases, and DFS at 10 years. The excellent results and advantage reported at 5 years of IOERT boost when combined with WBI over conventional BCT with EBRT boost have been maintained, with an TLR of 0.5 % vs. 4.4 % (p = 0.0029) and an IBTR of 1.6 % vs. 7.2 % (p = 0.0023). IOERT also has more favorable results for distant metastases and DFS, though with the small numbers in the study, it did not achieve statistical significance. It will be interesting to follow this study further to see if, as Clarke pointed out in his meta-analysis [11], the improved local control at 5 years translates into a survival advantage at 15 years.


Fig. 28.9
Long-term results of the University of Salzburg matched-pair analysis comparing IOERT boost (median FU 10.7 years) with EBRT boost (median FU 12.6 years). IOERT boost has a 10-year true local recurrence (TLR) of only 0.6 %, and an IBTR of 1.6 %, both significantly better than the EBRT boost. IOERT also results in fewer distant metastases, though at the time of the analysis the difference was not statistically significant
A pooled analysis [69, 70] from seven European centers combined and analyzed patients who were treated using IOERT boost plus 5 weeks of postoperative EBRT. There were 1,109 patients treated between October 1998 and October 2005, 52 % of whom had one or more risk factors for recurrence: young age (<40 years), positive nodes, high grade of tumor (G3), or larger tumors (T3). The patients in the study were all treated similarly to those in the Salzburg approach. With a median follow-up of 72.4 months, there were just 16 IBTR (1.44 %) and only 8 TLR (0.8 %). DFS, DSS, and OS were 88.6, 94.0, and 91.3 %, respectively. Young age is clearly a risk factor (Table 28.4), but the 5-year recurrence rate of 3.8 % for the 53 women under the age of 40 still compares very favorably with historical rates of 10 % for this age group. In fact, IOERT boost results in lower recurrence rates in every age group, compared to historic controls (Fig. 28.10, Table 28.5).

Table 28.4
Risk factors for IOERT
Factor | IOERT boost | IOERT APBI |
---|---|---|
Age | <40 years | <50 years |
Tumor size | Any BCS tumor OK | >2.0 cm |
Time between surgery and EBRT | >140 days | NA. No EBRT is given |
Positive margins on final pathology | Re-excise | Re-excise, ignore |
Tumor grade | G3 | G3 |
Hormonal status | Any OK | Negative |
Dose to ribs | ≤5 Gy | NA. Shield plate protects ribs |
Histology | No EIC | IDC and other low-risk histologies |
Multicentric | No, if close together to allow BCS | Yes |
Molecular subtype | Any OK | Non-Luminal A |

Table 28.5
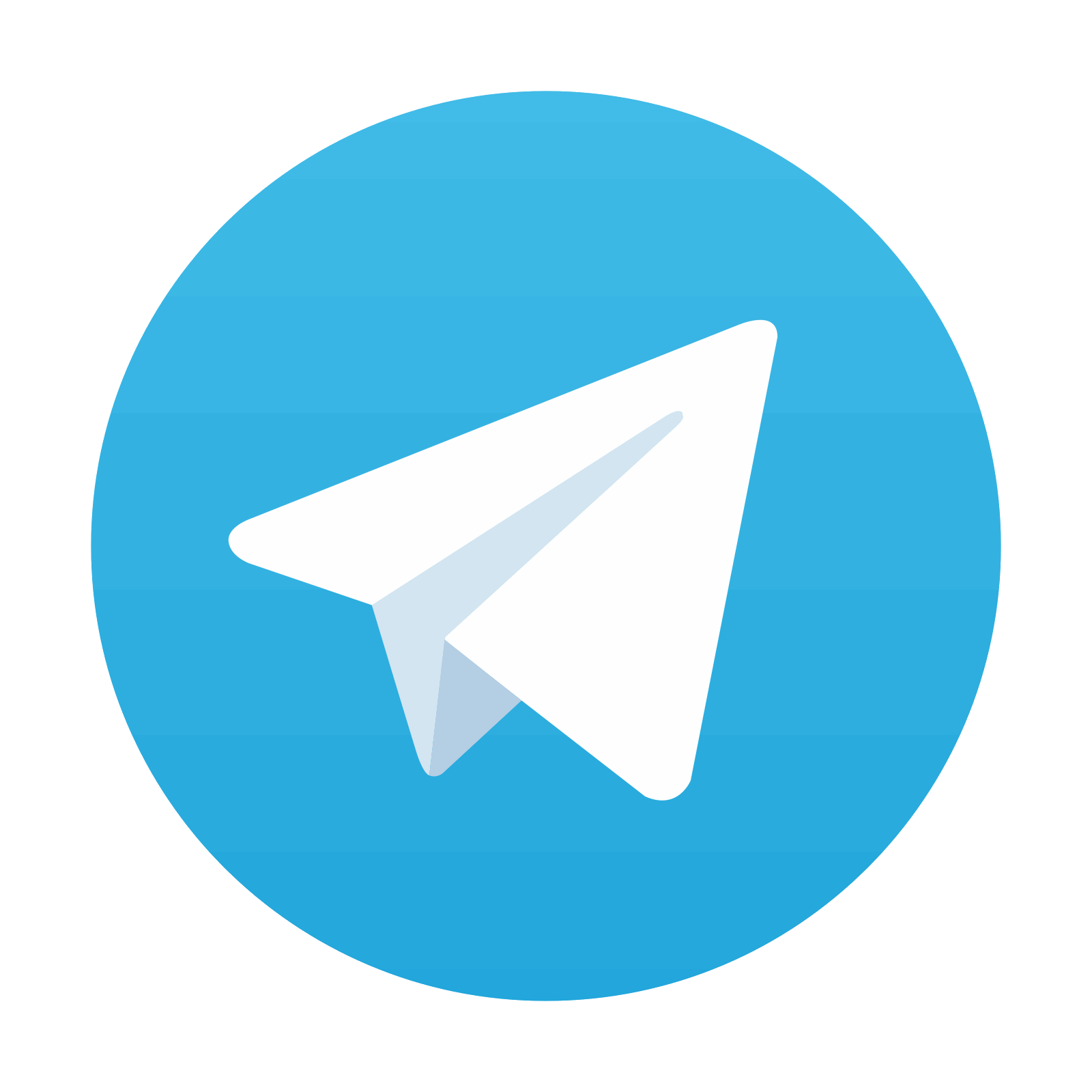
Local recurrences by age of patient after IOERT boost
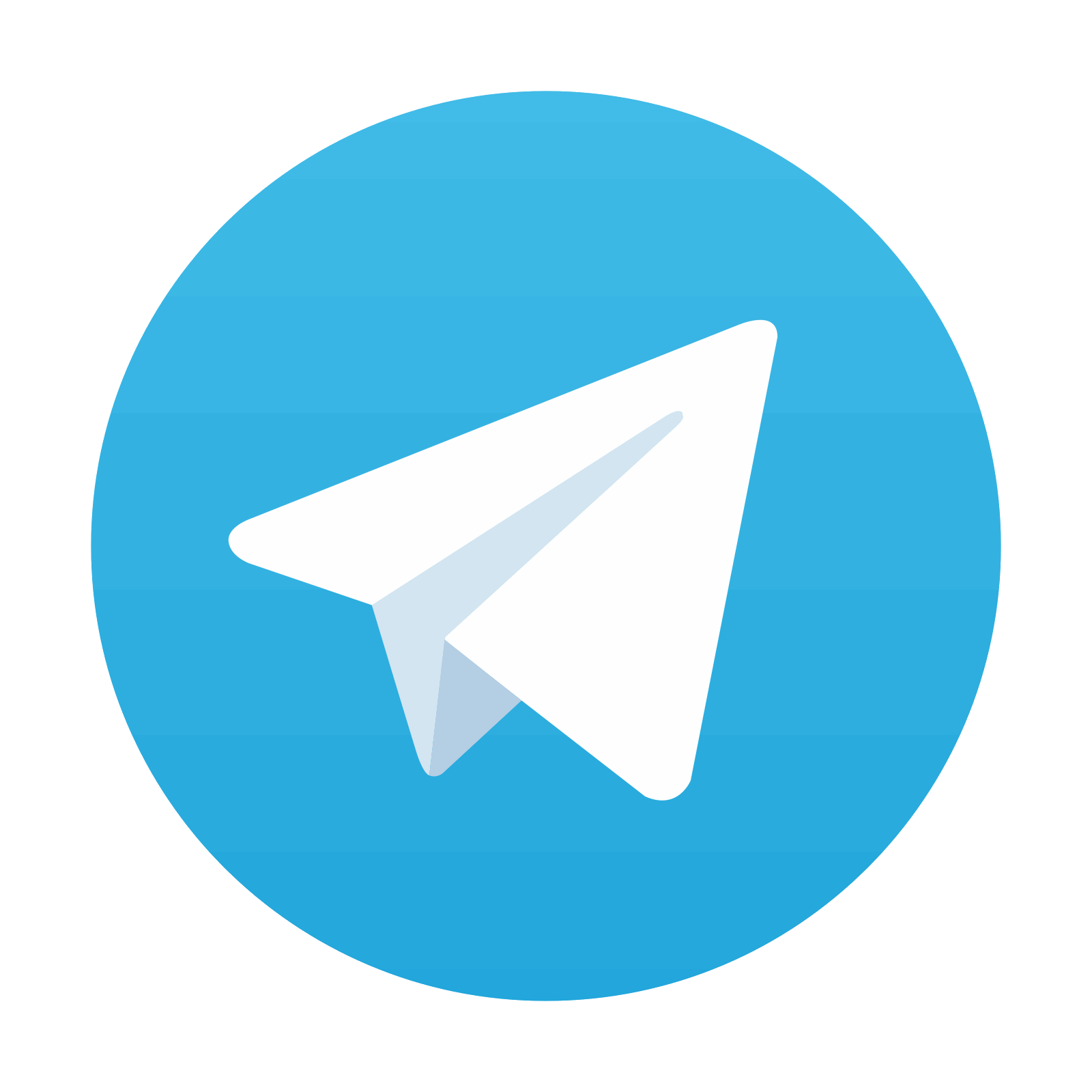
Stay updated, free articles. Join our Telegram channel

Full access? Get Clinical Tree
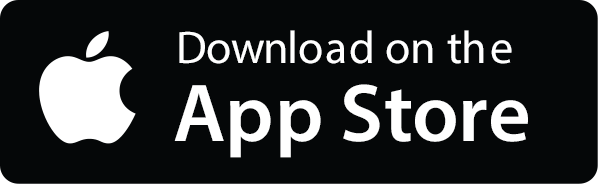
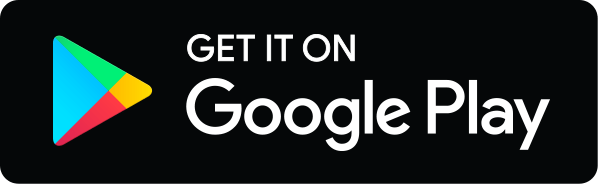
