SPERMATOGENESIS
The sequence of cytologic changes that produce spermatozoa from spermatogonia is called spermatogenesis. It can be subdivided
into three major phases: the replication of stem cells, meiosis, and spermiogenesis.
into three major phases: the replication of stem cells, meiosis, and spermiogenesis.
REPLICATION OF STEM CELLS
The replication of stem cells commences in fetal life with the migration of the primordial germ cells into the mesenchyme of the gonadal ridge. There is evidence that this migration is controlled by stem cell factor and its receptor c-kit.3 After a period of prenatal mitotic division, the gonocytes, which populate the seminiferous cords at birth, remain quiescent until immediately before puberty, when they divide by mitosis to form the spermatogonial population.4 The types of spermatogonia (Fig. 113-2) that can be characterized cytologically vary with each species. However, a feature common to all is the division of the stem cell population to provide two pools of cells, one that moves through the subsequent steps of spermatogenesis and the other that retains its stem cell function.5,6 In the human male, three types of spermatogonia are recognized classically: the A dark, thought to represent the most primitive; the A pale; and the B spermatogonia. They lie adjacent to the basement membrane of the tubule, interspersed with the basal aspects of the Sertoli cells. During cell division, the spermatogonia do not complete cytokinesis and remain linked by intercellular bridges.7Populations of spermatogonia that are injected into tubules devoid of germ cells will partially restore spermatogenesis.8 These studies will provide the experimental basis for further evaluation of the nature and control of the stem cells in the testis.
MEIOSIS
Responding to unknown signals, groups of type B spermatogonia begin meiosis, which involves two cell divisions. The spermatogonia lose their contact with the basement membrane of the tubule and are then called primary spermatocytes.9 During the prophase of the first division (see Chap. 90), the primary spermatocytes, which have already replicated their DNA and contain twice the diploid content, undergo a series of characteristic nuclear changes consistent with the appearance and pairing of homologous chromosomes (Fig. 113-3). During the leptotene stage, the chromosomes appear as single, randomly coiled threads, which thicken and commence pairing during zygotene. During pachytene, the chromosomes appear as condensed, closely paired structures, which begin to repel each other during diplotene. Diakinesis is associated with further repulsion of the pairs of chromosomes, which each consist of a pair of daughter chromatids. During the metaphase of this first division, each member of the pair of homologous chromosomes moves to each daughter cell, reducing the number of chromosomes to the diploid number; however, because each chromosome is composed of two chromatids, the DNA content of a diploid cell is retained. Incomplete cytokinesis causes the formation of a pair of joined secondary spermatocytes from each primary spermatocyte.
During the second division, the 23 chromosomes, each comprising a pair of chromatids, attach to the spindle, and the chromatids separate. This yields a cell, a spermatid, containing the haploid DNA and chromosomal complement.
SPERMIOGENESIS
Cell division stops after the formation of the spermatids. However, a dramatic metamorphosis, spermiogenesis, transforms a conventional cell into a highly specialized cell with the capability of flagellar-derived motility (Fig. 113-4 and Fig. 113-5). Little is known of the mechanisms by which these dramatic cytologic changes are controlled. The developmental phases are termed the Sa1, Sb1, Sb2, Sc2, Sd1, and Sd2 stages according to Clermont, but many of the details can be determined only by electron microscopy.9,10 and 11 With the increasing use of spermatids extracted directly from the testis by biopsy, the specific features that characterize each of the above stages become crucial in identifying
the nature of the cell type injected into the cytoplasm of the oocyte to achieve fertilization. The changes can be subdivided into nuclear, acrosome formation, flagellar development, redistribution of cytoplasm, and spermiation.
the nature of the cell type injected into the cytoplasm of the oocyte to achieve fertilization. The changes can be subdivided into nuclear, acrosome formation, flagellar development, redistribution of cytoplasm, and spermiation.
![]() FIGURE 113-4. Initial cytologic changes during spermiogenesis are shown. See Figure 113-7 for explanation of Sa, Sb1, and Sb2. (From de Kretser DM. The light and electron microscope anatomy of the normal human testis. In: Santen RJ, Swerdloff RS, eds. Male sexual dysfunction: diagnosis and management of hypogonadism, infertility and impotence. New York: Marcel Dekker Inc, 1986:3.) |
![]() FIGURE 113-5. Subsequent cytologic changes during spermiogenesis. (See also Fig. 113-7.) (From de Kretser DM. The light and electron microscope anatomy of the normal human testis. In: Santen RJ, Swerdloff RS, eds. Male sexual dysfunction: diagnosis and management of hypogonadism, infertility and impotence. New York: Marcel Dekker Inc, 1986:3.) |
NUCLEAR CHANGES
During the Sa1 and Sb1 stages, the nucleus, which ultimately forms the head of the sperm, remains centrally placed, but it is subsequently displaced peripherally, coming into apposition with the cell membrane, separated only by the acrosomal cap. There is also a progressive decrease in nuclear volume associated with chromatin condensation, causing the development of resistance by the DNA to degradation by the enzyme DNAase.
ACROSOME FORMATION
During the Sa1 and Sb1 stages, the Golgi complex of the spermatid produces several large vacuoles, which are applied to one pole of the nucleus. These vacuoles form a cap-like structure, the acrosome, which contains substances for penetration of the ovum during fertilization.11a Sperm that do not contain an acrosome cannot fertilize the ovum; because of the globular shape of the sperm head, the condition is known as globozoospermia. The Golgi complex subsequently migrates to the abacrosomal pole of the spermatid and is lost in the residual cytoplasm.
FLAGELLAR DEVELOPMENT
The initial development of the tail occurs from the pair of centrioles located near the Golgi complex of the Sa1 spermatid. The microtubular structure, comprising nine peripheral doublets surrounding a pair of single microtubules, grows out from the distal centriole and forms the axoneme or core of the tail. The developing axoneme lodges in a facet at the abacrosomal pole of the nucleus by a complex articulation called the neck of the spermatid. Initially, the axoneme distal to the neck is surrounded by the cell membrane, but several specializations develop. Immediately adjacent to the outer nine doublets, a set of nine electron-dense fibers develop, which are connected cranially to the neck but distally taper to disappear eventually. A pair of these dense fibers, which lie diametrically opposite to each other, persist distally and become surrounded by a collection of microtubules. These microtubules form the precursors for solid electron-dense fibers, or ribs, which run transversely around the axoneme, joining the pair of longitudinal, dense fibers. The region of the tail surrounded by these ribs is the principal piece. The region between the principal piece and the neck is the last segment to become organized. Some of the genes, which encode the proteins comprising the outer dense fibers and the fibrous sheath, have been identified; these will provide the basis for future studies on the formation and function of the components.12,13,14 and 15 Relatively late in spermiogenesis, between the Sd1 and Sd2 stages, the mitochondria that lie in the peripheral regions of the cell coalesce to form several helical arrays around the axoneme, forming the midpiece of the sperm.
The axoneme is identical to the core structure of cilia throughout the plant and animal kingdoms. The two centrally placed, single microtubules appear to be connected to the nine
peripheral doublets by a series of radial spokes (Fig. 113-6). One of each pair of doublets, termed subfiber A, is smaller and more electron dense; it sends a pair of hook-like extensions, or dynein arms, toward the adjacent doublet. They represent condensations of the protein, dynein, which has adenosine triphosphatase activity and is vital to the generation of flagellar motility; the absence of dynein arms is associated with immobility of sperm and cilia.16,17
peripheral doublets by a series of radial spokes (Fig. 113-6). One of each pair of doublets, termed subfiber A, is smaller and more electron dense; it sends a pair of hook-like extensions, or dynein arms, toward the adjacent doublet. They represent condensations of the protein, dynein, which has adenosine triphosphatase activity and is vital to the generation of flagellar motility; the absence of dynein arms is associated with immobility of sperm and cilia.16,17
REDISTRIBUTION OF CYTOPLASM
Associated with the eccentric placement of the nucleus is a significant repositioning of the cytoplasm and organelles. This probably is caused by a palisade of microtubules, the manchette, which extends as a cylindrical collection from the head, in the region where the acrosome ends, to the caudal pole of the spermatid. Ultimately, most of this cytoplasm is shed by the spermatid and effectively appears to be pulled off by processes of Sertoli cell cytoplasm, which invaginate into pouches developing in the spermatid cytoplasm.10 This cytoplasmic remnant, the residual body, is phagocytosed and degraded by the adjacent Sertoli cells.
SPERMIATION
The release of spermatids at the Sd2 stage occurs in association with the loss of the residual cytoplasm.
SPERMATOGENIC CYCLE
Careful and extensive cytologic studies have demonstrated a characteristic sequence of cell associations through which the seminiferous epithelium passes and that constitute the cycle of the seminiferous epithelium.6 In the rat, this consists of 14 cell associations, each of which may extend over several millimeters of tubule. However, in the human, six cell associations or stages have been identified, and each may occupy only 25% to 33% of a cross section of a seminiferous tubule9 (Fig. 113-7). Studies in which dividing cells are labeled by tritiated thymidine have shown that the time taken for the daughter cells of spermatogonial divisions to mature to Sd2 spermatids released from the seminiferous tubule is 64 ± 6 days or 4.5 cycles of the human seminiferous cycle.18 The time for spermatogenesis is unique for each species and represents a biologic constant. The germ cells either pass through these stages at a specified speed or degenerate.
It is uncertain whether these time constraints are an innate function of each cell or whether they are imposed by other controlling factors. Coordination of the spermatogenic process may be achieved by the unusual organization of the seminiferous epithelium, wherein the germ cells remain joined by intercellular bridges.7 Additionally, coordination may be achieved by the Sertoli cells, whose arborizing branches maintain contact with many germ cell stages around the radial axis of the seminiferous tubules.
SERTOLI CELLS
Sertoli Cell Cytology.
The general features of the Sertoli cell are similar in most mammalian species.19,20 The cells extend from the basement membrane of the tubule to the luminal surface of the epithelium, and, although the base of the cell is clearly identifiable, the central portions cannot be distinguished by light microscopy. This results from the formation of many thin cytoplasmic prolongations that extend in an arborizing network around the germ cells undergoing spermatogenesis.
Electron microscopy has demonstrated that these processes are always surrounded by the cell membrane of the Sertoli cell.
Electron microscopy has demonstrated that these processes are always surrounded by the cell membrane of the Sertoli cell.
In the Sertoli cell, the cytoplasmic organelles exhibit some degree of polarity. The basal aspects, which abut the basement membrane of the tubule, interspersed between spermatogonia, often show collections of mitochondria. The nucleus is pleomorphic, is aligned perpendicular to the basal lamina, and contains a prominent nucleolus. The nucleolus is a feature of the postpubertal Sertoli cell, probably related to the follicle-stimulating hormone (FSH)–induced maturation of the protein synthetic capacity of the mature Sertoli cell.21,22
In the perinuclear region, small collections of rough endoplasmic cisternae and Golgi membranes can be seen. Significant numbers of lipid inclusions, often surrounded by smooth endoplasmic reticulum, are found adjacent to the nucleus; in some species, these inclusions exhibit significant changes in number with the stages of the seminiferous cycle. Lysosomes, lipofuscin pigment, and residual bodies, which are the phagocytosed cytoplasmic remnants of the spermatids, may be seen deep within the Sertoli cell cytoplasm. The shape of the Sertoli cell is probably maintained by the cytoskeleton, consisting of a perinuclear array of fine filaments and microtubules that often extend into the smaller processes of cytoplasm surrounding the germ cells.
Inter–Sertoli Cell Junctions.
One of the characteristic features of the Sertoli cell is the specialized inter–Sertoli cell junction, which occurs where adjacent Sertoli cells abut. These usually commence at a level in the epithelium just luminal to the basal row of the spermatogonia and extend centrally. This unique cell junction consists of small occluding junctions, representing points of fusion of the cell membranes that obliterate the intercellular space. Adjacent to these points of occlusion, smooth-membraned cisternae run parallel to the cell membrane and demarcate a narrow band of cytoplasm containing bundles of fine filaments.23 The effect of these complexes is to prevent transport by way of the intercellular space to the centrally placed germ cells.23 The inter–Sertoli cell junctions divide the seminiferous epithelium into two compartments: a basal one containing the spermatogonia and preleptotene primary spermatocytes and an adluminal one containing the subsequent stages of germ cell maturation. The cell junctions represent the site of the blood–testis barrier; by preventing intercellular transport, they create a highly selective permeability barrier based on transport systems within the Sertoli cell.24 These cell junctions and the blood–testis barrier are absent in the immature testis but develop during pubertal maturation.21,25 The resultant development of the cell junctions and barrier coincides with the onset of meiosis and seminiferous fluid secretion.22,26 These cell junctions are not permanent structures since they must disassemble and reform basally as preleptotene spermatocytes lose their attachment to the basement membrane and move centrally.
Sertoli Cell Function.
There has been a great expansion in the knowledge of Sertoli cell function.27 Because of the intimate relationship of the Sertoli cells to germ cells, the function of these cells is probably crucial for the successful completion of spermatogenesis. The concept that the number of Sertoli cells determines the spermatogenic output of the testis has gained considerable support, and clearly, the Sertoli cell is critically important for the transport of substances into the seminiferous tubule. Numerous examples indicate that the Sertoli cells are essential for the metabolic activities of the germ cells: For example, the germ cells, which are unable to metabolize glucose, are provided with lactate by the Sertoli cells.28 Key roles of the Sertoli cells are discussed later.
Sertoli Cell Replication.
Studies in the rat demonstrate that the Sertoli cell population divides by mitosis in fetal and post-natal life until day 15 but remains stable thereafter.29 Neonatal hypothyroidism in the rat can extend the period of Sertoli cell replication, and in adult life the increased numbers of Sertoli cells lead to a marked increase in sperm output.30 These data strongly suggest that the number of Sertoli cells in the testis is a major factor in controlling the spermatogenic potential of the testis. Use of this model has also shown that the functional maturation of the Sertoli cells is delayed during hypothyroidism31and that there is a marked delay in the process of spermatogenesis.32 The control of Sertoli cell proliferation involves FSH, thyroid hormones, and growth factors such as activin A.33
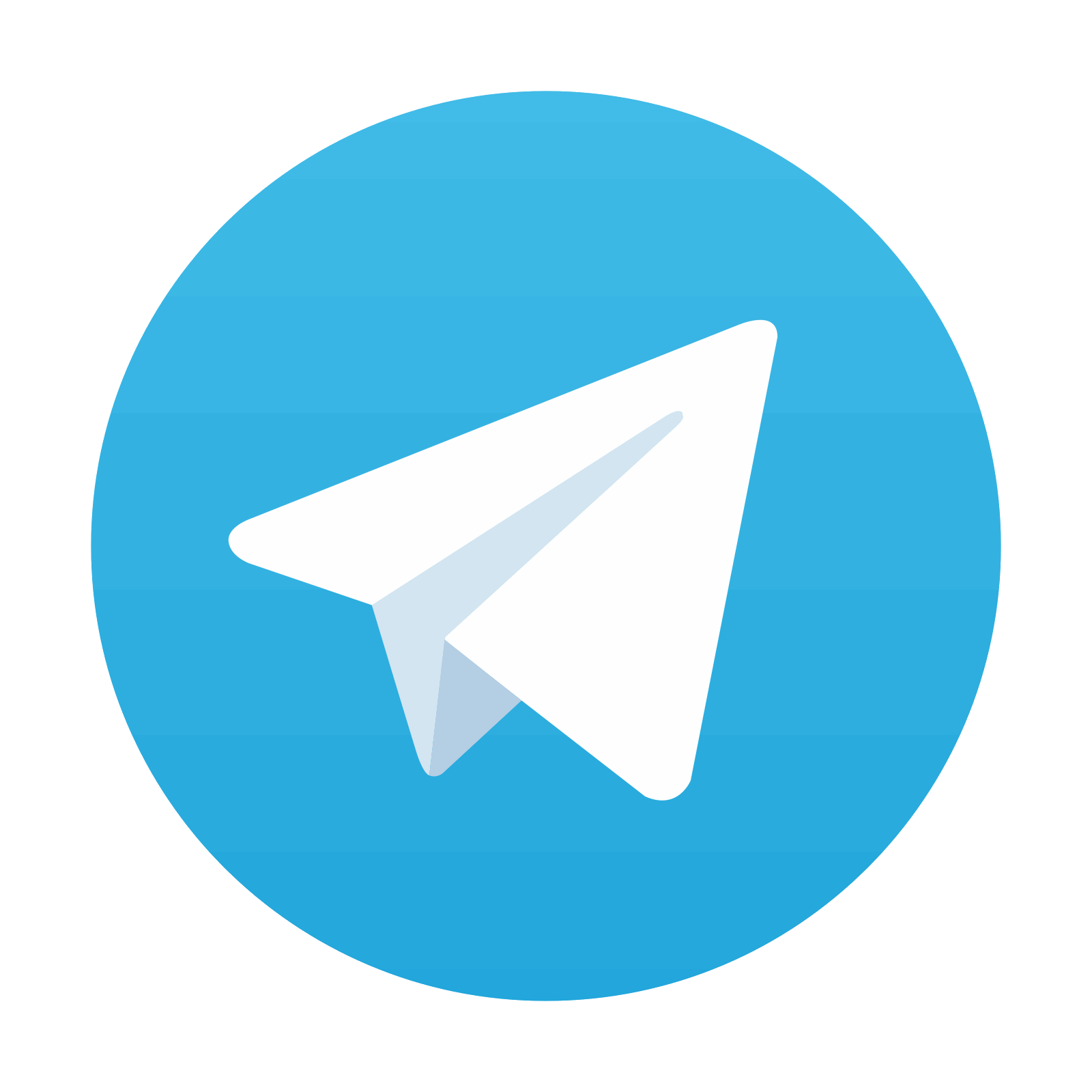
Stay updated, free articles. Join our Telegram channel

Full access? Get Clinical Tree
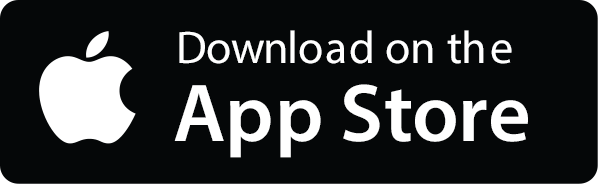
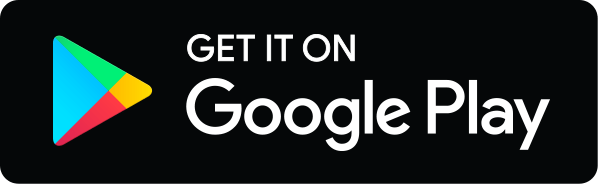
