Introduction
Since 1900, the percentage of Americans over the age of 65 years has more than tripled (4.1% in 1900 to over 12% in 2000) and their number has increased over eleven times (from 3.1 million to 34.9 million).1 Given the fact that the ability to perceive odours and tastes decreases markedly with age,2 it is not surprising that increasing numbers of elderly patients are seeking medical help for their chemosensory problem. Indeed, over half the population between the ages of 65 and 80 years, and over three-quarters beyond 80 years, have significant olfactory loss. The implications of such age-related chemosensory losses are far-reaching. Aside from being unable to appreciate fragrances, the taste of food, and the freshness of spring and the seashore, elderly persons suffering from chemosensory disorders are compromised in their ability to detect fire, leaking natural gas, toxic fumes and spoiled food. Many become depressed, and a disproportionate number die in accidental gas poisonings.3 Others lose their lives or are severely burned in the hundreds of butane and propane gas explosions that occur each year.
It is now well documented that olfactory dysfunction is among the first, if not the first, clinical signs of Alzheimer’s disease and sporadic Parkinson’s disease (for review, see Hawkes and Doty4). Although, as described later in this chapter, smell loss has multiple determinants and is not always a harbinger for such diseases, it is incumbent upon the physician to be aware of this association. Given the dietary and safety consequences of chemosensory disturbances, it is also incumbent upon the physician to employ the most modern means available to evaluate, counsel and treat patients with chemosensory disturbances whenever possible.
This chapter provides the gerontologist with an up-to-date overview of the nature and cause of age-related chemosensory disturbances, means for evaluating such disturbances, and approaches useful for counselling patients and treating the underlying dysfunction.
Characterization of Chemosensory Problems
The general term for inability to smell is anosmia, and for lessened smell function hyposmia. The corresponding terms for taste are ageusia and hypogeusia. In the older medical literature, anosmia is sometimes referred to as olfactory anaesthesia or anosphrasia. In some nosological schemes, anosmia and hyposmia are classified under the general term dysosmia (distorted smell function), whereas ageusia and hypogeusia are classified under the term dysgeusia (distorted taste function). In this scheme, dysosmia includes forms of dysfunction in addition to anosmia, such as distorted smell sensations (parosmia, cacosmia) and smell hallucinations (phantosmia). Dysgeusia similarly includes both ageusia and distortions in taste function, such as strong salty or sour sensations in the absence of appropriate stimulation. Today, however, it is more common that anosmia, ageusia, dysosmia and dysgeusia are classified separately from one another, with the first two terms signifying losses, and the second two distortions, of smell and taste sensations, respectively.
Anatomy of The Olfactory System
To be sensed, odorants must enter the nose and reach specialized receptors within the olfactory neuroepithelium, a patch of tissue a few square centimetres in size that lines the upper recesses of the nasal vault, including the cribriform plate and sectors of the nasal septum, middle turbinate and superior turbinate5 (Figure 88.1). When activated, the odorant receptors open or close (e.g. via second-messenger systems) membrane channels on the cilia, resulting in a flux of ions and an alteration of the cell’s resting potential that ultimate leads to an axonal action potential.6 cAMP is the primary second messenger involved in the transduction process. cAMP amplifies the signal coming from the receptors and facilitates the release of glutamate, the main neurotransmitter of olfactory receptor cells, into the synapse.
Figure 88.1 Schematic of the cellular organization of the human olfactory neuroepithelium. Not pictured are the microvillar cells, which are small goblet-shaped cells interspersed among the other cell types at the surface of the epithelium in a ratio to the mature receptor cells of 1:10. Reprinted from Gray’s Anatomy, Warwick R and Williams PL, Copyright 1973, with permission from Elsevier.
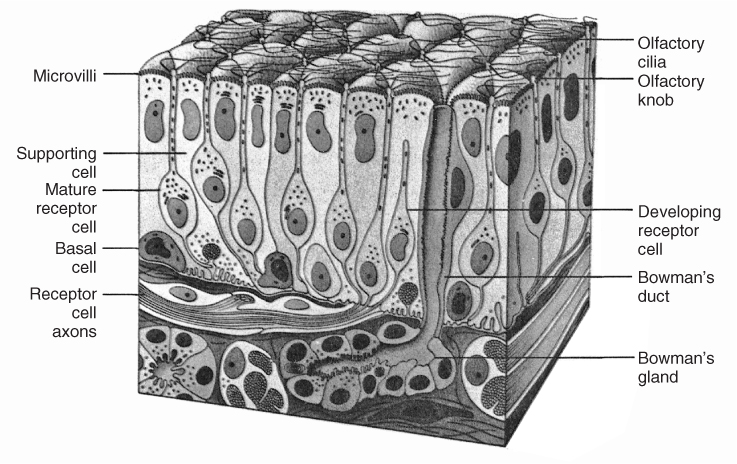
Odorant receptor genes, whose discovery by Buck and Axel in 1991 led to the Nobel Prize for Medicine or Physiology in 2004,7 represent the largest of all mammalian gene families, comprising nearly 3% of the more than 30 000 genes in the mouse and human genomes. Interestingly, only one type of receptor is expressed on the surface of the cilia of a given receptor cell, and odorants typically bind to more than one type of receptor. The olfactory receptor cells number 6–10 million in the adult human and are insulated from one another at the epithelial surface by sustentacular cells.5 A blanket of mucus, which contains a number of enzymes (e.g. cytochrome P450), covers the olfactory neuroepithelium and deactivates or filters materials that absorb into the mucus, including some odorants.8 This mucus also aids in protecting the epithelium from desiccation, heat and xenobiotic insult, and serves as a solvent and carrier for odorant binding proteins—proteins that facilitate the transport of some lipophilic molecules to the receptors through aqueous phases of the mucus.
The unmyelinated axons of the bipolar olfactory receptor cells collect into 15–20 fascicles (fila olfactoria) that collectively make up the olfactory nerve (cranial nerve (CN) I). These axons course through the cribriform plate and synapse within spherical masses of neuropile within the olfactory bulb termed glomeruli. Second order connections with the dendrites of mitral and tufted cells are made within these structures. The latter cells—the primary output cells of the olfactory bulb—project to the olfactory cortex, which includes the piriform, periamygdaloid and entorhinal cortices. These structures have extensive connections with the hippocampus, mediodorsal thalamus, hypothalamus and other brain regions, in addition to having efferent connections with cells within the olfactory bulb.4
Most odorants stimulate a broad range of receptor cells. Although limited sets of such cells respond to a given odorant, overlap is common and the pattern of neuronal activity across cells codes odour quality. Receptor cells that express the same receptor project to the same glomerulus, where information is further transformed. The second-order neurons—the mitral and tufted cells—send dendritic processes into the glomeruli, where they synapse with the axons of the incoming receptor cells. The axons of the mitral and tufted cell project, via the lateral olfactory tract, to the olfactory cortex, where further connections occur with structures in which perceptual elements of odours are formed; that is, perceived pleasantness and associations with environmental objects. It is noteworthy that the olfactory system differs from other sensory systems in sending projections first to the cortex rather than to the thalamus. It also is unique in the degree to which it exhibits plasticity—the olfactory receptor cells have the propensity to regenerate from stem cells within the basement membrane, and cells within the olfactory bulb, namely the periglomerular cells and the granule cells, are continuously repopulated by cells that migrate from periventricular regions along the rostral migratory stream. Because olfactory receptor cells directly project from the environment of the nasal cavity into the brain, they are a major conduit for viruses and a range of xenobiotic agents into the brain and may initiate neurodegenerative pathology in genetically susceptible individuals.9
In addition to the sensory innervation of the olfactory nerve, free nerve endings of the trigeminal nerve (CN V) are distributed throughout the nasal mucosa. The ophthalmic and maxillary divisions of CN V carry information regarding irritation, temperature and pungency. Sensations mediated by non-CN I nerves are those of the ‘common chemical sense’ and do not encode the qualitative perception of ‘odour’, per se.10 Although humans possess a rudimentary vomeronasal (Jacobson’s organ) pouch at the base of the nasal septum, the elements of this system are vestigial and humans lack an accessory olfactory bulb which would normally receive a projection from this structure. Despite fanfare to the contrary, it is questionable whether humans—indeed mammals in general—communicate by so-called pheromones.11
Anatomy of The Gustatory System
Taste receptor cells are located within taste buds on the tongue, soft palate, uvula, epiglottis, rostral oesophagus and mucous membranes of the laryngeal cartilages. Most lingual taste buds are found imbedded in the surface of protuberances termed papillae. Fungiform papillae are prevalent on the anterior tongue, circumvallate papillae within the chevron of the posterior tongue, and foliate papillae within the lateral margins of the medial tongue separating the anterior and posterior sectors12 (Figure 88.2).
Figure 88.2 (a) and (b) Schematic of the distribution of taste buds on the human tongue. Taste buds of the fungiform and foliate papillae are innervated by CN VII. Those of the circumvallate papillae are innervated by CN IX. CN V carries non-taste somatosensory sensations. See text for details. (c) Schematic of fine structure of taste bud. (1) and (2) are presumably supporting cells that secrete materials into the lumen of the bud; (3) is a sensory receptor cell; and (4) a basal cell from which other cell types arise. Image courtesy of RG Murray, 1973. Copyright RG Murray.
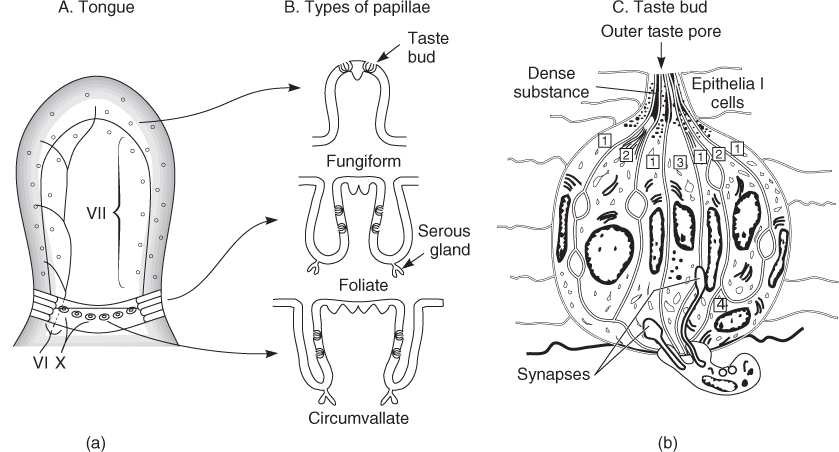
The sense of taste is supplied by three cranial nerves: the facial nerve (CN VII), the glossopharyngeal nerve (CN IX), and vagus nerve (CN X). As shown in Figure 88.2, the taste buds on the fungiform papillae are supplied by the chorda tympani branch of CN VII, whereas the taste buds on the other types of taste papillae are supplied by the lingual branch of CN IX. Although it is generally believed that the innervation of CN IX is limited to the posterior third of the tongue, recent studies suggest that it may project afferent fibres beyond this posterior boundary.13 Taste buds located on the soft palate send their projections centrally via the greater superficial petrosal branch of CN VII and those on the epiglottis, oesophagus and larynx transmit taste information by way of the superior laryngeal branch of CN X. As in the case of olfaction, trigeminal (CN V) free nerve endings, distributed throughout the oral cavity, mediate somatosensory sensations (e.g. pungency, burning, sharpness). All branches of the gustatory nerves enter the brainstem and terminate in the rostral part of the nucleus of the solitary tract. The subsequent projections are not thoroughly understood in humans; however, connections are made with the ventral posteriormedial thalamus and insular cortex. In rodents, fibres from the pons also travel to areas involved in feeding and autonomic regulation, including the lateral hypothalamus, central amygdala and stria terminalis.14
Clinical Tests of Olfactory and Gustatory Function
The physician of the past assessed the ability to smell by asking a patient to sniff vials containing one or two odorants such as coffee or tobacco, and to report whether or not an odour is perceived. The analogous taste test was to sprinkle grains of sugar or salt onto the tongue, and ask about the corresponding sensations. Unfortunately, such procedures are akin to testing vision by shining a flashlight into the eye, or audition by sounding a bull horn next to the ear. This problem is not corrected, in the case of olfaction, by having the patient attempt to identify the presented odorants, since without cuing even normal subjects have difficulty identifying most odorants. In the case of taste, non-solubilized tastants are often not recognized by patients whose mouths are dry, or who have little time to dissolve the tastants into saliva.
During the last 25 years remarkable progress has been made in the development of reliable, valid and clinically practical olfactory tests. Physicians and insurance carriers are now aware, more than ever, that objective chemosensory assessment is essential for (a) establishing the validity of a patient’s complaint, (b) characterizing the specific nature of a chemosensory problem, (c) accurately monitoring medical or surgical interventions, (d) detecting malingering, (e) counselling patients to help cope with their problem, and (f) assigning disability compensation. Importantly, accurate assessment decreases the costs of continuing treatment seeking on the part of patients, who are usually assumed to have a problem even in the absence of objective data.
It should be noted that patients have difficulty ascribing the degree of their olfactory or gustatory dysfunction unless total or near-total loss is apparent. In the case of taste, for example, questionnaire statements such as ‘I can detect salt in chips, pretzels, or salted nuts’, ‘I can detect sourness in vinegar, pickles, or lemon’, ‘I can detect sweetness in soda, cookies, or ice cream’, and ‘I can detect bitterness, in coffee, beer, or tonic water’ are relatively insensitive in detecting true cases of dysfunction. However, such questions are sensitive in detecting persons without such problems (i.e. they exhibit low positive but high negative predictive value).15
Several commercially available tests of olfactory function are now available, including tests of odour detection and identification (for review, see Doty, 2001).16 The most widely used of these tests (the University of Pennsylvania Smell Identification Test or UPSIT: commercially termed the Smell Identification TestTM, Sensonics, Inc., Haddon Hts, NJ) was developed at our centre and evaluates the ability of patients to identify, from sets of four descriptors, each of 40 ‘scratch and sniff’ odorants2 (Figure 88.3). The number of items correctly identified out of 40 serves as the test score. This measure is compared to norms based upon data from a large number of individuals sampled from the community at large and a percentile rank is determined, depending upon the age and gender of the patient. This test, which correlates strongly with traditional threshold tests, is amenable to self-administration and provides a means for detecting malingering. Commercially available taste tests with high reliability, validity and practicality are now being developed for use by physicians,17, 18 and electrogustometry, which has been available for a number of years, provides quantitative assessments of taste function that are correlated with the number of underlying taste buds.19
Figure 88.3 The 40-odorant, self-administered, University of Pennsylvania Smell Identification Test (UPSIT). Each page contains a microencapsulated odorant that is released by means of a pencil tip. Answers are marked on the columns on the last page of each booklet. Copyright 2004, Sensonics, Inc., Haddon Heights, NJ 08035.
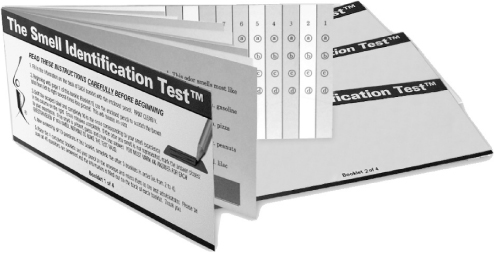
Traditionally, physicians have assumed that if a patient presenting with the complaint of anosmia fails to report the presence of an irritating vapour via CN V, he or she is malingering. However, this test is not foolproof, as even the most ardent malingerer rarely denies not perceiving a strong irritating substance, particularly one which leads to reflexive mucous secretion or eye watering. Furthermore, trigeminal thresholds to chemicals can be quite variable among individuals. Thus, a more valid means for detecting malingering is to determine the percentage of responses to stimuli that are correct in a forced-choice situation where chance responding can be calculated. When significantly fewer correct responses than expected on the basis of chance responding are demonstrated, malingering is suspected.
Age-Related Changes in Olfactory Function
The now well-established age-related decline in olfactory function is exemplified in Figure 88.4.2 As can be seen in this figure, considerable average decline occurs in the ability to identify odours in persons after the age of 60 years. In general, olfactory identification ability peaks, for both men and women, during the third to fifth decades of life and significantly declines in the seventh decade. Women outperform men at all ages, with the gender gap increasing in later years.21
Figure 88.4 Scores on the University of Pennsylvania Smell Identification Test (UPSIT) as a function of age in a large heterogeneous group of subjects. Numbers by data points indicate sample sizes. Reprinted with permission from Doty RL et al., Smell identification ability: changes with age. Science; 226: 1441–3. Copyright 1984 AAAS.
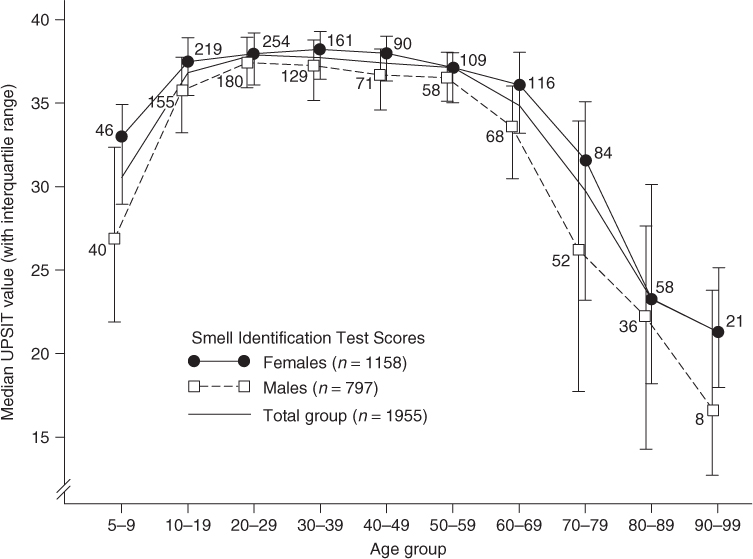
It is not known to what extent such age-related changes in olfactory function represent the process of ageing, per se, or alterations in the chemosensory systems brought about by factors correlated with age (i.e. cumulative viral insults, repeated exposures to environmental agents and air pollutants, alterations in trophic factors, the early progression of neurodegenerative disease pathology, etc.). It is now clear that cumulative exposure to high levels of air pollution significantly alters the ability to smell and may well contribute to neurodegenerative disease pathology.20 Age-related declines occurs, however, in all cultures, although large individual differences are present, and women, on average, maintain function later in life than men.
Age-Related Changes in Gustatory Function
Taste function, like olfactory function, also declines over the lifespan. Older persons show decreased ability to discern sweet, sour, bitter and salty tasting agents, including a number of amino acids at both threshold and suprathreshold levels. Functionally, however, such a decrease has much less impact on the individual than olfactory loss, since whole-mouth tests often show only moderate declines in age-related function.22
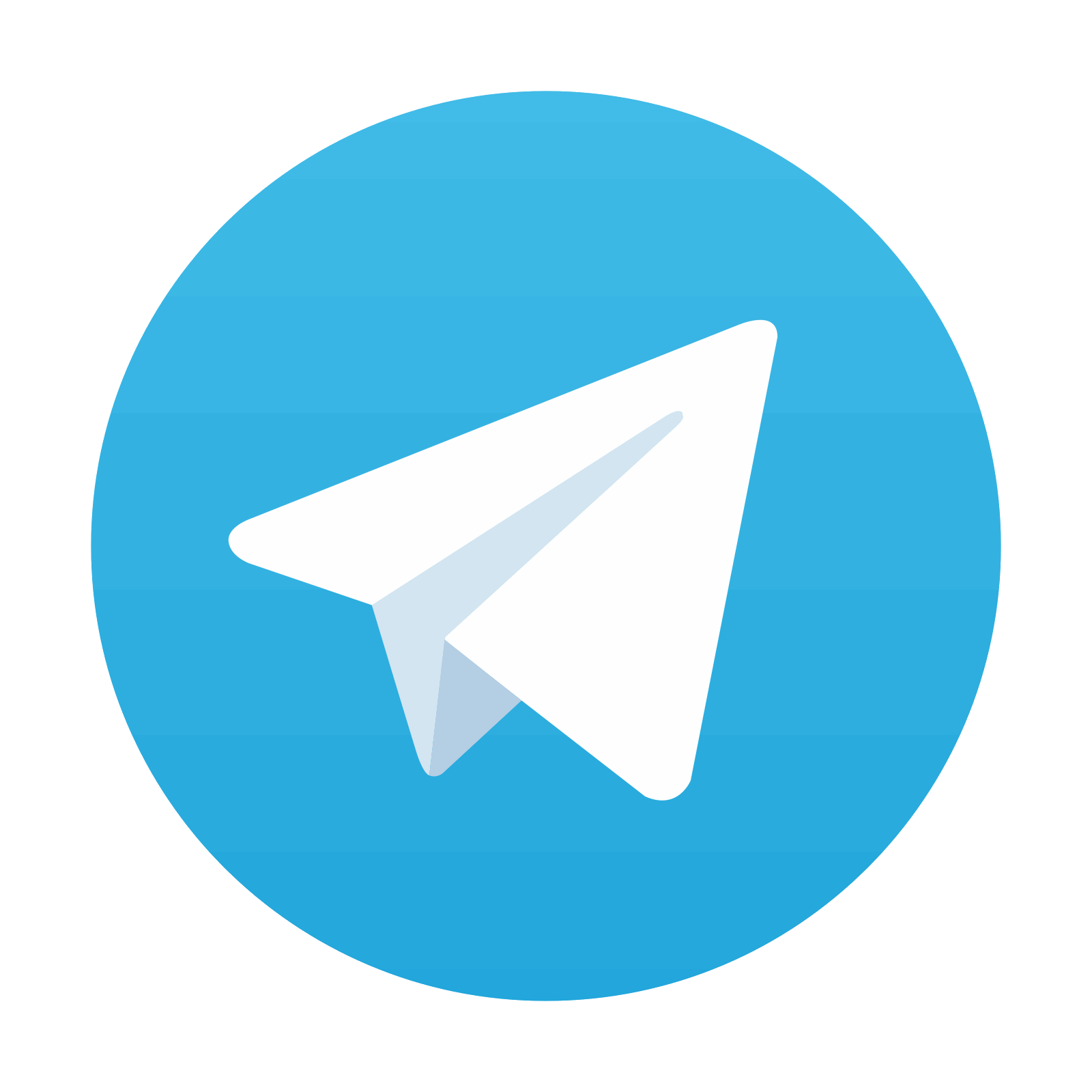
Stay updated, free articles. Join our Telegram channel

Full access? Get Clinical Tree
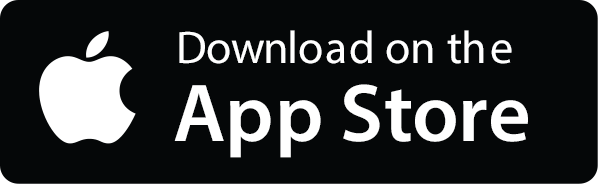
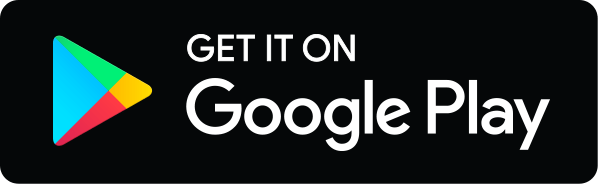