Techniques for Assessing Blood Disorders: Flow Cytometry, Multiplex Immunohistochemistry, Karyotyping, FISH, and Molecular Approaches
Scott A. Ely, MD, MPH
New techniques, platforms, and companies spring into view on a regular basis, at a seemingly exponential rate. The challenge for health care providers is to see through biotechnology industry hype and focus on clinical utility. When implementing technology in the laboratory, feasibility and cost are critical considerations. Some techniques are very powerful for research, but, for practical reasons, cannot be adapted for diagnostic use in real-life patients in a clinical setting. Over time, some techniques fall out of use altogether as new techniques are brought on board. More often, a valuable, feasible diagnostic technique will come into use, but for limited purposes and will come to exist alongside older techniques. Truly disruptive, one-size-fits-all, new techniques are rare.
A key, critical consideration affecting the use of various techniques is cost. In the United States, for a long time, laboratory testing has been billed on a fee for service basis, with little incentive for judicious use. However, bundled payment plans currently account for one-third of laboratory testing, and that fraction is growing at a steady pace. As such, a more judicious approach to testing is coming to the fore, with less redundancy and a greater demand for true clinical utility.
This chapter focuses primarily on current modalities recognized by and incorporated into the 2016 WHO classification of tumors of hematopoietic and lymphoid tissues.1 The mainstay techniques are multiparameter flow cytometry (MFC), and immunohistochemistry (IHC), described in relevant chapters for each type of neoplasm (see Chapters 6, 7, 8, 9 and 10). Because multiplex IHC (mIHC) use is increasing and especially relevant, it is described in detail in this chapter. In addition, we have included short descriptions of newer techniques not currently in widespread use, but that hold great promise for clinical diagnostics. We have not included techniques that appear to hold little promise for widespread use outside of research in the near future. For example, although next-generation sequencing (NGS) for whole genome analysis has been touted as the technique of the future for several years and has become relatively cheaper, it remains prohibitively expensive and is not reimbursed by most payers. Moreover, technical analysis of the vast data generated by whole genome sequencing renders the technique of great value in the research setting, but of little or no practical use in the diagnostic setting. The typical pipeline for development of new tests has become discovery by broad genome analysis, then development of a directed test by a more feasible, cost-effective method, such as immunophenotyping, fluorescence in situ hybridization (FISH), or polymerase chain reaction (PCR) analysis of a single gene.
It is important to emphasize that there is no single, dominant, one-size-fits-all technology for diagnosis. For myelodysplasia, morphology remains the gold standard. For myeloproliferative neoplasms, a combination of clinical data and PCR is standard. For chronic lymphocytic leukemia (CLL), flow cytometry is the gold standard and typically, no other testing is required. For myeloma, CD138+ magnetic bead, cell-selected FISH and mIHC are most sensitive. For each modality, its use and limitations in the diagnosis of various types of neoplasms is emphasized.
MULTIPARAMETER FLOW CYTOMETRY
MFC is the technique of assessing protein expression in individual cells in a liquid medium. This involves incubating cells with fluorescent-labeled antibodies that bind to specific proteins and allow detection of protein expression by a flow cytometer. Current clinical use of flow cytometry in hematology requires live cells. Because the antibodies will stick to
dead cells and red blood cells in a nonspecific manner due to electrostatic forces, rather than true antigen binding, careful processing is necessary to yield a clean population of viable white cells. The viable cell population is then divided into multiple tubes and a different set of antibodies is added to each tube. Following incubation, the cells from each tube are drawn into the cytometer and analyzed, one by one, for size, cytoplasmic granularity, and degree of expression of the proteins corresponding to the antibodies that were added to an individual tube.
dead cells and red blood cells in a nonspecific manner due to electrostatic forces, rather than true antigen binding, careful processing is necessary to yield a clean population of viable white cells. The viable cell population is then divided into multiple tubes and a different set of antibodies is added to each tube. Following incubation, the cells from each tube are drawn into the cytometer and analyzed, one by one, for size, cytoplasmic granularity, and degree of expression of the proteins corresponding to the antibodies that were added to an individual tube.
The data generated by this analysis can then be analyzed in any of a number of ways. Aside from the numeric assessment of lymphoid subsets, performed mainly to monitor acquired immune deficiency syndrome (AIDS) patients, there are no United States FDA-approved uses for flow cytometry. As such, each diagnostic laboratory is free to analyze samples however they see fit. The instrumentation and specimen preparation have become fairly uniform, but interpretation of data varies vastly, from one laboratory to the next. In Europe, the EuroFlow consortium has been successful in standardizing the approach across most west European countries, but EuroFlow has not yet been widely adopted elsewhere.2 So, unlike some other techniques, for which the methods and interpretation are fairly uniform, it is possible for an experienced user to be very comfortable interpreting flow data from his/her own institution, but be completely lost when trying to interpret data from an institution right across the street.
Nonpractitioners are often under the impression that interpretation of flow cytometry is objective or even binary.3 It is not. Interpretation is based on “pattern recognition.” The patterns are not evaluated by computer algorithms. They are evaluated by human eyes, just like microscopy. Interobserver variability is inherently great. There is no uniformity in interpretation of what is “positive” versus “negative.” Some centers use 10% as a cutoff, others use 30%, and still others report out without formal cutoff points. Similarly, there is no uniformity in the use of intensity reporting. What one person calls “dim positive,” another will call “negative,” or “partial.” Currently, there are some concerted efforts underway to standardize MFC assessment.2 In the meantime, it is incumbent on caregivers to attempt to understand MFC methodology in their own institution in order to know how results reported for their patients relate or fail to relate to published data.
The main strength of flow cytometry is the ability to isolate cell populations of interest and then assess expression of multiple proteins within each population. Increasing the number of colors in MFC is of practical use because it decreases the number of tubes, amount of reagents, cost, and technologist work time. However, going beyond the number of colors actually used to triangulate, there is no inherent added diagnostic value in increasing the number of colors. Because gating (selecting the cells of interest) is based on only two colors (e.g., for myeloma, bright coexpression of CD38 and CD138),4 and standard visualization of the data is two-dimensional (x and y axes), there is no inherent value to the use of more than four colors (two colors to gate, another two colors to assess other proteins; Fig. 2.1). So, from a real-life, clinical diagnostic perspective, 10 colors are no better than eight, and eight colors are no better than six. The use of more than eight color analysis presents technical difficulties and is rare for clinical purposes.
The Flow Cytometer
The flow cytometer is a relatively complex device consisting of four major components (Fig. 2.2). The fluidics system begins with pumps and tubing used to introduce the specimen into the cytometer at a controlled rate. There, the specimen stream is surrounded by a pressurized stream of buffered saline known as sheath fluid, such that the cells assume a roughly single-file position due to the phenomenon of hydrodynamic focusing, which produces laminar flow. The focused stream is then propelled to the flow chamber, where the cells are illuminated by light from lasers. Ultimately, the focused stream is directed to a waste receptacle. The optics system includes: (1) the laser light source used to excite the fluorescence dyes conjugated to the antibodies; (2) the system for conveying the emitted light from the flow chamber to specific detectors; and (3) the specific detectors themselves, which are typically photomultiplier tubes (PMTs) that convert the detected photons to electrical impulses whose magnitude is proportional to the amount of light. In most flow cytometers, the emitted light is conveyed to the PMTs via a combination of dichroic mirrors (which allow light of defined wavelengths to pass, while reflecting light of other wavelengths) and optical filters (which further narrow the wavelengths reaching a PMT, and include short pass, long pass, and bandpass filters).
The electronics system measures the electrical impulses generated by the PMTs and converts these analog measurements to digital information that is gathered and interpreted by the analysis software.
The associated computer system typically consists of a personal computer that directly interfaces with the flow cytometer and controls the functions of the flow cytometer, including the acquisition and analysis of the flow cytometric data. All four of these flow cytometer components have seen major advances in recent years. Fluidics systems are managed electronically. Optical systems have progressed from using single, relatively inefficient lasers and in-air transmission of emitted light from one or two fluorochromes, to utilizing three or more, highly efficient lasers and, in some instruments, fiberoptic systems for transmitting emitted light to the PMTs. Finally, flow cytometry has benefited from the revolution in computer technology, in terms of both hardware and software for data acquisition and analysis. The net result of these technical advances has been steady progression in the number of antigens that can be measured simultaneously by flow cytometry and the quality of the data generated.
Clinical Indications for Flow Cytometry
Four major indications have evolved for the use of flow cytometry in the clinical laboratory for hematopoietic neoplasms. First, flow cytometry has been critical to the diagnosis and classification of hematopoietic neoplasms, especially lymphomas and acute leukemias. Second, with improved understanding of the antigen expression profiles associated with prognostic subgroups in certain diseases (e.g., CD28 and CD117 in myeloma5), flow cytometry has some, limited use to identify antigens with prognostic significance in hematopoietic neoplasms. Third, with the advent of therapies targeted at specific antigens in hematopoietic neoplasms (e.g., anti-CD20 therapy in B-cell non-Hodgkin lymphomas [B-NHL]), flow cytometry can identify potential therapeutic targets, although assessment of targets is typically performed by IHC because it is typically requested ex post facto, when there are no more viable cells for flow cytometry. Fourth, flow cytometry has been extensively used to monitor response to therapy by looking for minimal residual disease (MRD).3 MRD assessment has become a standard to guide therapy for potentially curable diseases, like acute leukemias. Although MRD assessment is performed for other diseases, like CLL, the clinical utility is questionable in incurable diseases nearly certain to relapse regardless of MRD.6 MRD, defined as disease undetectable by microscopic examination, typically represents less than 1% involvement by neoplastic cells. However, if more than 500,000 cells can be evaluated, the detection limit for MRD may be as low as 0.01%.
Specimen Requirements for Flow Cytometry
Although flow cytometric evaluation of DNA content can be performed on isolated nuclei from fixed tissue, flow cytometric immunophenotyping requires fresh, unfixed cells to permit evaluation of surface antigens. In the clinical flow cytometry laboratory, most specimens are peripheral blood (PB), bone marrow, or finely minced lymphoid tissue (e.g., from a lymph node biopsy or splenectomy). However, body fluids, including paucicellular cerebrospinal fluid (CSF) specimens, can be evaluated by flow cytometry. Although it is technically possible to analyze disaggregated nonhematopoietic solid tumors such as carcinomas and sarcomas, analysis of solid tissues by flow cytometry is rare, even in the research setting. Regardless of the starting tissue type, it is critical that the cells be maintained in an environment that maximizes viability prior to analysis. For tissues and paucicellular fluids such as CSF, immediate immersion into an ample volume of tissue culture medium, such as RPMI supplemented with 10% fetal bovine serum, 1% glutamine, and 1% penicillin-streptomycin antibiotics, is a common method for enhancing and preserving cell viability and preventing bacterial overgrowth. If the specimen is likely to reach the flow cytometry laboratory in 24 hours or less, the cells may be maintained at room temperature. For tissue samples, if delivery to the flow cytometry laboratory is likely to take more than 24 hours, it is reasonable to place the sample container on wet ice prior to shipment, particularly during the summer months. Although cell suspensions should never be subjected to extremes of temperature (below 0°C or above 37°C), many laboratories analyze specimens up to 24 hours old, transported on dry ice. It is typical for 50% or more of neoplastic cells to die in overnight transit; if the specimen contains a large percentage of tumor cells, it may still be diagnostic, but if the percentage of tumor cells is low, or if they are labile (e.g., myeloma [MM] cells), especially when looking for small numbers of cells (e.g., MRD), false negatives are always a possibility.7,8 PB or bone marrow specimens, as well as other body fluids containing a large amount of PB contamination, must be anticoagulated prior to transport to the flow cytometry laboratory. The most common anticoagulants include ethylenediaminetetraacetic acid (EDTA) and heparin, both of which allow adequate flow cytometric evaluation, although EDTA may deplete myeloid cells more rapidly than heparin.
Flow Cytometric Analysis Specimen Processing
All clinical specimens must be handled with universal biohazard precautions. Laboratory coats and gloves should be worn by all personnel who directly manipulate specimens. Specimen processing steps that could potentially release hazardous aerosols, such as mincing lymph node or other tissues to create cell suspensions, should be performed under a hood. Sharp objects, such as scalpels for mincing tissues, must be handled with caution and disposed of properly. After specimens have been incubated with antibodies and washed, but before they are analyzed on the flow cytometer, they should be fixed in 1% paraformaldehyde that both stabilizes antigen-antibody interactions by introducing cross-links and inactivates infectious agents. The addition of bleach to the instrument’s waste container, at a final concentration of 10%, as well as daily purging of the cytometer fluidic system with a bleach solution prior to instrument shutdown further minimize biohazard risks. A zero-tolerance policy should exist for specimen mix-ups. To minimize the chance of such mix-ups, procedures must be instituted such as the use of checklists. Perhaps most useful is advanced bar code tracking and the use of electronically generated barcode labels. Separate processing of specimens minimizes the risk of cross-contamination. Morphologic evaluation of a cytospin made after processing of each specimen is a useful mechanism for ensuring that the correct specimen is being used, in addition to providing valuable information about specimen cellularity and viability.
Red cells and dead cells must be removed from the specimen to allow accurate evaluation. This was traditionally performed by Ficoll-Hypaque density centrifugation, which is still performed in many research laboratories but is rare in the clinical laboratory setting. In this technique, the specimen is mixed with density gradient media such that, after centrifugation, red cells and dead cells lie below a clearly visible intermediate layer of medium and the viable white cells of interest are located above that layer; the viable white cells are then retrieved by a vacuum pipette. However, this technique
suffers from two major shortcomings: granulocytes are not retained in the collected specimen following centrifugation and, even within the mononuclear cell component, selective cell loss may occur. Therefore, most clinical laboratories do not manipulate the leukocytes prior to adding antibodies, but simply lyse the red cells at some point during specimen processing. Bulk lysis of the red cells using either a commercially available reagent, or an ammonium chloride solution prepared in house, may be performed prior to the incubation of leukocytes with antibodies. Alternatively, antibodies may be incubated with the leukocytes in the presence of erythrocytes, and the erythrocytes lysed at the end of the preparation step (the so-called “whole blood lysis” technique), just prior to evaluation of the cell suspension in the flow cytometer. Note that erythrocyte lysis techniques, when applied to bone marrow specimens, also destroy the great majority of the nucleated erythroid precursors. Especially in specimens with a large percentage of nucleated erythroid cells, an artifactual, relative increase in the percentage of white cell subsets will result. For this reason, myeloid blast counts for diagnostic purposes are acceptable only by smear morphology (MFC blast counts are not allowed by WHO criteria).
suffers from two major shortcomings: granulocytes are not retained in the collected specimen following centrifugation and, even within the mononuclear cell component, selective cell loss may occur. Therefore, most clinical laboratories do not manipulate the leukocytes prior to adding antibodies, but simply lyse the red cells at some point during specimen processing. Bulk lysis of the red cells using either a commercially available reagent, or an ammonium chloride solution prepared in house, may be performed prior to the incubation of leukocytes with antibodies. Alternatively, antibodies may be incubated with the leukocytes in the presence of erythrocytes, and the erythrocytes lysed at the end of the preparation step (the so-called “whole blood lysis” technique), just prior to evaluation of the cell suspension in the flow cytometer. Note that erythrocyte lysis techniques, when applied to bone marrow specimens, also destroy the great majority of the nucleated erythroid precursors. Especially in specimens with a large percentage of nucleated erythroid cells, an artifactual, relative increase in the percentage of white cell subsets will result. For this reason, myeloid blast counts for diagnostic purposes are acceptable only by smear morphology (MFC blast counts are not allowed by WHO criteria).
For tissue biopsies and fine-needle aspiration (FNA) biopsies, cell suspensions should be prepared by mechanical dissociation of the tissue in nutrient medium such as RPMI, followed by removal of larger particles by filtration through mesh with 40- to 50-µm pores. Although there are proponents of enzymatic digestion for solid tissue dissociation, especially for the performance of flow cytometry on skin biopsies, we strongly advise against it. In our experience, enzymatic digestion of biopsies yields a tiny percentage of intact, viable cells available for analysis. Consequently, enzymatic digestion typically destroys tissue that might be used for histology, while providing little or no diagnostic MFC data.
Most antigens currently evaluated are cell surface associated, but cytoplasmic and nuclear antigens (e.g., terminal deoxynucleotidyl transferase, or TdT, in lymphoblast nuclei) also can be evaluated by flow cytometry by using permeabilization techniques. A number of commercially available reagents are available for fixation and permeabilization of cells prior to the addition of antibodies to cytoplasmic and nuclear antigens. When both cell surface and cytoplasmic antigens are evaluated in the same assay, the surface staining is performed first. The cells then are fixed and permeabilized, followed by staining for cytoplasmic and nuclear antigens. It is important to note that the permeabilization process introduces some amount of artifactual, background “shift” in antigen expression. Assessment of antigen expression in permeabilized cells must be performed using the level of expression of known positive and negative cellular populations as controls.
Instrument Configuration and Quality Control
Deciding upon the number of antigens to simultaneously evaluate is dependent on the model of flow cytometer used. Single-laser flow cytometers typically are used to evaluate three or four antigens simultaneously, in addition to the two light scatter properties of forward scatter (proportional to cell size) and side scatter (also known as orthogonal or 90-degree light scatter, which is proportional to cytoplasmic complexity and granularity). Two-laser instruments can potentially evaluate six to eight antigens simultaneously, depending on the PMT configuration, whereas three-laser instruments generally are required to evaluate nine or more antigens simultaneously. Although most clinical flow cytometry laboratories use six- or eight-color flow cytometry for leukemia and lymphoma immunophenotyping, 10-color machines are now commercially available for clinical use. The simultaneous assessment of such a large number of antigens minimizes the number of tubes that must be set up, and therefore is of particular benefit for the analysis of paucicellular specimens, while offering the potential for cost savings. Regardless of the type of flow cytometer, quality control (QC) measures must be performed on a daily, weekly, and monthly basis to ensure optimal instrument performance. The required QC procedures are mandated by laboratory-certifying organizations, including Clinical Laboratory Improvement Amendments (CLIA), College of American Pathologists (CAP), and the appropriate arm of the Department of Health in each state. Daily QC measures typically use a stable fluorescence standard, such as brightly fluorescent microbeads, to ensure that the voltages allotted to the individual PMTs are adequate to detect the expected level of fluorescence from these microbeads. PMT voltages are typically set so that the autofluorescence of unstained lymphocytes (i.e., the innate fluorescence of the cell due to endogenous biomolecules such as flavins, in the absence of added antibodies) falls within the first decade of the logarithmic scale. On a less frequent basis, the linearity of detected fluorescence should be confirmed by using a series of microbeads with known fluorescence properties ranging from negative to very bright; in addition, acceptably low levels of cell carryover between specimens must be confirmed.
Antibodies: Compensation and Panel Design
Each fluorochrome has well-characterized absorption and emission spectra. Because the emission spectrum of each commonly used fluorochrome extends over a range of wavelengths (Table 2.1), the simultaneous use of multiple antibodies conjugated to different fluorochromes invariably results in some degree of spillover, in which a portion of the fluorescence from a given fluorochrome is detected by a PMT whose range of detected wavelengths is targeted for a different fluorochromes (e.g., a red fluorochrome is detected as red, but also as “orange,” possibly leading to false-positive expression of an orange-labeled antibody). As a result of this spectral overlap, the fluorescence detected by each PMT actually represents the sum of the fluorescence from multiple fluorochromes. Although most of the fluorescence typically comes from the fluorochrome, the PMT was designed to detect significant contributions may occur from other fluorochromes due to spillover. To adjust for the spillover fluorescence from
other fluorochromes, a mathematical correction, known as compensation (or color compensation), is applied routinely to all MFC data. Although a detailed discussion of compensation is beyond the scope of this chapter, it is important to recognize that proper compensation of flow cytometry data is another critical QC function.
other fluorochromes, a mathematical correction, known as compensation (or color compensation), is applied routinely to all MFC data. Although a detailed discussion of compensation is beyond the scope of this chapter, it is important to recognize that proper compensation of flow cytometry data is another critical QC function.
Antibodies typically are used in defined combinations, or panels, to answer specific questions about specific cell populations. For example, most laboratories that perform leukemia and lymphoma immunophenotyping have an acute leukemia panel designed to distinguish acute myeloid leukemia from acute lymphoid leukemia and a lymphoma panel to distinguish benign from malignant lymphoid tissue. (Table 2.2 shows commonly used diagnostic panels, Table 2.3 has descriptions of relevant, commonly used antigens, and Fig. 2.3 is a graphic representation of the most useful antigens.) For the most current, regularly updated information about antigens important in the evaluation of hematopoietic cell populations, the best, most user-friendly source can be found at http://www.sciencegateway.org/resources/prow/index.html.
Although a detailed discussion of flow cytometric panel design is beyond the scope of this chapter, suffice it to say that a great deal of thought must go into panel design. At a minimum, antibody panels should measure a sufficient number of antigens to distinguish normal and benign from abnormal and neoplastic cell populations with a high degree of sensitivity and specificity. However, the quality of the flow cytometric data also depends on how antibodies are used in combination. For example, when the detection of a dimly expressed antigen—such as an aberrantly expressed lymphoid antigen on myeloid blasts—is important, conjugation of the relevant antibody to a bright fluorochrome, such as phycoerythrin (PE), can maximize the chance of detecting such aberrant antigen expression. Conversely, it may be unwise to use a bright fluorochrome to detect a strongly expressed antigen, because the bright fluorescence emission will likely create compensation problems due to spillover.
When a specimen is received in the flow cytometry laboratory, it is important that the pathologist or flow cytometry technologist consider the underlying clinical question in deciding which panels to use. If the clinical question is a limited one, such as whether a bone marrow aspirate from a patient with known B-NHL has any evidence of disease, then it may be appropriate to perform a limited study focused primarily on the cell population in question, such as the mature B cells (e.g., tube B1; see Table 2.2). On the other hand, when the clinical question is much broader, for example, when ruling out a hematolymphoid neoplasm in the marrow of a patient with pancytopenia and no known malignancy, then a broader evaluation of the myeloid, lymphoid, and plasmacytic lineages is likely to be appropriate (e.g., tubes B1, T1, PL1, and AML4; see Table 2.2). When clinical concern exists for a lymphoproliferative disorder, then it is prudent to evaluate both the B cells and T cells, whenever possible. However, because B-cell lymphomas are much more common than T-cell lymphomas, if analysis is limited by a paucity of cells in the specimen, it is reasonable to rule out a B-cell malignancy before evaluating the T cells.
Data Acquisition
The actual collection of flow cytometric data, while the stained cells are propelled through the flow cytometer, illuminated by the lasers, and detected by the PMTs, is known as acquisition. In flow cytometry parlance, a particle of sufficient size to be detected is typically a cell, but is referred to as an “event” (e.g., a clump of platelets may register as an event). In general, “cell” and “event” are interchangeable terms. The number of events required for evaluation depends on both the purpose of the flow cytometric evaluation and the nature of the specimen. For example, if one is evaluating a lymph node that is replaced by lymphoma, acquisition of a relatively low number of events per tube of cells and antibodies (e.g., 10,000 events) may allow adequate characterization of the neoplastic population. By contrast, if one is looking for evidence of very low-level marrow or PB involvement by lymphoma, then a much larger number of cells (e.g., 100,000-500,000) should be evaluated. In most laboratories, if adequate cells are available, then at least 100,000 viable cells are routinely collected per tube. However, at least 500,000 cells will be collected to quantify stem cells in a specimen being evaluated for use in stem cell transplantation. Assuming that the presence of 50 cells would enable confident identification of a population, then the evaluation of 500,000 cells offers the ability to detect a population at a frequency of 1 in 10,000 cells, or 0.01%.
A potential artifact in the evaluation of flow cytometric data is carryover from one tube to the next, in which stained cells from previous specimens accumulate in the tubing of the instrument and are released in relatively small numbers as subsequent acquisition occurs. The hazard in carryover is that certain combinations of bound fluorochromes on the contaminating cells could lead to interpretation as a small abnormal cell population, suggesting the possibility of malignancy despite the fact that no such cell population truly exists in the specimen in question. Most clinical flow cytometers have manufacturer specifications guaranteeing no more than 1% carryover, and these instruments frequently demonstrate much less carryover in practice. However, if one is looking for MRD, then one should make every effort to eliminate carryover altogether. One way to accomplish this is to run plain sheath fluid through the instrument between tubes and to monitor the data generated by such a procedure to ensure that no increase occurs in the number of cellular events beyond the usual background level for the instrument.
Data Analysis
MFC data analysis requires specialized computer programs designed for this purpose, that are able to perform compensation, generate two-dimensional histograms and scatterplots (commonly referred to as “scattergrams,” also known as two-parameter dot plots) of the data that can be adjusted to define abnormal cell populations, and enumerate the various
cell populations of interest for reporting purposes (Fig. 2.4).* The process of targeting the analysis to the population of interest is known as gating. In this process, greater and greater numbers of extraneous cellular events are excluded from the analysis in a sequential manner, to maximize the likelihood of identifying an abnormal cell population. The first step is exclusion of doublets (cohesive aggregates of cells and/or platelets). Cohesive cell aggregates can be excluded by displaying time of flight (TOF; the time necessary for the event to pass the detector) and gating out events with a prolonged TOF. Aggregates can also be excluded by displaying forward light scatter (FS or FSC) area by height (Fig. 2.5, left). In the latter analysis, round objects (i.e., single cells) have FS area that is proportionate to FS height. Events falling off the 45-degree FS area/FS height are excluded because they represent doublets or aggregates.
cell populations of interest for reporting purposes (Fig. 2.4).* The process of targeting the analysis to the population of interest is known as gating. In this process, greater and greater numbers of extraneous cellular events are excluded from the analysis in a sequential manner, to maximize the likelihood of identifying an abnormal cell population. The first step is exclusion of doublets (cohesive aggregates of cells and/or platelets). Cohesive cell aggregates can be excluded by displaying time of flight (TOF; the time necessary for the event to pass the detector) and gating out events with a prolonged TOF. Aggregates can also be excluded by displaying forward light scatter (FS or FSC) area by height (Fig. 2.5, left). In the latter analysis, round objects (i.e., single cells) have FS area that is proportionate to FS height. Events falling off the 45-degree FS area/FS height are excluded because they represent doublets or aggregates.
After excluding cellular aggregates, nonviable cells are excluded, which is necessary due to their nonspecific antibody binding. Nonviable cells have porous membranes that leak out cytoplasmic contents and nuclei that are shrunken, so these events are compact with low forward and side scatter. Gating out low FS/low SS cells is a simple means of excluding dead cells from the analysis (see Fig. 2.5, middle). Forward versus side scatter gating also will exclude any residual, un-lysed erythrocytes, due to their small size and lack of granules. An alternative method for removing nonviable cells from the analysis is the addition of a viability dye, such as 7-amino-actinomycin-D (7-AAD), to the cell suspension just prior to data acquisition on the flow cytometer. 7-AAD penetrates the damaged plasma membranes of nonviable cells, but is excluded from viable cells. Therefore, cells demonstrating the characteristic fluorescence of 7-AAD are excluded from further evaluation by gating. Because 7-AAD emission complicates the process of MFC data compensation necessary for analysis, it is not used in most laboratories.
After cellular aggregates and dead cells have been excluded, the next step is separate gating of leukocyte subpopulations, which is performed by displaying CD45 expression and side scatter area SSC-A (Fig. 2.5B, right; see also Fig. 2.8). As such, a CD45 antibody must be in all tubes. WBC subpopulations typically fall into the lymphocyte gate (bright CD45, low SSC-A), monocytes (brighter CD45, higher SSC-A), neutrophils (intermediate intensity CD45 and high SSC-A), blasts (dim CD45 and low SSC-A), erythroid cells and plasma cells (PCs; negative CD45 and intermediate SSC-A), and hematogones (i.e., pre-B cells; dim CD45 and low SSC-A). After WBC are divided into these constituent groups, lineage-specific antibodies are used to delineate subgroups: CD19 is used to identify B cells, CD3 to identify T cells, CD7+/3−/5− to identify natural killer (NK) cells, bright CD38 to identify PCs, and CD34/CD117 to identify blasts.
The key to identification of a neoplastic population by MFC is finding a discrete abnormal population. This is distinguished from a continuum from normal to abnormal antigen expression (compare Figs. 2.6 and 2.7).
Aberrant antigen expression indicating a neoplastic population typically includes:
An abnormal decrease in the expected level of expression of an antigen in the cells of interest, including complete loss of expression (e.g., aberrant loss of CD7 on the neoplastic CD4+ T cells of mycosis fungoides (MF) or Sézary syndrome; see Fig. 2.7)
An abnormal increase in the expected level of expression (e.g., abnormally bright CD10 in B- acute lymphoblastic lymphoma [ALL] cells; Fig. 2.8)
Abnormally homogeneous expression of antigens that normally show variable expression in a population of interest (e.g., abnormally homogeneous expression of CD34 and CD117 on myeloid blasts in acute myeloid leukemia)
Aberrant expression of nonlineage antigens (e.g., aberrant expression of the T-cell-associated antigen, CD7, on leukemic myeloid blasts)
There are data supporting the use of asynchronous antigen expression, in which the timing of antigen expression during a maturational process is abnormal (e.g., asynchronous expression of CD13 and CD16 during neutrophil maturation in myelodysplasia), and this type of analysis is used by some practitioners for diagnostic purposes. However, assessment of maturation patterns is a highly nuanced process. Moreover, this type of analysis is not included in WHO diagnostic criteria. Lastly, all published studies show that cases diagnosed as neoplastic by this method are also diagnosed by accepted WHO criteria, making such flow analysis redundant. Lastly, no studies have shown strong data indicating that finding an “abnormal” maturation pattern is predictive of transformation to a clinical neoplasm.
Data Reporting
There is a great need to standardize data reporting. Although some attempts have been made, no recommendations have been widely adopted. A critical fact that must always be taken into account is that flow cytometry findings are reported to the clinical team, who typically have little or no practical experience in flow cytometry. As such, the interpretation must be clear and precise, rid of extraneous, possibly misleading information. For example, in a patient with a clinical query of CD8+ large granular lymphocyte leukemia, “r/o LGL,” reporting CD4:CD8 often is misleading, because, for example, an absolute decrease in CD4 cells will cause a ratio skewed toward CD8 cells. In this example, even if there is no expanded population of CD8 T cells, reporting the ratio is
potentially misinterpreted. Also, instead of reporting “viable, singlet events,” the best term is “all cells.” Simply reporting the percentage of cells in the population of interest that is considered positive for each of the antigens evaluated is not adequate and should be avoided. To indicate which populations were analyzed, and for billing purposes, a list of tested antigens must be included. The immunophenotype of each abnormal cell population, including the proportion of the total viable cells represented by each abnormal population should be reported in a free-text format. Descriptions of the fluorescence intensities of diagnostically relevant antigens are useful, especially considering that many reports are used as a basis for detecting residual disease after therapy. The addition of key two-dimensional histograms to the report can provide a snapshot of a neoplastic immunophenotype that can be of great help in assessing residual disease when subsequent specimens are received. The analysis and interpretation should be directed toward the clinical query or prior diagnosis. It should begin with a binary statement of positive or negative, followed by statements citing which cell populations were analyzed. If positive, the percentage of total cells should be cited along with the most salient immunophenotypic properties. What to include and exclude, and useful wording are as follows:
potentially misinterpreted. Also, instead of reporting “viable, singlet events,” the best term is “all cells.” Simply reporting the percentage of cells in the population of interest that is considered positive for each of the antigens evaluated is not adequate and should be avoided. To indicate which populations were analyzed, and for billing purposes, a list of tested antigens must be included. The immunophenotype of each abnormal cell population, including the proportion of the total viable cells represented by each abnormal population should be reported in a free-text format. Descriptions of the fluorescence intensities of diagnostically relevant antigens are useful, especially considering that many reports are used as a basis for detecting residual disease after therapy. The addition of key two-dimensional histograms to the report can provide a snapshot of a neoplastic immunophenotype that can be of great help in assessing residual disease when subsequent specimens are received. The analysis and interpretation should be directed toward the clinical query or prior diagnosis. It should begin with a binary statement of positive or negative, followed by statements citing which cell populations were analyzed. If positive, the percentage of total cells should be cited along with the most salient immunophenotypic properties. What to include and exclude, and useful wording are as follows:
Example of negative interpretation in a known lymphoma patient:
The following antibodies were used: CD2, sCD3, CD4, CD5, CD7, CD8, CD10, CD19, CD20, CD21, CD23, CD34, CD38, CD45, CD57, CD103, FMC7, HLA-DR, surface Kappa (sKappa), sLambda (total 20)
Abnormal cells were not detected. The B cells are polytypic and show no aberrant antigen expression. A discrete, abnormal T-cell population is not detected. These findings provide no evidence of the patient’s previously diagnosed lymphoma.
Example of positive interpretation in a known lymphoma patient:
The following antibodies were used: CD2, sCD3, CD4, CD5, CD7, CD8, CD10, CD19, CD20, CD21, CD23, CD34, CD38, CD45, CD57, CD103, FMC7, HLA-DR, sKappa, sLambda (total 20)
Monotypic B cells with bright expression of surface kappa light chains, CD5+, CD23−, bright CD20+ composed 15% of all cells. These findings are consistent with involvement by the patient’s previously diagnosed mantle cell lymphoma (MCL). A discrete, abnormal T-cell population is not detected. These latter findings provide no evidence of a T-cell neoplasm.
MFC for Nonneoplastic Disorders
The assessment of lymphoid subsets, used to monitor patients with AIDS, is the only widely used FDA-approved indication for flow cytometry in the United States. Unlike flow cytometry for neoplasia, which is performed somewhat differently in each institution, FDA approval mandates a precise method for lymphocyte subset analysis, including software with pre-drawn gates and unchangeable algorithms. There is no interpretation; simply a reporting of percentages and absolute numbers of B cells, NK cells, CD4+ T cells, CD8+ T cells, and the CD4:CD8 (the latter calculation only including T cells, defined by expressing CD3). Although specimens are sometimes received for the evaluation of other immune abnormalities, any interpretation of the results should be performed with great caution. For example, because the etiologic diagnosis of pulmonary fibrosis can be so difficult to confirm, in many institutions, bronchoalveolar lavage specimens are sent for CD4:CD8 by flow cytometry. There are some data suggesting that CD4:CD8 falls within certain ranges in pulmonary fibrosis, which correlate with the underlying etiology.9 However, because there are no normal values for CD4:CD8 in any site other than P, the ratio may be reported, but it cannot be interpreted as high or low. Similarly, there are no normal values for CD4+ or CD8+ T cell subsets in lymph nodes.
MFC for Fetomaternal Hemorrhage
Detection of fetal red cells in maternal circulation is necessary not only for the prevention of Rh D alloimmunization, but also for the diagnosis of clinically important fetomaternal hemorrhage (FMH) leading to fetal and neonatal morbidity and mortality. The rosette test is still the most widely used screening test in the United States. The most common confirmatory test remains the Kleihauer-Betke acid elution assay. Although a minority of laboratories use MFC, because the methodology employed is so different from that used for other MFC testing, technicians skilled in this analysis are rare. Also, the urgent need for results would be prohibitively disruptive to the MFC laboratory workflow. Moreover, because of the necessity of round-the-clock testing for FMH, an MFC laboratory would not serve because most are not open nights or weekends.10
MFC for Paroxysmal Nocturnal Hemoglobinuria
MFC is a standard method for diagnosis of paroxysmal nocturnal hemoglobinuria (PNH). In PNH, somatic mutation of the X-linked phosphatidylinositol glycan complementation class A (PIGA) gene causes a partial or absolute inability to make glycophosphatidylinositol (GPI)-anchored proteins. Antigens such as CD55, CD58, CD59, CD14, CD16, and CD24 are affected. The channel-forming toxin aerolysin and its precursor, pro-aerolysin, bind selectively and with high affinity to the GPI anchor. An inactive aerolysin variant conjugated with Alexa Fluor 488 (FLAER-A) is now widely used to detect GPI-anchor-deficient cell populations. Current guidelines include a combination of CD235a-FITC and CD59-PE for detection of GPI-deficient RBC, FLAER-A/CD24-PE/CD15-PECy5/CD45-PECy7 for detection of GPI-deficient granulocytes, and FLAER-A/CD14-PE/CD64-PECy5/CD45-PECy7 for GPI-deficient monocytes11 (Fig. 2.9). High-resolution assays allow detection of GPI-deficient RBC
at a level of 105 and GPI-deficient WBC at 104, which has been noted in patients with aplastic anemia and myelodysplastic syndrome (MDS).11
at a level of 105 and GPI-deficient WBC at 104, which has been noted in patients with aplastic anemia and myelodysplastic syndrome (MDS).11
MFC for Myeloid Neoplasms
WHO criteria for the diagnosis of myeloid neoplasms (described in other chapters) include morphology, genetics, and clinical features.1 Aside from confirming myeloid lineage of the neoplastic cells in acute myeloid leukemia (AML), immunophenotype is not included in WHO criteria for myeloid neoplasms. Still, flow cytometry can be useful in confirming that a myeloid population is neoplastic when diagnostic features are not known or are pending evaluation. Also, some WHO myeloid entities have distinctive immunophenotypic features that can be useful. Lastly, MFC is the basis of MRD detection in AML. What follows is a concise description of flow cytometry for clinically relevant purposes in myeloid neoplasms.
General Approach to Detecting Neoplastic Myeloid Cells by MFC
Unlike some lymphoma diagnoses, which can be made solely on the basis of MFC, for myeloid neoplasms, in general, MFC is used merely to confirm the immunophenotype, not as a primary diagnostic modality per se. When MFC shows a marked increase in blasts (Fig. 2.10), the percentage alone is diagnostic of acute leukemia; the remainder of the immunophenotype confirms myeloid lineage, e.g., CD117 expression and negative for lymphoid antigens. As with detection of any neoplastic population, the key to identifying neoplastic myeloid cells is recognizing a discrete population of abnormal cells, distinct from the normal expected background populations (see Fig. 2.7 for an application of this concept to detect neoplastic T cells). Aside from subtle abnormalities like abnormally dim or abnormally bright expression of expected antigens, detection of aberrant antigen expression is useful and important. The most useful, distinctive, and common antigens aberrantly expressed on neoplastic myeloid blasts are CD7 and CD56, which can be expressed by the neoplastic blasts in MDS, myeloproliferative neoplasms (MPN), or AML (see Fig. 2.10). CD7, a pan-T-cell antigen, is rarely expressed by nonneoplastic myeloid blasts. Although dim, partial coexpression of CD7 in a small blast population is not sufficient to confirm the neoplastic nature of myeloid blasts, finding a discrete population with uniform aberrant expression can be a useful adjunct in the diagnosis of a myeloid neoplasm. CD56, an NK cell antigen, is aberrantly expressed in some myeloid neoplasms, usually on blasts, but sometimes on neoplastic monocytic cells. Aberrant expression of CD56 is acquired in the malignant transformation of some solid organ cancers and also in myeloma. Again, dim/partial expression is not significant, but uniform expression by a distinct, discrete myeloid population is typically indicative of a neoplasm.
The most important aspect of MFC in a myeloid neoplasm is in cataloging and recording the distinct immunophenotype of the neoplastic cells prior to therapy. Aside from confirming an increase in cells with blast antigen expression (CD34 and/or CD117), an adequate initial analysis, including expression of numerous antigens, can be used as a template for future specimens from the same patient. The basis of MRD detection is in finding the same, discrete, distinctive population, based on the intensity of expression of a set of antigens. The most effective way to accomplish assessment of residual disease is to display data from the original, diagnostic specimen, side by side or overlapping, with data from the post-therapy specimen, using the same tubes and display criteria (Fig. 2.11).
MFC for Assessment of Myeloid Maturation Patterns and Mature Myeloid Cells, Including Monocytes and Granulocytes
Although there are publications describing the use of maturation patterns in mature myeloid cells (monocytes, neutrophils) to diagnose myeloid neoplasms, this is not a well accepted practice.12,13 There are no published criteria, WHO or otherwise, that include maturation pattern data for the diagnosis of any neoplasm. Diagnostic criteria for myeloid neoplasms in which the neoplastic cells are mature (e.g., chronic myelogenous leukemia [CML], chronic eosinophilic leukemia) involve a combination of clinical and genetic features. The use of flow cytometry in such diseases is limited to the assessment of blasts in the event that a blast crisis has been diagnosed by clinical and morphologic findings.
MFC for Diagnosis in Myelodysplasia and Myeloproliferative Neoplasms
Although there are numerous publications over the past decades, describing the use of MFC for assessing myeloid maturation patterns, for various reasons, MFC never became an accepted method for the diagnosis of MDS or MPN. MDS is diagnosed by morphology, clinical features, and cytogenetic analysis. MPN are diagnosed by the same features and also by including specific genetic analyses. Studies have shown that some practitioners may be capable of detecting MDS or MPN by MFC pattern recognition. However, this practice is highly user dependent. The same studies have shown that MFC does not provide an increase in sensitivity. In other words, a case thought to be MDS by MFC can be detected by standard microscopy. Also, cases with “abnormal maturation patterns” in which diagnostic criteria are not met are not found to have an increased future risk for development of a myeloid neoplasm. Moreover, when there is a need for another technique to diagnose an MPN or MDS, genetic analysis is far more specific and sensitive than MFC. In conclusion, the use of MFC in MPN and MDS is limited to the assessment of MRD, by showing the clear presence of the same, unique neoplastic population as was present at diagnosis.
MFC in Acute Myelogenous Leukemia
WHO criteria for the diagnosis of AML include finding at least 20% blasts that are proved to be myeloid by a combination of special stains (myeloperoxidase [MPO] and non-specific esterase [NSE]) and immunophenotypic analysis. The most
useful antigens in defining the myeloid lineage are expression of CD13 and/or CD33. Other useful myeloid-associated antigens include CD11b and CD15. Also important is the general lack of lymphoid antigen expression (although some lymphoid antigens may be aberrantly expressed by neoplastic blasts). By WHO criteria, a cell is a blast as determined purely by morphology.1 However, in most cases, blast morphology coexists with the expression of CD34 and/or CD117. Although CD117 is expressed by pronormoblast-stage erythroid precursors and by mast cells (MCs), CD34 expression in hematopoietic cells is limited to blasts. MFC is used to confirm the lineage in an acute leukemia but is not, according to WHO criteria, used to quantify blasts. The reason for this is that, for technical reasons, the blast quantity may be over or underestimated by MFC. For example, in some cases, the marrow aspirate submitted for MFC is diluted by PB (hemodilution), causing an artifactually low number to be quantitated. Hemodilution, a marrow specimen composed mainly of PB, can be a problem in marrow evaluations, but is most commonly problematic in CSF specimens where CSF often is diluted by blood because of a traumatic spinal tap procedure (Fig. 2.12).
useful antigens in defining the myeloid lineage are expression of CD13 and/or CD33. Other useful myeloid-associated antigens include CD11b and CD15. Also important is the general lack of lymphoid antigen expression (although some lymphoid antigens may be aberrantly expressed by neoplastic blasts). By WHO criteria, a cell is a blast as determined purely by morphology.1 However, in most cases, blast morphology coexists with the expression of CD34 and/or CD117. Although CD117 is expressed by pronormoblast-stage erythroid precursors and by mast cells (MCs), CD34 expression in hematopoietic cells is limited to blasts. MFC is used to confirm the lineage in an acute leukemia but is not, according to WHO criteria, used to quantify blasts. The reason for this is that, for technical reasons, the blast quantity may be over or underestimated by MFC. For example, in some cases, the marrow aspirate submitted for MFC is diluted by PB (hemodilution), causing an artifactually low number to be quantitated. Hemodilution, a marrow specimen composed mainly of PB, can be a problem in marrow evaluations, but is most commonly problematic in CSF specimens where CSF often is diluted by blood because of a traumatic spinal tap procedure (Fig. 2.12).
MFC in Specific AML Subtypes
Although AML subtypes must be confirmed by clinical features and cytogenetics, as MFC data typically become available within only a few hours or a day, whereas cytogenetics typically takes much longer, it is sometimes useful to provide a preliminary subtype assessment by MFC (Table 2.4).
AML with t(8;21)(q22;q22)
In addition to a markedly expanded myeloid blast population identified by CD45 versus SSC-A gating, this AML typically shows a relatively prominent population of maturing granulocytes, reflecting the underlying maturation characteristic of this entity. CD34 typically is expressed brightly, frequently in combination with aberrant CD15 that is normally a more mature myeloid antigen, not expressed on blasts. There is characteristic aberrant expression of the B-cell-associated antigen CD19, and CD56 and TdT also may be aberrantly expressed (see Table 2.4).
AML with t(15;17)(q22;q12)
This entity, also known as acute promyelocytic leukemia (APL), is a leukemic proliferation of promyelocytes. Because HLA-DR is normally expressed by myeloblasts but lost upon maturation to the promyelocyte stage, it follows that APL cells typically do not express HLA-DR (see Table 2.4). Similarly, as CD34 is expressed by normal myeloblasts but lost upon maturation to promyelocytes, it follows that CD34 is typically negative in APL. However, because the morphology alone is typically diagnostic, especially in combination with extremely high expression of MPO, MFC is neither important nor particularly helpful in the diagnosis of most cases of APL. Moreover, WHO criteria require FISH or cytogenetic documentation of the t(15;17) or a variant translocation to confirm the diagnosis. The take-home message is that HLA-DR negativity is neither unique to APL nor particularly helpful for diagnosis in real-life practice. If APL is a diagnostic consideration, the clinical team should be alerted immediately and cytogenetic analysis expedited.
MFC in Acute Leukemias of Ambiguous Lineage, Including Mixed Phenotype Acute Leukemia, Acute Bilineal Leukemias, and Undifferentiated Acute Leukemia
Mixed phenotype acute leukemia is characterized by blasts that coexpress myeloid and T or B lineage-specific antigens or concurrent B and T lineage antigens. Acute biphenotypic leukemia is rare, and coexpression of markers for all three lineages is extremely rare. The WHO contrasts this entity with acute bilineal leukemias, because the latter include two or more discrete blast populations, each of which demonstrates the features of a single lineage (Table 2.5; for an example of acute bilineal leukemia, see Fig. 2.13). In such cases, IHC often is a helpful adjunct in the immunoarchitectural demonstration of separate blast populations in separate spatial areas (Figs. 2.14 and 2.15; see Table 2.5). In cases in which surface antigen evaluation is ambiguous for lineage, cytoplasmic antigen evaluation frequently is helpful. In this context, specific B-lymphoid-associated markers include cytoplasmic CD79a, cytoplasmic immunoglobulin M (IgM), and cytoplasmic CD22. The most specific T-lymphoid-associated antigens include membranous or cytoplasmic CD3 or the T-cell receptor (TCR), whereas cytoplasmic myeloperoxidase is considered the most specific myeloid-associated antigen. Because no well-defined immunophenotype exists in mixed phenotype acute leukemia, these cases must be evaluated on a case-by-case basis. Finally, both mixed phenotype acute leukemia and acute bilineal leukemia should be distinguished from undifferentiated acute leukemia. According to the WHO classification, the latter is characterized by an absence of lineage-specific antigens. Non-lineage-specific antigens expressed in undifferentiated leukemias include HLA-DR, CD34, and CD38, with a subset of cases expressing TdT and CD7. Note that CD7 is not considered to be T lymphoid specific if only expressed at low levels, in the absence of other T-cell-associated antigens.
MFC for Precursor Lymphoid Neoplasms
Although the official WHO terminology differs, in common parlance, these cancers are still referred to as ALL, B-ALL being implied if T-ALL is not specified. These neoplasms are defined by lymphoblast morphology and confirmed by immunophenotype.
B-lymphoblastic leukemia/lymphoma (B-ALL) has characteristically low expression of CD45 and low SSC (Fig. 2.16; see Fig. 2.8). They typically express CD10 and CD19 along with other B cell antigens (e.g., cytoplasmic CD79a and CD22). They also usually express the blast antigen, CD34, but usually lack expression of CD117. As the expression of surface immunoglobulin denotes mature B cells, B-ALL sometimes shows expression of cytoplasmic IgM, but is
generally negative for surface immunoglobulin expression (see Fig. 2.8). Expression of CD20 is variable, usually low or negative. Dim coexpression of the myeloid-associated antigens CD13 and/or CD33 is common. The most helpful antigen in the diagnosis of B-ALL is TdT, which is strongly expressed in most cases, while negative in mature B cells, both reactive and neoplastic. In AML, TdT is typically negative, but some cases show weak/partial expression of TdT
generally negative for surface immunoglobulin expression (see Fig. 2.8). Expression of CD20 is variable, usually low or negative. Dim coexpression of the myeloid-associated antigens CD13 and/or CD33 is common. The most helpful antigen in the diagnosis of B-ALL is TdT, which is strongly expressed in most cases, while negative in mature B cells, both reactive and neoplastic. In AML, TdT is typically negative, but some cases show weak/partial expression of TdT
Subtypes of B-ALL are defined by the presence of cytogenetic abnormalities, not by immunophenotype.
T-lymphoblastic leukemia/lymphoma (T-ALL) also has characteristically low expression of CD45 and low SSC, like other acute leukemias. They typically express various combinations of CD2, CD4, CD5, CD7, and CD8, but are usually negative for surface CD3. Cytoplasmic CD3 is usually expressed. Unlike nonneoplastic T cells, T-ALL cells often express CD10. They also usually express the blast antigen, CD34, but usually lack expression of CD117 as well as myeloid-associated antigens, like CD13 and CD33 (Fig. 2.17). As with B-ALL, TdT is perhaps the most useful antigen in the diagnosis of T-ALL and is strongly expressed in most cases. T-ALL is relatively rare. There are no subtypes.
MFC for B-Cell Non-Hodgkin Lymphoma
Of all hematopoietic neoplasms, MFC is most straightforward for B-NHL. Whereas a reactive population of mature B cells is composed of some that express kappa surface light chains (sIgκ+ and other cells that express lambda (sIgλ+, in a ratio of approximately two or three sIgκ+ cells to each sIgλ+ cell (Fig. 2.18), a monoclonal, neoplastic population is composed of all one or the other type of surface light chain. Whereas a population of some sIgκ+ and some sIgλ+ reactive B cells is called polytypic, a neoplastic population with only sIgκ+ or sIgλ+ is called “monotypic.” Although the term monotypic often is used interchangeably with “monoclonal,” technically, clonality can only be assessed at the genotypic level. As with MFC for other types of cancer, detecting a discrete population of abnormal cells is the diagnostic key. Focusing on κ:λ ratios can be misleading. For example, an sIgk+ lymphoma may exist in a specimen with a relatively large number of nonneoplastic B cells; because the background B cells have a normal κ:λ, the overall ratio may be skewed only slightly or not at all, depending on the size of the neoplastic population. However, in that same case, the typically discrete population of abnormal B cells may only become visible in scattergrams by the use of multiple antigen display strategies (Fig. 2.19). Also, some B-NHL do not express sIg at all; the finding of a discrete population of sIg(−) B cells in a lymph node usually indicates lymphoma and is most commonly seen in follicular lymphoma (FL) (Fig. 2.20). Also, CLL cells typically express sIg very dimly and are sometimes completely negative. In such cases, permeabilizing the cells and assessing cytoplasmic immunoglobulin light chain expression often shows clear monotypic expression.
Once a B-NHL is diagnosed on the basis of finding a discrete, abnormal, monotypic B-cell population, algorithms can be used for WHO subtyping. However, precise subtyping requires correlation with morphology. In most cases, a cytospin preparation made from the flow specimen is adequate. In some, FNA smear morphology is required. In others, a needle or an open biopsy is required.
If the monotypic population is composed of intermediate or large cells with disbursed chromatin, it is a high-grade B-NHL. If it is composed of small cells with clumped chromatin, it is low grade.
Most common high-grade B-NHLs:
Diffuse large B-cell lymphoma (DLBCL)
Burkitt lymphoma (BL)
Most common low-grade B-NHLs:
CLL
Follicular lymphoma (FL)
Mantle cell lymphoma (MCL)
Marginal zone lymphoma (MZL)
Chronic Lymphocytic Leukemia and Mantle Cell Lymphoma
The algorithm for low-grade B-NHL begins with assessment of CD5 coexpression (see Fig. 2.20B). CD5 is positive in CLL and MCL. Whereas CLL has dim CD20 and sIg, and MCL has relatively brighter CD20 and sIg, they can usually be distinguished by the expression of CD23, which is positive in CLL and negative in MCL. However, because the prognosis and therapy are vastly different for CLL and MCL, it is critical to assess the expression of cyclin D1, a nuclear antigen at the time of initial diagnosis. Cyclin D1 (formerly called bcl-1) is expressed by MCL but not CLL. Cyclin D1 expression is consequent to t(11;14), which can be detected by FISH; however, a definitive diagnosis can be established by demonstrating the standard immunophenotype (FISH is not required unless the immunophenotype is unclear). For technical reasons, principally the relatively low intensity of expression, cyclin D1 cannot be reliably detected by MFC. Cyclin D1 IHC can be performed on a cell block or tissue biopsy. Although there is a single publication describing cases of “cyclin D1-negative” MCL, the authors define it by an extensive gene panel showing similarity to MCL except for cyclin D1. Surrogate markers for the gene panel have been promulgated for the purpose of diagnosing cyclin D1-negative MCL, such as SOX11. However, published data for SOX11 expression are conflicting and published studies do not describe expression, for example, in extranodal MZL. Because MZL can rarely express CD5, this is an important gap in the published data. In summary, the existence of cyclin D1-negative MCL is neither firmly established nor well accepted.
Follicular Lymphoma and Marginal Zone Lymphoma
Once a low-grade B-NHL has been established by morphology and MFC showing monotypic sIg, if it is CD5−, the next fork in the algorithm is CD10 (Figs. 2.21 and 2.22). CD10 is positive in two-thirds of cases of FL but never in MZL. Although
BCL6 is always expressed by FL and never by MZL, it is a nuclear antigen and, like cyclin D1, cannot be assessed by MFC for technical reasons, chiefly the low level of expression. Although a low-grade, CD5−, CD10+ lymphoma is usually FL, a CD5−, CD10(−) lymphoma might be FL or MZL. If tissue or a cell block is available, morphologic assessment and BCL6 immunostaining can be performed to distinguish between them. If not, the diagnosis would be descriptive, “B-NHL, CD5−, CD10− with a differential including FL and MZL.” In most patients with these findings, a conclusive subtype may be inferred based on clinical findings. It should be noted that there are rare cases of CD5+ MZL. Also, in some cases, expression of some antigens is equivocal. Also, there are rare cases of CD10+ hairy cell leukemia (HCL), which can be mistaken for FL.
BCL6 is always expressed by FL and never by MZL, it is a nuclear antigen and, like cyclin D1, cannot be assessed by MFC for technical reasons, chiefly the low level of expression. Although a low-grade, CD5−, CD10+ lymphoma is usually FL, a CD5−, CD10(−) lymphoma might be FL or MZL. If tissue or a cell block is available, morphologic assessment and BCL6 immunostaining can be performed to distinguish between them. If not, the diagnosis would be descriptive, “B-NHL, CD5−, CD10− with a differential including FL and MZL.” In most patients with these findings, a conclusive subtype may be inferred based on clinical findings. It should be noted that there are rare cases of CD5+ MZL. Also, in some cases, expression of some antigens is equivocal. Also, there are rare cases of CD10+ hairy cell leukemia (HCL), which can be mistaken for FL.
Lymphoplasmacytic Lymphoma
For practical, diagnostic purposes, lymphoplasmacytic lymphoma (LPL) can be thought of as MZL limited to bone marrow: CD5−, CD10− (see Fig. 2.22). However, whereas MZL has plasmacytoid differentiation in only 30% of cases, LPL has it in 100%. LPL is usually associated with a monoclonal immunoglobulin serum spike of the IgM type. Waldenström macroglobulinemia (WM) is defined as the presence of a B-NHL and an IgM serum spike. Another helpful feature in the diagnosis of LPL is noting the presence of numerous MCs, which are accessory cells, secreting cytokines necessary for LPL growth. MCs can be found by routine morphology on aspirate smears, or immunostaining for MC tryptase. Also, the diagnosis of LPL/WM can be confirmed by the presence of an MYD88 gene mutation (described below).
Hairy Cell Leukemia
Like MZL, HCL shows monotypic sIg and is CD5−, CD10−(see Fig. 2.22). However, a more extensive panel shows expression of CD103, which is not seen in other B-NHL, and CD25, which is rarely seen in other NHL (Fig. 2.23). Although expression of CD11c is fairly nonspecific, in HCL it is extremely bright; this intensity is not seen in other lymphomas. Like MCL, HCL cells usually show cyclin D1 expression by IHC, but it does not present a diagnostic quandary because the HCL immunophenotype is otherwise so distinctive and different from MCL (e.g., HCL is CD5−). Although molecular confirmation is not required for HCL, most cases harbor a specific BRAF driver mutation, detectable by genetic sequencing (described below).
Diffuse Large B-Cell Lymphoma and Burkitt Lymphoma
Once a high-grade lymphoma has been diagnosed by the combination of morphology and MFC showing surface light chain restriction, a more complex assessment is required for subtyping. The cornerstone is histology (reviewed elsewhere in this text). If the specimen is limited to MFC and cytology (FNA or cytospins), it must be diagnosed descriptively, “mature B-NHL with a differential including DLBCL and BL.” Although a discussion of therapy is beyond the scope of this chapter, brief consideration is helpful and important when rendering a diagnosis, as a reminder of what is at stake. B-NHL can generally be divided into low grade and high grade (Fig. 2.24). For low-grade lymphomas, the clinical approach is “watch and wait”, expectant management (exceptions include local therapy for extranodal MZL and MCL may require induction chemotherapy and bone marrow transplant for MCL). By contrast, high-grade lymphomas are treated with induction regimens.
T-Cell Neoplasm Diagnosis by MFC
Whereas the algorithmic approach to B-NHL diagnosis by MFC is fairly straightforward, T-NHL diagnosis by MFC often is difficult or impossible. Also, the misdiagnosis of T-NHL by flow cytometry is a well-known pitfall. Whereas the process of B-cell ontogenic maturation is limited to peripheral lymphoid organs, T-cell populations are highly plastic at all sites. Consequently, MFC results for analysis of reactive B-cell populations are predictable, but analysis of T-cell populations is highly variable. Also, temporary “abnormal” T-cell populations commonly arise during immune reactions and can be seen in numerous clinical scenarios in patients with different kinds of illnesses. Consequently, whereas diagnostic criteria for B-NHL do not require persistence of the abnormal population (i.e., finding a B-NHL at a single point in time is diagnostic), for some T-NHLs, WHO criteria require demonstrating persistence of the abnormal T-cell population for at least 6 months. Whereas MFC can be diagnostic in B-NHL as a standalone test, MFC for T-NHL should be thought of as an adjunct, not the primary means of establishing a diagnosis.
The cornerstone of T-NHL diagnosis by MFC is demonstration of a discrete population with loss of pan-T-cell antigen expression. All peripheral (i.e., post-thymic) T cells express CD2, CD3, CD5, and CD7. MFC for T-NHL begins with assessment of T cells, as defined by expression of CD3, and looking for coexpression of CD2, CD5, and CD7 (Fig. 2.25; see Fig. 2.6). In addition, T cells should express either CD4 or CD8 (neither double-negative nor double-positive). Consequent to its use in monitoring AIDS patients, most practitioners are familiar with the normal CD4:CD8, being in the range of 1.1 to 2.3 in most centers (the CD4:CD8 must be limited to T cells, CD3+, because NK cells can express CD8 and artifactually skew the ratio). It must be emphasized that this ratio only applies to PB T cells. Normal ranges are not available for any other site. Anecdotally, in a nonneoplastic marrow, the CD4:CD8 often is weighted toward CD8+ T cells and in reactive lymph nodes, it often is heavily weighted toward CD4+ T cells. “Normal” CD4:CD8 only applies to PB and must not be applied to a specimen from any other site. If, upon examination of the initial antigen panel, a T-NHL is suspected, a second panel of antigens should be performed for confirmation and subtyping (Fig. 2.25B).
Even in the PB, CD4:CD8 is not useful in the diagnosis of T-NHL. For example, in a blood sample received with the
clinical query “r/o T-LGL” (a CD8+ neoplasm), reporting a CD4:CD8 can be misleading; it may be low, not because of an increase in CD8+ cells, but because of a decrease in CD4+ cells. Unlike B-NHL, in which finding even a small monotypic population is typically diagnostic of lymphoma, when assessing blood for T-NHL, absolute numbers must be reported (Table 2.6). Because any clinically relevant neoplasm is an abnormal growth, diagnosis of a neoplasm must be based upon demonstration of an expanded population. Because minor “abnormal” T-cell subsets are common, especially in sick patients, diagnosis of a T neoplasm should not be made in a blood specimen unless there is a clear, numeric expansion of a T-cell population.
clinical query “r/o T-LGL” (a CD8+ neoplasm), reporting a CD4:CD8 can be misleading; it may be low, not because of an increase in CD8+ cells, but because of a decrease in CD4+ cells. Unlike B-NHL, in which finding even a small monotypic population is typically diagnostic of lymphoma, when assessing blood for T-NHL, absolute numbers must be reported (Table 2.6). Because any clinically relevant neoplasm is an abnormal growth, diagnosis of a neoplasm must be based upon demonstration of an expanded population. Because minor “abnormal” T-cell subsets are common, especially in sick patients, diagnosis of a T neoplasm should not be made in a blood specimen unless there is a clear, numeric expansion of a T-cell population.
For most WHO classification of T-NHLs, MFC is not a reliable mainstay for diagnosis. The remainder of this section will focus on entities for which MFC is most often used and/or most useful. It should be emphasized that the gold standard and most sensitive means for diagnosing recurrent or residual T-NHL is PCR. In particular, when TCR PCR analysis shows a clonal population in the original, diagnostic specimen, PCR is run on subsequent specimens, comparing DNA from the original sample with DNA from the new sample, a technique that can confirm the presence or absence of the neoplastic clone.
T-Cell Large Granular Lymphocyte Leukemia
Because T-cell large granular lymphocyte leukemia (T-LGL) is commonly associated with neutropenia, submission of a PB specimen for MFC in patients with clinically significant neutropenia is a standard part of the workup. As detailed above, the focus is on the demonstration of an abnormal T-cell population of an expanded magnitude (Fig. 2.26; see Table 2.6). WHO criteria for T-LGL diagnosis require demonstration of an expanded, abnormal T-cell LGL population in blood that persists for at least 6 months, typically between 2,000 and 20,000 cells/mm3. A method must be employed to quantify the neoplastic cells (see Fig. 2.26). As with most T-cell neoplasms, the most common MFC abnormality is loss of CD7 expression. Reactive CD7− T cells exist in most specimens. Typically, displaying CD3 versus CD7 in nonneoplastic T cells shows a teardrop-shaped scattergram, in which the CD3+ T cells include a majority population with strong coexpression, trailing down to a minority subpopulation with low/negative CD7 (see Figs. 2.6 and 2.25A). This is distinctly different from finding a normal population and a discrete, separate population with uniform low/negative CD7 (see Fig. 2.7). This same concept applies to the other pan-T-cell antigens, CD2 and CD5. The typical immunophenotype of T-LGL: CD2+, CD3+, CD4−, CD5+/−, CD7+/−, CD8+, CD16+, CD56−, CD57+. However, variations exist, including rare CD4+ cases.
Mycosis Fungoides and Sézary Syndrome
MF is the most common cutaneous T-cell lymphoma and is diagnosed by a skin biopsy. Sézary syndrome is the advanced, generalized form of MF, with systemic involvement. MFC is a mainstay of diagnosis for SS. Although leukemic cells can be found in the PB in many patients with MF, the presence of a small population is not of prognostic significance. The development of Sézary syndrome indicates transformation from an indolent disease (MF) to an aggressive disease with poor survival. Also, some patients present with Sézary syndrome, never having had a clinical diagnosis of MF. WHO criteria for the diagnosis of Sézary syndrome require erythroderma, generalized lymphadenopathy, and the presence of clonal T cells in skin, lymph nodes, and blood. In addition, WHO criteria require at least one of the following, as established by MFC of a blood sample: a neoplastic cell count of at least 1,000/mm3 (see method for quantitation; see Fig. 2.26), an expanded CD4+ T-cell population resulting in a CD4:CD8 ratio below 10, and/or loss of at least one pan-T-cell antigen.
Adult T-Cell Leukemia/Lymphoma
Although indolent forms have been described, adult T-cell leukemia/lymphoma (ATLL) is typically an aggressive, widely disseminated neoplasm in an acutely ill patient. Although it involves skin and marrow, because it is typically leukemic, a blood sample usually is submitted for diagnosis. The leukemic ATLL cells have distinctive, cerebriform, nuclei with highly convoluted contours, in most cases readily identifiable with routine microscopy. The immunophenotype, like most T-NHL, is typically CD2+, CD3+, CD5+, but with loss of CD7. Like MF and Sézary syndrome, the neoplastic cells are usually CD4+. Unusually strong/uniform expression of CD25 is seen in most cases. Although MFC can be diagnostic of a T-cell neoplasm in such patients, because the immunophenotype is not specific (e.g., aside from the intensity of CD25, it is nearly identical to MF and Sézary syndrome), PCR studies are mandatory. ATLL is caused by the HTLV1 virus, which is found in the neoplastic cells. PCR for HTLV1 is confirmatory. If PCR is negative, the diagnosis would be peripheral T cell lymphoma, not otherwise specified (PTCL, NOS), rather than ATLL. Serologic studies can reveal exposure to HTLV1, but are not confirmatory, because exposure is fairly common and the latency period typically lasts for decades. Also, as with all T-cell neoplasms, TCR gene PCR is a mainstay of diagnosis. Even if there is a firm diagnosis by other techniques, TCR analysis can establish that a clone was detected by PCR and the DNA extracted from the original specimen can then be used for comparative analysis on DNA from subsequent specimens, when looking for recurrent or residual disease.
NK Cell Neoplasm Diagnosis by MFC
NK cell neoplasms are extremely rare. NK cells are defined by the expression of CD2 and CD7, without CD3 or CD5 (Fig. 2.27). They show various combinations of CD8, CD16, CD56, and CD57. The diagnosis of the WHO entity, chronic lymphoproliferative disorders of NK cells (CLPD-NK), requires an expanded NK cell population (usually >2,000 cells/mm3) that persists longer than 6 months (in PB, the NK cell normal range is 94 to 484 mm3 [see Table 2.6; see Fig. 2.26 for absolute number calculation method]). Because
most patients are asymptomatic, a definitive diagnosis is not usually required. However, because NK cell populations express variable KIR-family receptor proteins, it is possible to define a neoplastic population by MFC with KIR subtype analysis. Because of the rarity and lack of clinical importance of CLPD-NK, most laboratories do not perform KIR analysis. In stark contrast, the WHO entity, aggressive NK cell leukemia, typically presents with fulminant disease in a clinically ill patient. The diagnosis of lymphoma is typically easy to make on the basis of a large population of circulating and/or infiltrative, markedly atypical lymphoid cells. The immunophenotype is like that of reactive NK cells (CD2+, surface CD3-negative) except that they show strong/uniform expression of CD56 (an antigen typically weak/variable in NK cells). Also, aggressive NK cell leukemia is Epstein-Barr virus (EBV)-related, so in situ hybridization or immunostaining for EBV is typically confirmatory.
most patients are asymptomatic, a definitive diagnosis is not usually required. However, because NK cell populations express variable KIR-family receptor proteins, it is possible to define a neoplastic population by MFC with KIR subtype analysis. Because of the rarity and lack of clinical importance of CLPD-NK, most laboratories do not perform KIR analysis. In stark contrast, the WHO entity, aggressive NK cell leukemia, typically presents with fulminant disease in a clinically ill patient. The diagnosis of lymphoma is typically easy to make on the basis of a large population of circulating and/or infiltrative, markedly atypical lymphoid cells. The immunophenotype is like that of reactive NK cells (CD2+, surface CD3-negative) except that they show strong/uniform expression of CD56 (an antigen typically weak/variable in NK cells). Also, aggressive NK cell leukemia is Epstein-Barr virus (EBV)-related, so in situ hybridization or immunostaining for EBV is typically confirmatory.
Plasma Cell Neoplasm Diagnosis by MFC
Whereas leukemias are readily diagnosable by MFC, this technique is problematic in myeloma. Leukemic cells are typically plentiful in blood and/or marrow and are readily identified by MFC. Leukemic cells typically survive well outside of the marrow and so they remain viable upon processing for MFC. By contrast, multiple myeloma (MM) cells typically cause stromal fibrosis, preventing their aspiration14,15 (Fig. 2.28). Consequently, there are, on average, 20% fewer MM cells in aspirate smears than in the corresponding marrow core biopsy. A trephine core biopsy is the gold standard specimen type for myeloma diagnosis. Also, unlike leukemia, MM cells die quickly after removal from the marrow stroma (Fig. 2.28). Also, studies have shown that the immunophenotype in the tiny population of MM cells detectable by MFC usually differs significantly from the majority, in vivo population making the assessment of prognostic or predictive markers problematic, if performed by MFC. For example, MM cells typically show strong/uniform coexpression of CD56 and CD138, clearly demonstrable by core biopsy IHC; however, the MM cells detected by MFC often are “negative” in aspirated cells from the same patient (Fig. 2.29). Lastly, all gating strategies for MM depend on CD38 expression, which is problematic because CD38 is downregulated in patients treated with anti-CD38 drugs, which are becoming standard therapy for myeloma. All current, published MM gating strategies rely on CD38; alternatives are not known. For these reasons, bone marrow core biopsy immunostaining is the best and most accurate technique for analysis of myeloma (see below).
In MFC, PCs are typically gated by bright expression of CD38 and coexpression of CD138 or dim CD45 (see Figs. 2.1 and 2.29). Many cell types express CD38, but usually not as brightly as PCs. CD138 is specific to PCs but is technically problematic because expression can be affected by antibody clone, processing solutions, and time.16 CD138 expression decreases rapidly in PCs ex vivo during processing for MFC.17 Also, CD138 shedding by MM cells is induced by chemotherapy, which would decrease detection of MM cells by MFC in the post-therapy setting.18 Normal PCs express dim CD19 and dim CD45 but not CD56, whereas MM cells are typically negative for CD19 and CD45 but positive for CD56. However, there are numerous, different neoplastic populations seen in the EuroFlow panel, as well as more than 50 populations of normal PCs.2,19 Also, there is great variability in antigen expression from case to case. CD45, for example, is usually negative in MM, but may be expressed at any level from case to case.16 Moreover, the immunophenotype of any cancer often changes after therapy or at relapse. Also, after intensive therapy and especially after marrow transplantation, reconstitution of the immune system gives rise to transient “abnormal” PC populations, which can easily be misinterpreted as residual disease.20 For these reasons, although MFC is the gold standard for MRD assessment in acute leukemias, it is problematic for MRD in MM.
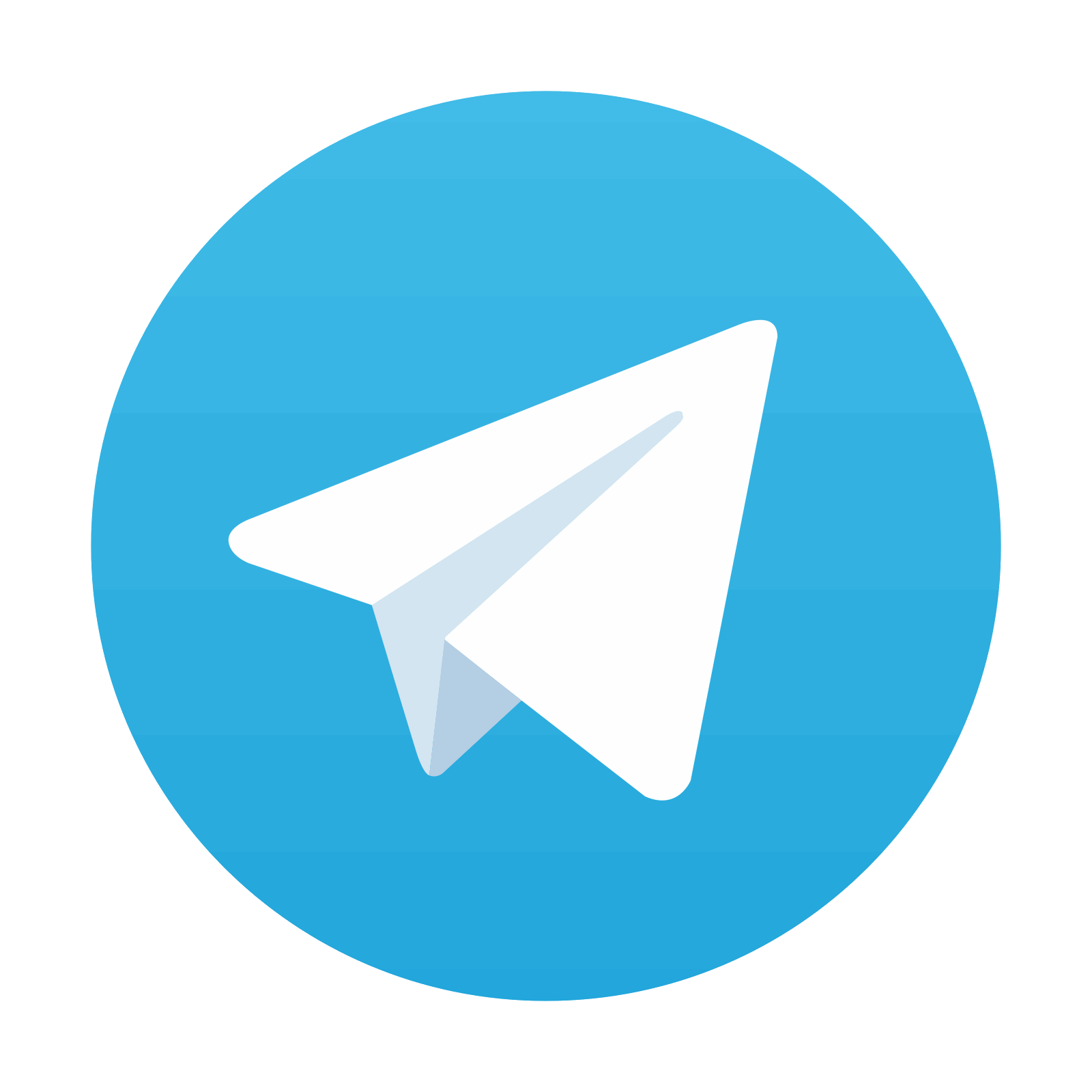
Stay updated, free articles. Join our Telegram channel

Full access? Get Clinical Tree
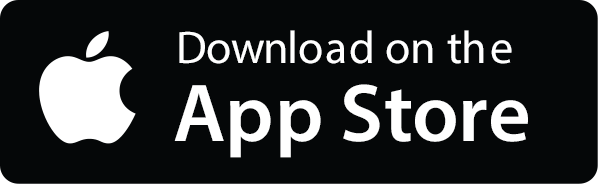
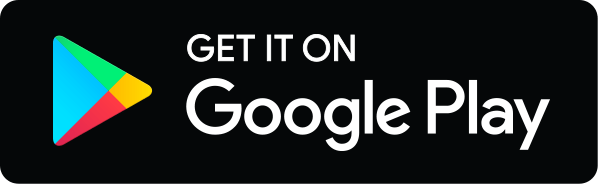