Anemia
Joshua A. Hayden, PhD
Babette B. Weksler, MD
The criteria for anemia depend upon age, gender, ethnicity, and pregnancy status. The World Health Organization (WHO) has defined anemia as a hemoglobin level below 13 g/dL (hematocrit [Hct] <39) in adult males and below 12 g/dL (Hct <36) in adult females.1 For persons of African origin, the lower limit of normal hemoglobin is about 0.5 g/dL less.2 In newborns, hemoglobin level averages 16.5 g/dL, falling to 12 g/dL between age 6 months and 2 years, then rising to normal adult levels by age 6 years. For accurate diagnosis of anemia, hemoglobin levels must be assessed with a reliable method, such as the cyanmethemoglobin method.3 Maintenance of a normal hemoglobin level requires good nutrition, normal gastrointestinal (GI) function, and absence of chronic diseases. Thus, although anemia is common in the elderly, in most cases it is not caused by normal aging; rather anemia is secondary to the effects of other diseases, especially chronic renal or inflammatory disease.4 Whether normal aging may be the cause of some anemia in the elderly is under active research.
Physical signs and symptoms caused by anemia depend upon rate of onset, exercise tolerance, and degree of anemia, and may include weakness, pallor, exertional dyspnea, tachycardia, dizziness, or poor concentration. Usually, tissue oxygen supply is not limiting for normal activity in anemia if the hematocrit is above 20%, unless the subject has underlying heart disease. In patients with coronary artery disease, angina may develop or worsen if anemia is present. Diminished tissue oxygenation may provoke the compensatory mechanisms of tachycardia and increased force of ventricular contraction, which patients sometimes detect as palpitations.
Physical examination may be unremarkable, or may show pallor of the conjunctiva, palms, and face, or in the presence of a hemolytic anemia, jaundice as well as pallor. A smooth or painful tongue may occur, especially with iron deficiency or macrocytic anemias. Flat, spoon-shaped nails (koilonychia) are seen in severe iron deficiency. Systolic heart murmurs, usually in the pulmonic area, can develop in anemia, probably resulting from a combination of lowered blood viscosity and increased blood flow across the heart valves. Pulmonary rales (“crackles”) or peripheral edema may reflect heart failure. Retinal examination in severe anemia may reveal hemorrhages that are white-centered (Roth spots), flame-shaped, or round. Some may be preretinal. The retinal veins are sometimes tortuous, and cotton wool spots, representing infarction of the nerve fiber layer, may occur. Ischemia of the vessels can lead to leakage of proteinaceous material, causing “hard” exudates.
Anemias are classified either morphologically (by erythrocyte size or shape) or by mechanism of hemoglobin reduction (decreased erythrocyte [RBC] production or increased RBC loss, either through increased RBC destruction or increased RBC loss as in bleeding). Normal RBCs are flexible biconcave discs about 8 µm in diameter with little variation in size or shape. Any deviation from normal morphology is associated with shortened RBC lifespan. The morphologic schema divides anemia into three groups, based on RBC mean corpuscular volume (MCV): (1) normocytic (MCV 90-100 fL); (2) macrocytic (MCV >100 fL); and (3) microcytic (MCV <80 fL). In some anemias, the RBCs may vary considerably in size and shape and result in mixed morphologies. In hypothyroidism, for example, the RBCs may be normocytic or macrocytic. A valuable aspect of morphologic classification of anemia is that the measurement of RBC size is rapidly available from automated blood counts, and the differential diagnosis of microcytic and macrocytic anemias is limited. In contrast, the causes of normocytic, normochromic anemias are more numerous and complex (see below).
RBC production can be estimated from the abundance of erythroid precursors seen on bone marrow examination, but the simplest measure is by evaluating the number of newly released RBCs, i.e., reticulocytes, in the peripheral blood. Reticulocytes actively synthesize hemoglobin, and detection of their ribosomal RNA requires staining of RBCs with RNA-binding dyes, such as new methylene blue, revealing blue granules or networks of RNA, hence the name “reticulocyte.” Automated cell counters similarly use a fluorescing
RNA-binding dye like acridine orange to detect reticulocytes; the reticulocyte count is expressed as an absolute number per volume of blood or as a percentage of the red cells. The percentage of reticulocytes needs to be corrected for degree of anemia by multiplying it by the patient’s hemoglobin (or hematocrit) divided by the normal value. For a hematocrit of 20% and a reticulocyte count of 6%, for example, the corrected reticulocyte count would be 6% × (20/45) = 2.6%. When the anemia is severe and polychromatophilia (a bluish tinge to the RBC cytoplasm) is prominent on the smear, a second correction is necessary because polychromatophilic RBCs circulate for 2 to 3 days, rather than for 1 day. The reticulocyte percentage that emerges from these corrections is called the reticulocyte index.
RNA-binding dye like acridine orange to detect reticulocytes; the reticulocyte count is expressed as an absolute number per volume of blood or as a percentage of the red cells. The percentage of reticulocytes needs to be corrected for degree of anemia by multiplying it by the patient’s hemoglobin (or hematocrit) divided by the normal value. For a hematocrit of 20% and a reticulocyte count of 6%, for example, the corrected reticulocyte count would be 6% × (20/45) = 2.6%. When the anemia is severe and polychromatophilia (a bluish tinge to the RBC cytoplasm) is prominent on the smear, a second correction is necessary because polychromatophilic RBCs circulate for 2 to 3 days, rather than for 1 day. The reticulocyte percentage that emerges from these corrections is called the reticulocyte index.
The reticulocyte count is important in distinguishing hypoproliferative anemias from ineffective erythropoiesis or increased bone marrow response to acute hemorrhage or hemolysis. The reticulocyte index, the bone marrow findings, and measures of hemolysis such as serum bilirubin and lactate dehydrogenase (LDH) permit an accurate designation of the type of anemia.
MICROCYTIC ANEMIA
The major causes of microcytic anemia are iron deficiency, the thalassemias, and anemia of chronic disease (ACD; in which microcytosis occurs in about 30% of cases). Less common causes include certain microcytic hemoglobinopathies such as hemoglobin C or hemoglobin E, in which latter microcytic anemia is accompanied by ineffective erythropoiesis with excess destruction of the developing, abnormal RBC within the bone marrow and sideroblastic anemias characterized by deficiencies in heme or porphyrin synthesis (either acquired, as in lead poisoning, or genetic).5 In iron deficiency, thalassemias, and microcytic hemoglobinopathies, the MCV is often smaller than in ACD, and the blood smear often discloses dramatic variations in RBC size and shape. This anisocytosis is captured in the RBC distribution width (or RDW) parameter.
Anemia of Iron Deficiency
It is estimated that there are more than 2 billion cases of iron deficiency anemia in the world making iron deficiency the commonest cause of microcytic anemia worldwide.6 Iron deficiency is caused by blood loss, inadequate dietary iron, rapid growth without iron supplementation, or poor iron absorption. Iron is normally the most abundant trace metal in the human body, totaling 3 to 4 g, mainly contained in hemoglobin, myoglobin, and enzymes; only a minor fraction, about 1 g, is stored as a reserve for production of new hemoglobin. Normal iron balance is tightly maintained, with daily needs of about 1 mg of absorbed iron, reflecting a dietary intake of about 20 mg, to balance obligatory excretion of about 1 mg of iron in sweat, shed skin or shed mucosal cells. Little absorbable iron is present in many foods, including most fruits and vegetables and grains, where iron is tightly complexed to phytates. Good iron sources are meat, poultry, fish, eggs, and legumes. Because daily iron loss is slight in adult males, men need little dietary iron, and deficiency from inadequate dietary intake is uncommon in men. When iron utilization is increased as in infancy, during growth spurts, and in pregnancy, or when concurrent blood loss occurs, as in menstruation, dietary iron intake may be insufficient, especially in women and children who tend to consume less than the recommended minimal daily requirement.7,8 The problem increases during pregnancy, when maternal iron is diverted to the fetus for hematopoiesis and with breastfeeding, as iron is lost in the milk.
Plasma iron levels are closely regulated by the hepatic peptide hormone hepcidin, which limits the release of newly absorbed iron from both enterocytes in the GI tract and from macrophages in the bone marrow and reticuloendothelial organs by binding to the membrane iron transporter, ferroportin, which promotes degradation of both proteins and thus limits the amount of iron bound to transferrin available to maturing erythroblasts for hemoglobin synthesis.9 Inflammation, chronic disease, and cancer, as well as high plasma iron levels, all favor increases in hepcidin, resulting in decreased iron availability, and thus, decreased erythropoiesis associated with these disease states. Conversely, in iron deficiency, hepcidin falls, promoting iron trafficking. Erythroferrone present on developing erythroblasts is a natural inhibitor of hepcidin. Numerous other proteins including hemojuvelin, bone morphogenic protein-2, SMADs, furin, and TMPRSS6 (transmembrane protease serine member 6) affect hepcidin transcription. During infection, stimulation of toll receptors can directly depress ferroportin levels independently of hepcidin and thus lower plasma iron levels; in addition, activated neutrophils release the iron-binding protein lactoferrin, which further limits the availability of iron required for bacterial growth.10
The reduction of body iron in developing iron deficiency anemia follows a standard pattern. Well prior to any anemia, iron stores (i.e., bone marrow ferritin) disappear, followed by a decrease in plasma iron levels and increase in unbound transferrin. Next, a normocytic anemia develops, which only with increasing iron deficiency becomes microcytic and then hypochromic. The reticulocyte count decreases. Thus, with mild and recent iron deficiency, the red cell indices (MCV, mean corpuscular hemoglobin [MCH], mean corpuscular hemoglobin concentration [MCHC]) and the blood smear remain normal, but over time, increasingly severe anemia develops and the MCV and MCHC fall. Anisocytosis, resulting in an increase in RDW, occurs. Later morphologic changes in iron deficiency anemia include poikilocytosis, microcytosis, and hypochromia. Tiny microcytes, elongated pale elliptical RBCs (pencil cells), and target cells may be visible on the blood smear, but some of the erythrocytes may appear normal. Often, the platelet count is elevated, with characteristically very small, single platelets. In overt iron deficiency anemia, the serum iron is markedly decreased, whereas the total
iron-binding capacity (i.e., transferrin level) is elevated, and the transferrin saturation is below 10%. The serum ferritin is markedly decreased (<20 ng/mL). Soluble transferrin receptors increase in iron deficiency but remain normal in ACD. Usually, the diagnosis of iron deficiency anemia is established by these tests, but occasionally, obtaining a bone marrow sample to verify the absence of iron stores or a trial of iron therapy may be necessary to confirm the presence of iron deficiency.
iron-binding capacity (i.e., transferrin level) is elevated, and the transferrin saturation is below 10%. The serum ferritin is markedly decreased (<20 ng/mL). Soluble transferrin receptors increase in iron deficiency but remain normal in ACD. Usually, the diagnosis of iron deficiency anemia is established by these tests, but occasionally, obtaining a bone marrow sample to verify the absence of iron stores or a trial of iron therapy may be necessary to confirm the presence of iron deficiency.
The clinical features of iron deficiency are generally similar to other anemias, e.g., fatigue, weakness, pallor, poor exercise tolerance, but three distinctive findings are only seen in iron deficiency anemia: pica, koilonychia, and blue sclera. Pica is the craving for, and ingestion of, certain unusual substances, such as starch, dirt, clay, cardboard, and ice (pagophagia). Koilonychia, marked by fingernails that become thin, brittle, and concave (spoon-shaped), is virtually pathognomonic of iron deficiency. Thinning of the sclera from impaired epithelial growth causes a blue tint because of the more visible choroid beneath.
Iron deficiency anemia in adults frequently results from chronic blood loss. The major cause of blood loss in women of childbearing age is heavy menstruation or repeated pregnancies. In non-menstruating women and in men, the most common reason for iron deficiency is GI hemorrhage. However, in resource-poor countries, lack of dietary iron or parasitic infestation with GI or genitourinary bleeding are common causes of iron deficiency. Infants, children, and pregnant women develop iron deficiency because of poor dietary intake of iron in the face of increased demands such as growth spurts or later stages of pregnancy (a developing fetus takes about 400 mg of iron from the mother). Other less common reasons for iron deficiency include chronic hematuria, epistaxes, hemoptysis, or intrapulmonary hemorrhage in such disorders as idiopathic pulmonary hemosiderosis, microscopic polyangiitis, or Goodpasture syndrome. In hereditary hemorrhagic telangiectasia, repeated mucosal bleeding from the vascular malformations may result in severe chronic iron deficiency anemia only remediable by parenteral iron repletion. Rare causes of iron deficiency include intravascular hemolysis from such diseases as paroxysmal nocturnal hemoglobinuria (PNH) or mechanical heart valve-related fragmentation of RBC, conditions which result in chronic intravascular hemolysis that leads to chronic urinary excretion of iron as hemoglobinuria (for PNH) or hemosiderinuria (for both).
Iron deficiency occasionally develops from iron malabsorption related to chronic malabsorptive GI disease such as inflammatory bowel disorders or sprue, or following gastric bypass surgery. Iron is absorbed throughout the GI tract, but best in the upper duodenum. In the presence of small intestinal disease, such as celiac sprue, or after gastric resection or bariatric surgery, which may accelerate the movement of intestinal materials through the duodenum and thereby diminish absorption time, iron deficiency may develop. Indeed, iron deficiency anemia, often refractory to oral iron, frequently develops within a year in the majority of patients who have undergone gastric bypass procedures and may require parenteral iron for correction.
A rare variant of iron deficiency anemia, iron-refractory iron deficiency anemia (IRIDA), representing a mutation in the TMPRSS6 gene, has improved our understanding of susceptibility to iron deficiency.11 In IRIDA, the TMPRSS6 mutation interrupts the normal pathway that inhibits hepcidin transcription, so that hepcidin levels remain high, leading to blocked GI absorption of iron, and a microcytic, hypochromic anemia develops that is refractory to oral iron. Anemia severe enough to require parenteral iron may be required in some menstruating women. However, as normal release of macrophage iron is also blocked, IRIDA patients will show increased iron stores when treated with parenteral iron. Studying other variants in the TMPRSS6 gene has revealed that some variants are associated with low hepcidin levels which have been described in blood donors with a relative resistance to development of iron deficiency, whereas carriers of variants with high hepcidin levels are more susceptible to iron deficiency anemia after blood donations. Similarly, male blood donors who carry a common polymorphism for the HIF-1 α gene that affects protein stabilization and hypoxia have higher hemoglobin and ferritin levels after blood donation than persons who have the wild-type allele. In the future, therapies that alter hepcidin concentration may become important in managing both iron deficiency and iron overload.12
Sideroblastic Anemia
Sideroblastic anemia results from impaired synthesis of heme. This impairment results in the presence of ring sideroblasts in the bone marrow. Ring sideroblasts are erythroblasts which contain a perinuclear ring of at least five blue granules representing iron-loaded mitochondria covering at least one-third of the nuclear circumference upon staining with Prussian blue. Sideroblastic anemia can be a genetic or acquired condition.13 The most common inherited form is X-linked sideroblastic anemia, which is caused by a germline mutation in the erythroid-specific aminolevulinic acid (ALA) synthase gene (ALAS2). ALAS2 catalyzes an early step in the synthesis of heme and mutation of this gene leads to impaired production. Because ALAS2 is an early step in the synthesis of heme, there is no buildup of toxic porphyrin metabolites or the associated symptoms seen with the porphyrias. Recent studies have identified mutations in a range of additional genes which can cause sideroblastic anemia. These include mutations in the glutaredoxin 5 (GLRX5) and the SLC25A38 genes, which encode mitochondrial proteins. Although X-linked sideroblastic anemia generally only affects males, there are documented cases of affected females as a result of autosomal dominance and lyonization. Acquired sideroblastic anemia is most often caused by myelodysplastic syndromes (MDS) or lead poisoning. MDS are a group of hematopoietic stem cell diseases with dysplasia in one or all of the major myeloid cell lineages; patients with MDS have a 15% chance
of developing acute leukemia. The WHO defines three distinct entities for MDS with sideroblastic anemia: refractory anemia with ringed sideroblasts (RARS), refractory anemia with ring sideroblasts associated with marked thrombocytosis (RARS-T), and refractory cytopenia with multilineage dysplasia with at least 15% ring sideroblasts [RCMD(+RS)].
of developing acute leukemia. The WHO defines three distinct entities for MDS with sideroblastic anemia: refractory anemia with ringed sideroblasts (RARS), refractory anemia with ring sideroblasts associated with marked thrombocytosis (RARS-T), and refractory cytopenia with multilineage dysplasia with at least 15% ring sideroblasts [RCMD(+RS)].
Beyond MDS, lead poisoning is a major cause of acquired sideroblastic anemia. Lead can inhibit porphobilinogen synthase, which leads to impaired heme synthesis. In addition, lead inhibits ferrochelatase, which catalyzes the insertion of iron into the completed porphyrin ring to produce heme. This inhibition results in the formation of zinc protoporphyrin (containing zinc instead of iron). Measurement of the zinc protoporphyrin:heme ratio can be used to detect lead poisoning. Beyond this, routine measurements of blood lead levels are possible using either atomic absorption spectroscopy or inductively coupled plasma mass spectrometry (ICP-MS).
MACROCYTIC ANEMIAS
Macrocytic anemias result from several mechanisms including slowed DNA synthesis in the bone marrow (leading to megaloblastic changes in RBC precursors), MDS, increased reticulocytosis, alcoholism, liver disease, hypothyroidism, and hemolysis or hemorrhage resulting in early marrow release of immature, large RBCs.14 In general, the macrocytosis in disorders where DNA synthesis is normal is mild (MCV 100-110 fL) and the enlarged RBCs are round, rather than oval, and often show polychromasia as they represent reticulocytes. Hypersegmented neutrophils, a feature of nutritional megaloblastic anemias, are not present in the nonmegaloblastic macrocytic anemias, except with myelodysplastic disorders.
Megaloblastic Anemia
Megaloblastic anemia is most often caused by nutritional deficiencies (especially deficiencies of folic acid or vitamin B12, vitamins essential for normal DNA synthesis) or medications that impair DNA synthesis or block folic acid metabolism, such as cytotoxic agents used in cancer chemotherapy or immunosuppression (e.g., cyclophosphamide, azathioprine, hydroxyurea, methotrexate, trimethoprim).15,16 In either instance, DNA synthesis is slowed whereas protein synthesis continues normally, resulting in abnormally large erythrocyte precursors (megaloblasts) in the bone marrow and large well-hemoglobinized RBCs (macrocytes) in the blood. In addition to the macrocytic anemia, macrocytic leukopenia and giant platelets are often present, and other nonhematopoietic cells such as skin cells and cervical mucosal cells are also macrocytic.
Nuclear maturation of hematopoietic cells in the bone marrow involves rapid cell division, which requires high levels of DNA synthesis. In situations where these high levels of DNA synthesis cannot be supported, as in deficiencies of essential nutrients, there is defective nuclear maturation of hematopoietic cells in the bone marrow.17 The defective nuclear maturation is primarily due to inadequate production of thymidine, an essential nucleic acid specific for DNA. Folic acid and vitamin B12 are essential in the production of thymidine from deoxyuridine, participating in methylation of the latter nucleotide. Tetramethyl folate is a methyl carrier, whereas vitamin B12 is the cofactor for the methyl transfer reaction. Whereas thymidine deficiency slows nuclear maturation and cell division, cytoplasmic maturation continues normally, as it is regulated by RNA (which does not require thymidine). As a consequence, hematopoietic precursor cells become megaloblastic, i.e., unusually large with relatively immature nuclei at each growth stage. This nuclear-cytoplasmic asynchrony is seen not only in erythroblasts but also in granulocyte precursors and megakaryocytes/platelets. The bone marrow is markedly hypercellular, but many of these megaloblastic precursors are destroyed in the marrow before release into the circulation, termed ineffective erythropoiesis. This intramedullary cell wastage is reflected by peripheral leukopenia and thrombocytopenia as well as anemia, and by elevated levels of serum iron, unconjugated bilirubin, and LDH.18 Early in megaloblastic anemias, mild macrocytosis may be the only abnormality in the peripheral blood, where only a small percentage of RBCs are actually macroovalocytes. As anemia worsens, the peripheral blood smear typically shows many RBC anomalies including anisocytosis, poikilocytosis, teardrop cells, schistocytes, and basophilic stippling. Polychromasia is uncommon, and the reticulocyte count is inappropriately low. Leukopenia and thrombocytopenia may occur. The presence of oval-shaped macrocytes, giant platelets, and hypersegmented neutrophils (>5% of cells with five lobes or any with six or more lobes) strongly suggests a megaloblastic process.
The clinical features of folic acid and vitamin B12 deficiency include signs of a hemolytic anemia such as pallor, mild jaundice, and exercise intolerance. The anemia may develop very slowly so that exercise intolerance is minimal even at very low hemoglobin levels. In addition, vitamin B12 deficiency can result in GI complaints, such as diarrhea and weight loss, and episodes of glossitis, leading to erythema, soreness, and, eventually, to loss of papillae, causing a smooth, beefy red tongue. Most importantly, vitamin B12 deficiency impairs nerve myelination, leading to degeneration of white matter in the brain and in both the dorsal and lateral columns of the spinal cord (subacute combined degeneration). Dorsal column involvement causes diminished vibratory sensation, creating numbness and tingling in the feet and hands (stocking-glove neuropathy), and decreased proprioception, producing gait difficulties and a positive Romberg sign. Lateral column damage causes limb weakness, spasticity, hyperactive reflexes, and a positive Babinski sign. Evidence of cerebral involvement includes depression, dementia, confusion, delusions, and hallucinations. With regard to folic acid deficiency, the symptoms of anemia are similar to those of B12 deficiency, but the neurologic changes do not occur, as folic acid is not involved in myelination in adults.
Folic acid deficiency is usually caused by an inadequate diet. Rich sources are fruits, vegetables, and animal protein, but cooking easily destroys folate. Furthermore, the body stores of folate are small, and only a few months of poor intake, caused by food fads, ignorance, poverty, or alcoholism, are necessary before anemia develops. A major concern for folate deficiency is in pregnancy where the folate requirement is increased and inadequate folic acid intake results in low birth rates and neural tube defects.
Alcohol intake compounds the problem by increasing urinary folate excretion, impeding liver storage of folate, and decreasing folate absorption, which occurs primarily in the duodenum and jejunum. Disorders affecting these portions of the intestine, such as sprue, lymphoma, amyloidosis, and Crohn’s disease, can cause folate malabsorption. Folic acid deficiency also can occur when the body’s demand for it increases, not only in pregnancy but also in any condition associated with increased cell turnover, such as chronic hemolytic anemias, acute exacerbations of hemolytic anemia, leukemia, and exfoliative dermatitis. Some medications, such as methotrexate and trimethoprim, cause folate deficiency by altering its metabolism. The measurement of serum folate level is very sensitive to recent folate intake and thus testing should be performed on fasting samples. Although some have advocated for the use of red cell folate as a more accurate indicator of long-term folate storage, this test has a number of analytical issues (including high coefficient of variation) that make it of limited value; published studies have shown that red cell folate performs no better than fasting serum folate measurements.19 Although folic acid deficiency is possible, recent studies have shown that the introduction of mandatory folic acid supplementation in cereal grain in the United States has drastically reduced the prevalence of folic acid deficiency in the population and has been associated with a documented reduction in neural tubular defects and as well as macrocytic anemia due to folate deficiency.
Vitamin B12 is the common name for either cyanocobalamin or hydroxycobalamin; these compounds are cobalamins, which include a group of molecules with a central cobalt atom bound by tetrapyrrole rings. In serum, methylcobalamin is the major form, whereas deoxyadenosylcobalamin is the primary form found in the cytosol. Cobalamins are not synthesized by humans but must be acquired in the diet, mainly meat, poultry, seafood, and dairy. The recommended daily allowance of cobalamins is 5 µg, and the total body content is about 2 to 5 mg, about 1 mg being present in the liver. Because the daily losses are minute, cobalamin deficiency from diet alone takes years and occurs almost exclusively in strict vegetarians, e.g., vegans. Therefore, in adults, the main cause of vitamin B12 deficiency is impaired absorption. Food cobalamin binds to a substance in gastric juice called R protein (haptocorrin) and is released by pancreatic enzymes when it reaches the second portion of the duodenum. Vitamin B12 then binds to intrinsic factor, a glycoprotein produced by the parietal cells in the fundus and cardia of the stomach, and the complex then travels to the distal ileum. Intrinsic factor receptors are present on the ileal mucosa, especially in the terminal ileum, where cobalamin is specifically absorbed from the cobalamin-intrinsic factor complex in a receptor-mediated process.
A major cause of cobalamin deficiency is from reduced intrinsic factor activity. This can occur from destruction of parietal cells by gastric resection or from chronic gastric inflammation due to autoimmune mechanisms that lead to mucosal atrophy in the stomach’s fundus and body. The latter disorder, pernicious anemia, occurs primarily in older adults, often with a family history of the disease; northern European descent; or concurrent autoimmune disorders, such as Graves disease, vitiligo, or Hashimoto thyroiditis. About 90% of patients with pernicious anemia have antibodies to parietal cells, compared with 5% in the general population, and approximately 60% have antibodies to intrinsic factor, which are rare in healthy people.
Disorders of the ileum, such as Crohn’s disease or lymphoma, can also cause cobalamin deficiency because of vitamin B12 malabsorption. Malabsorption of cobalamin also can occur with pancreatic insufficiency, when inadequate pancreatic enzymes fail to release cobalamin from the R proteins. Another cause of cobalamin deficiency is its consumption in the small intestine by a fish tapeworm, Diphyllobothrium latum, found mostly in fish from Canada, Alaska, and the Baltic Sea, and acquired by humans by eating undercooked fish or fish roe. Excessive intestinal bacteria in diseases associated with impaired motility, or intestinal stasis, such as systemic sclerosis, extensive diverticula, or surgical blind loops, also can consume enough cobalamin to cause vitamin B12-related macrocytic anemia.
Assessment of vitamin B12 levels is more complicated than folic acid levels. The Schilling test had previously been used to help distinguish among the causes of cobalamin deficiency. Unfortunately, because of technical difficulties producing and measuring the cobalt radioisotopes required, this test is no longer available in the United States. The most common tests offered clinically are quantitative immunoassays. These assays typically first convert serum cobalamins to cyanocobalamin and then quantify this species. Recently, approximation of vitamin B12 levels has been accomplished by measuring serum homocysteine and methylmalonic acid (MMA). Homocysteine is increased in both vitamin B12 and folate deficiency because methionine synthesis is impaired by deficiency of either. This testing has higher sensitivity because the increase occurs earlier in nutritional deficiency. A cobalamin-dependent, but folate-independent, enzymatic reaction leads to increased serum levels of MMA in cases of vitamin B12 deficiency. This finding also tends to precede changes in serum cobalamin. Accordingly, measurement of both homocysteine and MMA can reliably detect, and distinguish between, folate and cobalamin deficiencies. When both are elevated, cobalamin deficiency is confirmed, although concurrent folate deficiency is possible. If homocysteine is elevated and MMA is normal, folate deficiency is likely. If both are normal, deficiency of either is highly
improbable. If cobalamin deficiency is present, the presence of antibody against intrinsic factor confirms the diagnosis of pernicious anemia.
improbable. If cobalamin deficiency is present, the presence of antibody against intrinsic factor confirms the diagnosis of pernicious anemia.
Nonmegaloblastic Macrocytic Anemia
Macrocytic anemia also occurs frequently in MDS because of abnormal erythrocyte maturation and ineffective erythropoiesis caused by a clonal expansion of abnormal hematopoietic stem cells. Another source of mild macrocytosis is reticulocytosis, an increase in young erythrocytes released early from the marrow, in hemolysis. Reticulocytes may be presumptively identified on peripheral blood smears because they still contain residual blue-staining nuclear RNA, as well as red-staining hemoglobin, producing a purplish color with the Romanowsky stains ordinarily used for peripheral blood films. The presence of a few reticulocytes is common on normal smears, but numerous polychromatophilic cells, representing a stress erythropoiesis, can result in mild macrocytosis. Even more immature normoblasts that have not yet shed their nuclei (nucleated RBC) can be detected in severe anemias, especially in chronic severe hemolytic anemias. Macrocytosis, usually with an MCV of 100 to 110, but typically without anemia, is present in about 60% of alcoholics. The cause may be deficiency of folic acid or vitamin B12 due to poor nutrition, but this anemia can also be a direct effect of ethanol byproducts (acetaldehyde) to depress hematopoiesis. In addition, there are a number of inherited dyserythropoietic anemias or marrow failure states, which are characterized by macrocytosis, such as Fanconi syndrome, dyskeratosis congenita, or congenital dyserythropoietic anemia.
NORMOCYTIC ANEMIAS
Normocytic anemias have many diverse causes. In response to acute hemorrhage or hemolysis, the bone marrow responds maximally by increasing RBC production and releasing young erythrocytes prematurely. However, marrow erythropoiesis may be inadequate in normocytic anemias due to intrinsic bone marrow disease, insufficient iron availability, or inadequate erythropoietin effect. Reasons for anemia resulting from poor bone marrow response include (1) diminished numbers of erythroid precursors, as in aplastic anemia or following irradiation or cancer chemotherapy; (2) infiltration of the marrow by fibrosis, leukemia, cancer, or tuberculosis; and (3) myelodysplastic disorders, in which abnormalities in RBC maturation result in excessive RBC death in the marrow, i.e., ineffective erythropoiesis. Insufficient iron availability results from either absence of iron (iron deficiency) or from poor iron utilization (such as during inflammatory states). Low erythropoietin levels can result from (1) impaired renal production because of renal disease; (2) reduced erythropoietin target effect, as in endocrine disorders such as hypothyroidism and hypogonadism or antibodies to erythropoietin; or (3) interference with erythropoietin production or with its bone marrow effects, caused by inflammatory cytokines, contributing to the pathogenesis of the ACD.20 Rarer causes are pure red cell aplasia and aplastic anemia.
Anemia of Chronic Renal Disease
Anemia typically occurs with chronic renal disease only after the creatinine clearance decreases below 40 mL/min, which corresponds to a serum creatinine of about 2.5 mg/mL. Studies have shown that anemia of chronic renal disease can be more accurately assessed with cystatin C compared to creatinine. The anemia tends to worsen as the renal function decreases, but it usually stabilizes at a hematocrit of 15% to 30%. The cause of the kidney disease is not usually important in determining the severity of anemia, but anemia is typically less severe with polycystic kidney disease possibly due to erythropoietin production by the renal cyst epithelial cells. Several factors contribute to the anemia of chronic renal disease, the most important, however, being inadequate renal production of erythropoietin, a glycoprotein hormone synthesized in the kidney and responsible for the proliferation, maturation, and differentiation of erythrocytes in the bone marrow.21 Erythropoietin is also an erythroblast survival factor. In addition, red cell survival is shortened in uremia, and various toxins ordinarily excreted by the kidney accumulate in the serum and appear to depress erythropoiesis. Replacement by recombinant erythropoietin or erythropoietin-stimulating agents has become central to the management of anemia in chronic renal disease.22,23
The anemia is normochromic, normocytic, and most RBCs are unremarkable on the peripheral smear. Burr cells (echinocytes), however, may form via unknown mechanisms, and sometimes schistocytes appear. Both burr cells and schistocytes have shortened survival.
Anemia of Endocrine Disorders
Anemia, usually normocytic, occurs in several endocrine disorders. About 30% of patients with hypothyroidism have anemia, and about one-third of these are macrocytic. The anemia, usually mild, seems to relate to the hormone deficiency itself, and its severity is related to the duration and degree of hypothyroidism. Approximately 10% to 25% of patients with hyperthyroidism, usually with severe, prolonged disease, are anemic. The mechanism is uncertain.
Most patients with adrenal insufficiency have anemia, usually normocytic, normochromic. In those with autoimmune causes, pernicious anemia, producing a macrocytic anemia, is present in about 10%. Androgen deficiency also is a cause of normochromic, normocytic anemia. Hypopituitarism causes anemia through deficiencies of the previously mentioned thyroid, adrenal, and androgenic hormones.
A small number of patients with hyperparathyroidism have a normocytic, normochromic anemia, with bone marrow examinations typically demonstrating fibrosis. The increased parathyroid hormone may also decrease erythropoiesis.
Anemia of Chronic Disease
ACD is quite common with infections, noninfectious inflammatory states that last more than 1 or 2 months, or
malignancy.20 In about 25% of cases of ACD, anemia may be associated with congestive heart failure, hypertension, or diabetes mellitus. The anemia is mild to moderate, usually with a hemoglobin of about 10 mg/dL, but, in about 20% of patients, it can be more severe. The RBCs are typically normocytic, normochromic, but in about 30% they are mildly microcytic (usually 70-79 fL), and in about 50% they are hypochromic (MCHC 26-32). On peripheral smear, the RBC may display mild poikilocytosis and anisocytosis, but markedly small and thin cells, often seen in iron deficiency, are absent.
malignancy.20 In about 25% of cases of ACD, anemia may be associated with congestive heart failure, hypertension, or diabetes mellitus. The anemia is mild to moderate, usually with a hemoglobin of about 10 mg/dL, but, in about 20% of patients, it can be more severe. The RBCs are typically normocytic, normochromic, but in about 30% they are mildly microcytic (usually 70-79 fL), and in about 50% they are hypochromic (MCHC 26-32). On peripheral smear, the RBC may display mild poikilocytosis and anisocytosis, but markedly small and thin cells, often seen in iron deficiency, are absent.
In ACD, both serum iron and iron-binding capacity are low, unlike iron deficiency in which low serum iron and elevated iron-binding capacity coexist. As in iron deficiency, the iron saturation may be below 10%. The serum ferritin, which is characteristically less than 15 µg/L in iron deficiency, is at least 100 µg/L in ACD and usually much higher unless there is coexisting iron deficiency. Circulating transferrin receptor levels are normal in ACD, but elevated in iron deficiency. Bone marrow iron is present in ACD, and a trial of oral iron therapy does not correct the anemia.
The factors linking different anemias of chronic disease are inflammatory cytokines that negatively affect erythropoiesis, in particular interferon and interleukin-6.24,25 They impair the proliferation and differentiation of erythroid precursors, diminish erythropoietin production, and decrease the bone marrow response to erythropoietin. Cytokines affect iron metabolism by increasing iron retention in the bone marrow and in macrophages by stimulating hepcidin synthesis, thus decreasing iron availability for production of hemoglobin in maturing erythroid precursors. In addition, RBC lifespan is moderately decreased in ACD.
Pure Red Cell Aplasia
In this rare disorder, a normocytic anemia with diminished reticulocytes (<1%) and absence of polychromasia on the peripheral blood smear is accompanied by a marked diminution to absence of erythroblasts in the bone marrow (<0.5% of the marrow differential count), despite the presence of normal numbers of megakaryocytes and myeloid precursors and normal overall marrow cellularity. Pure red cell aplasia may develop without apparent cause or be associated with a wide variety of systemic diseases. It may be associated with viral infections such as parvovirus B19, in which case giant erythroblasts containing viral inclusions may be seen in the bone marrow. Pure red cell aplasia is also associated with numerous conditions characterized by disturbed immunity: in about 5% of patients with thymoma (accounting for approximately 10% of cases of pure red cell aplasia), hematologic malignancies, especially chronic lymphocytic and large granular lymphocytic leukemias; rheumatologic diseases (such as Sjögren syndrome and systemic lupus erythematosus), and even solid tumors. Numerous medications have been implicated, including phenytoin, azathioprine, and isoniazid. Sometimes, pure red cell aplasia occurs during pregnancy without any apparent explanation and typically disappears following delivery. In many patients, no cause is found but an immunoglobulin G (IgG) that inhibits erythropoiesis is present in the serum.
Aplastic Anemia
In aplastic anemia, pancytopenia in the peripheral blood and hypocellularity of the bone marrow accompany the anemia. Clinical presentations usually are for anemia or bleeding; infections are less common until the late stages. Certain types, such as Fanconi anemia, are hereditary, whereas some acquired aplastic anemias may have identifiable causes, such as exposure to medications, (chloramphenicol) or chemicals (benzene), or infections with certain viruses (hepatitis). Aplastic anemia may develop in patients with PNH or in other bone marrow failure states (e.g., dyskeratosis congenita) or as a complication of certain rheumatic diseases, such as eosinophilic fasciitis, systemic lupus erythematosus, or Sjögren syndrome. In the hemophagocytic syndrome, most commonly associated with viral infections or certain malignancies, pancytopenia, fever, hepatosplenomegaly, and lymph node enlargement occur, and the bone marrow, often hypocellular, shows macrophages ingesting erythrocytes.
Acquired aplastic anemia in the majority of cases appears to result from an immunologic reaction against an unknown target that damages bone marrow stem cells. The use of intensive immunosuppressive therapy during the past 30 years has resulted in marked improvement in survival,26 which has further improved since the introduction of the thrombopoietin agonist eltrombopag which has been able to stimulate growth of megakaryocytic and erythroid precursors.27 Clonal hematopoiesis is common in aplastic anemia (documented in 30%-40% of cases) as is acquired mutations associated with MDS/acute myeloid leukemia, which, as well as PNH occur in increased frequency in patients with preceding aplastic anemia.28
HEMOLYTIC ANEMIAS
Hemolytic anemias are characterized by increased RBC destruction, so that the average lifespan of RBC may be shortened from the normal 120 days to a few days or even hours. Hemolytic anemias are classified either by site of destruction (extravascular vs. intravascular) or by mechanism (destruction that is intrinsic or extrinsic to the RBC). In extravascular hemolysis, which is common, the red cells are destroyed by macrophages in the spleen, liver, or bone marrow, whereas in intravascular hemolysis, which is rare, RBCs are destroyed within the bloodstream. The differential diagnosis includes (1) an abnormal RBC environment because of infections, medications, or immunologic processes; (2) RBC membrane abnormalities; (3) RBC metabolic defects (including glucose-6-phosphate dehydrogenase [G6PDH] deficiency and pyruvate kinase deficiency); and (4) abnormalities in hemoglobin structure or synthesis.
During intravascular hemolysis, released hemoglobin first binds to haptoglobin, and then to hemopexin. When free hemoglobin exceeds the binding capacity of haptoglobin, it turns the plasma pink. Free hemoglobin is filtered in the kidneys, and the urine may appear red. The dipstick testing of urine for blood is positive, but the urine microscopy is negative for RBC. This discrepancy is an important distinction between hemoglobinuria and hematuria. The renal tubular epithelium cells take up some of the hemoglobin, transforming it into hemosiderin, which is visible on iron stains of cells in the urinary sediment. Evidence of recent or ongoing intravascular hemolysis, thus, includes a reduced serum haptoglobin level (which also occurs in extravascular hemolysis), the presence of plasma or urine hemoglobin, and detection of hemosiderin in renal tubular cells in the urinary sediment. Intravascular hemolysis is typically severe and has disparate causes, including (1) mechanical damage to the red cell caused by fibrin strands within the vessel lumen during disseminated intravascular coagulation, vasculitis, or thrombotic thrombocytopenic purpura; (2) physical trauma from red cells passing through dysfunctional prosthetic valves or through small vessels of the feet during hard marching; (3) thermal injury from burns; (4) RBC infection by malaria parasites or toxic exposure to snake venoms; (5) complement-mediated damage to erythrocytes caused by cold agglutinins, incompatible red cell transfusions, and PNH; and (6) hemolytic uremic syndrome from shiga toxin-producing microorganisms like Escherichia coli.
The other major classification of hemolytic anemias differentiates disorders intrinsic to the red cell, which are typically hereditary, and those extrinsic to the red cell, usually acquired. The intrinsic disorders include abnormal hemoglobins, enzyme defects, and membrane abnormalities. The extrinsic disorders are immunologic disorders, mechanical factors, infections and toxins, liver disease (spur cell anemia), and hypersplenism.
Immunologic hemolytic anemias are a collection of relatively rare disorders all characterized by the production of autoantibodies that target RBCs for destruction. Immune hemolytic anemia can arise spontaneously, be associated with disturbed immunity (e.g., in immunodeficiency state, in chronic lymphocytic leukemia, in lupus erythematosus, or after bone marrow transplantation), or follow transfusion with incompatible or incompletely matched blood products. The three major categories of autoimmune hemolytic anemia are (1) warm antibody (most cases and typically involves an IgG antibody), (2) cold antibody (antibodies that react at temperatures below body temperature and are usually IgM), and (3) mixed cold and warm type. Drugs such as penicillin and cephalosporins can evoke a warm antibody type autoimmune hemolytic anemia by functioning as haptens that attach to the RBC membrane.29 The direct antiglobulin test (or Coombs test) is used to detect autoimmune hemolytic anemias; in this test, the patient’s RBCs are incubated with antibodies to human IgG and complement component 3 (C3).30 If autoantibodies are present, they will have coated the patient’s RBCs and the addition of the anti-IgG and anti-C3 will result in RBC agglutination. The indirect Coombs test is used to detect the presence of anti-RBC antibodies in the patient’s serum, even when the sensitized RBCs have all been destroyed.
The Coombs test is negative in cases of PNH (where destruction of the RBCs is due to deficiency of complement inhibitors on them, occurs sporadically and often at night, but is not immunologically mediated).31
Diagnostic abnormalities in RBC morphology associated with hemolytic anemias may be detected on peripheral blood smear, such as sickle cells, bite cells, schistocytes, and spherocytes. The presence of relatively uniform spherocytes may suggest an underlying RBC membrane abnormality such as hereditary spherocytosis (in which abnormal cytoskeletal proteins result in spherical rather than discoid RBC forms), hereditary elliptocytosis (an autosomal dominant genetic defect characterized by a destabilized cytoskeleton and thus an elliptical shape), and hereditary stomatocytoses (autosomal dominant genetic defect impairing the ability of the RBC to maintain proper cationic balance).32 Other findings may include red cell agglutination denoting the presence of a cold antibody, organisms such as malarial parasites, and ingestion of erythrocytes by macrophages (erythrophagocytosis), which especially suggests immune hemolytic anemias, but also can occur with infections or toxins. The peripheral smear in immune hemolytic anemias typically shows spherocytes of varied size, substantial polychromatophilia caused by the increased release of immature red cells from the bone marrow, and if anemia is severe, nucleated RBCs. The reticulocyte index is above 3 and the absolute reticulocyte count is over 100,000/mm3. The indirect bilirubin is elevated and represents over 80% of the total bilirubin. The serum LDH is often increased and the serum haptoglobin low or absent. Coombs tests detect immunoglobulin and/or complement on the red cell surface, indicating an immune hemolysis. For suspected intravascular hemolysis, urine and plasma hemoglobin measurements are useful, and if hemolysis is chronic, iron stains of urinary sediment may be positive. A hemoglobin electrophoresis is indicated for suspected hemoglobinopathies.
Microangiopathic hemolytic anemias are caused by intravascular fibrin formation or by mechanical valve dysfunction and will be discussed in the chapter on hemostasis.33
The most frequent hemolytic anemia caused by an intrinsic RBC enzyme defect is G6PDH deficiency.34 This involves a mutation in the G6PDH gene, which is active in the pentose monophosphate shunt. The pentose monophosphate shunt is the sole source of nicotinamide adenine dinucleotide phosphate (NADPH), the main antioxidant in RBCs. NADPH is essential for preventing oxidative damage to the RBCs and lack of this important reductant can lead to increased RBC hemolysis, especially in situations that exacerbate oxidative damage as treatment with certain drugs (antimalarials, methylene blue, and benzocaine) or consumption of foods like fava beans. G6PDH deficiency is an X-linked disorder
most common in Africa, the Mediterranean and Southeast Asia, and affects over 400 million persons worldwide.
most common in Africa, the Mediterranean and Southeast Asia, and affects over 400 million persons worldwide.
HEMOGLOBINOPATHIES AND THALASSEMIAS
Hemoglobin A (Hb A), which constitutes more than 90% of the adult hemoglobin, consists of four polypeptide chains, two α and two β (α2β2). Hemoglobin A2, composed of two α and two δ (α2δ2), is present in small quantities. Hemoglobin F (α2γ2), the main hemoglobin during fetal life, comprises two α and two γ chains, and usually comprises <1% of the normal adult’s hemoglobin. As β-chain production begins before birth, the level of Hb F represents about 75% of the hemoglobin at birth. By 6 months of age, Hb F has normally diminished to 5%. Defects in globin gene synthesis can result in two distinct categories of mutations: qualitative defects (the hemoglobinopathies) and quantitative defects (thalassemias).35 Qualitative defects involve globin gene mutations which lead to altered hemoglobin structure. It is worth noting that the vast majority of hemoglobin variants are clinically silent. Major clinically important abnormal hemoglobins include Hb S, Hb C-Harlem, and Hb C. Hb S and the rare Hb C-Harlem both have an essential glutamic acid residue at position 6 mutated to a valine (Hb C-Harlem has an additional mutation). This single amino acid substitution in Hb S arising from a missense mutation drastically alters the behavior of the Hb, especially at low oxygen conditions. The presence of valine results in the formation of Hb S polymers within the RBCs that cause the RBCs to deform into the characteristic sickle shape.
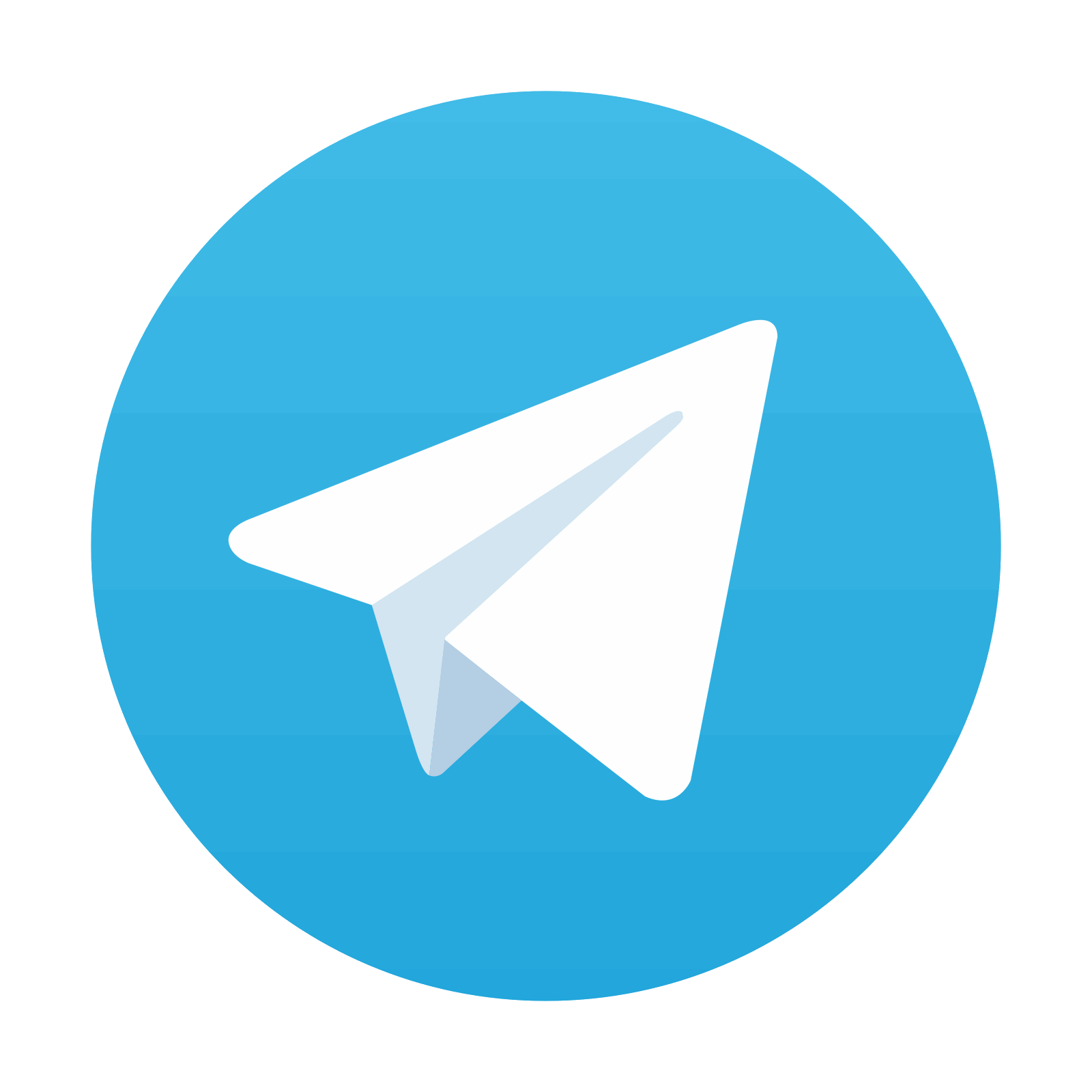
Stay updated, free articles. Join our Telegram channel

Full access? Get Clinical Tree
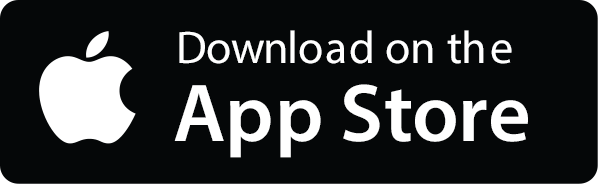
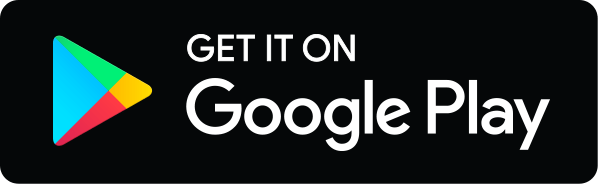