Sleep Disorders in the Allergic Patient
Lisa F. Wolfe
In the fourth century biblical scholars believed that sleep was “the incomplete experience of death.” The modern understanding that sleep is an active, complex, and essential behavior did not begin until the use of electroencephalography (EEG), which highlighted differences between wake and sleep (1).
Sleep Architecture
EEG has been used to identify the hallmarks of sleep and categorize stages of sleep (2,3). For ease of communication, sleep is divided first into either rapid eye movement sleep (stage REM) or nonrapid eye movement sleep (NREM). Although the eye movements of REM sleep were first discovered in 1953, it was not until 1957 that REM sleep was first described by EEG and the classic architecture of a full night’s sleep was first reported (4). These papers noted that REM sleep was associated with dreaming, and heart rate variability, and the episodes recurred about three to four times per night. Ultimately, in 1968, a formal protocol was developed for scoring sleep stages combining EEG, electro-oculography (EOG), and chin electromyography (EMG) (5).
Typical, normal adult sleep architecture is demonstrated in Fig. 42.1. Sleep onset is associated with NREM sleep. NREM sleep is composed of three stages: N1, N2, and N3. N3 is also referred to as slow wave sleep (SWS). During sleep onset, N1 sleep is seen with its characteristic slow rolling eye movements and easy arousability. N2 sleep is seen soon after and is defined by a specific EEG pattern referred to as K-complexes and spindles. It becomes more difficult to awaken the sleeper. N3 follows with hallmark EEG slow waves. SWS is associated with physiologic events including endocrine changes such as growth hormone release (6). NREM sleep becomes a bridge to initiate stage REM. Reduced muscle tone and ventilatory variability are associated with REM sleep.
![]() Figure 42.1 A representative sample of sleep from a healthy young adult without sleep complaints. W; wake; 1, N1 (NREM) sleep; 2, N2 (NREM) sleep; 3, N3 (slow wave) sleep; R, REM sleep. |
A complete cycle of NREM to REM sleep lasts approximately 90 minutes, and there are three to four cycles per night. SWS predominates at the beginning of the night and is virtually gone by the final cycle. Stage REM is minimal during the first cycle and concentrated in the early morning. Maturity also affects the architecture of sleep. With aging, there are significant reductions in SWS and sleep continuity. SWS decreases from 24% to 30% of the sleep period to 16% and wake after sleep onset (WASO) time increased from 2% to 4% to 17%. The percentage of REM sleep remains stable at about 20% of the total sleep time (TST) (7).
Determinates of Sleep Regulation
There are two processes that regulate the occurrence of sleep and the architecture of sleep periods. The homeostatic drive quantifies the physiologic need to sleep and the circadian pacemaker ensures proper timing of the sleep process. Additionally, the circadian pacemaker is influential in the architecture and NREM/REM distribution sleep stages throughout the night (8).
Circadian Rhythms
The word circadian is derived from Latin roots circa, about, and diem, day. The term circadian rhythm refers to any behavior or physiologic process that is known to vary in a predictable pattern over a 24-hour period. This internal process is governed by a three-component mechanism. First, inputs such as light and activity help synchronize (entrain) to the environment. These inputs are called zeitgebers, which is German for “time giver.” Next, information from zeitgebers is transferred to an internal clock, which acts as a pacemaker—setting the rate and timing of output pathways. Examples of these output pathways include lung function (9),
sympathetic tone (10), and urine production (11), all of which vary over a 24-hour period so that optimum performance occurs during the daytime.
sympathetic tone (10), and urine production (11), all of which vary over a 24-hour period so that optimum performance occurs during the daytime.
The circadian pacemaker is focused in a hypothalamic structure, the suprachiasmatic nucleus (SCN). Genes that play a role in generating circadian rhythms were first identified in the SCN and subsequently identified in cells of every organ. There are an ever-increasing number of genes that participate in a negative feedback system to regulate the circadian processes. The mammalian circadian genes include period (per 1, per 2, and per 3), clock (clock), B-mal (B-mal 1), casein kinase 1 epsilon/1 delta (CSNK1D and CSNK1E), cryptochrome (cry1 and cry2), and the nuclear hormone receptor Rev-erba. Less characterized components include Timeless, Dec1, Dec2, and E4bp4. These genes are highly conserved and mutations appear to impact many human conditions such as circadian rhythm disorders (advanced or delayed sleep phase), obesity, addiction, sleepiness, and bipolar disorder (12).
Sleep as a Homeostatic Process
Homeostasis is the process by which the body maintains stability. Thirst, hunger, and temperature are all processes that are carefully regulated to ensure optimum function. Sleep can be thought of as kin to these processes, and investigations in sleep deprivation have been the main tool for understanding the body’s drive. Utilizing spectral analysis of EEG, slow wave activity has been shown to be a significant hallmark of sleep debt, and as that debt is repaid by sleeping, slow wave activity is reduced (13). Sleep deprivation is increasing in prevalence in western societies. The percentage of U.S. Gallup poll respondents reported sleeping 7 or more hours on weeknights decreased from 63% in 1998 to 57% in 2005. This type of partial sleep deprivation is often associated with limited insight into impairments in cognitive performance. This phenomenon contributes to increases in car accidents for those that are chronically sleep deprived. Physiologic impacts of sleep deprivation include metabolic changes. Satiety hormones are altered such as decreases in Leptin and increases in Ghrelin. These findings have been associated with risk for obesity and diabetes (14).
Two Process Model
The two process model of sleep regulation has been used to explain the relationship between circadian rhythm regulation of sleep (process C) and the homeostatic drive to sleep (process S). Both processes S and C have an impact on sleep regulation, and to promote optimum sleep quality, maximum sleep debt should intersect with appropriate circadian time (15).
Sleep and the Physiology of The Immune System
Both sleep homeostasis and circadian rhythms modulate the expression of immune molecules and cells. Cytokines such as interleukin-2 (IL-2), tumor necrosis factor-α (TNF-α), and granulocyte macrophage-colony stimulating factor (GM-CSF) cycle in a circadian manner each with a unique pattern of expression (16). IL-6 on the other hand appears to be linked more to the sleep homeostatic process, levels peak in relationship to the degree of sleep deprivation, and is associated with latency to REM, sleep efficiency, and wake-after-sleep onset time (17,18). In Table 42.1 the influence of cytokines in the generation of non-REM sleep is summarized. The sleep process influences immune cells as well; lymphocytes, monocytes, and NK cells all have a diurnal rhythm of expression but this rhythm is modified by sleep (19).
Some of the circadian influence on the immune system is mediated through the impact of melatonin. Melatonin is a hormone produced in the pineal gland as a result of dark exposure and in coordination with the central circadian clock. Binding of melatonin to specific receptors in antigen-activated T-helper cells results in an upregulation of cytokine production and immune function. Human studies demonstrate that melatonin favors a TH1 cell response. The melatonin rhythm positively correlates with the rhythmicity of the TH1/TH2
cell ratio. This seems to be most prominent when in a state of stress or immune suppression. Melatonin may play an additional role in enhancing immunity as melatonin is produced in T-lymphocytes and acts in intracrine, autocrine, and/or paracrine fashion (20) (Table 42.2).
cell ratio. This seems to be most prominent when in a state of stress or immune suppression. Melatonin may play an additional role in enhancing immunity as melatonin is produced in T-lymphocytes and acts in intracrine, autocrine, and/or paracrine fashion (20) (Table 42.2).
Table 42.1 Summary of the Impact of Cytokines on Generation of Nonrapid Eye Movement Sleep | ||||||||||||||||
---|---|---|---|---|---|---|---|---|---|---|---|---|---|---|---|---|
|
Table 42.2 Cytokines That Increase in Response to Nocturnal Elevation in Melatonin | ||||||||
---|---|---|---|---|---|---|---|---|
|
Immunology Impacts of Sleep Deprivation
Sleep deprivation on human immune function has many areas of impact. One night of sleep loss can induce a significant increase in E-selectin, intercellular adhesion molecule-1 (ICAM-1), IL-1β, and IL-1ra, while suppressing C-reactive protein and IL-6 (21). Sleep deprivation limits the ability of the immune system to function and respond to an influenza vaccine challenge (22). Sleep loss reduces myeloid dendritic cell precursors that produce IL-12, thereby resulting in a limit to antigen presentation due to sleep reduction (23). Overall, cellular immunity has been difficulty to evaluate as multiple studies have shown contradictory results depending on the amount of sleep loss and the cellular immunity components studied (24). Humoral immunity is also impacted by sleep loss with increasing serum IgG, IgA, IgM, and C3, C4 (25). Sleep deprivation drives up IL-6 levels and is associated with increasing complaints of pain (26). Response to influenza vaccination is impaired in the setting of chronic partial sleep loss (22,27).
Sleep Disorders in the Allergy Patient
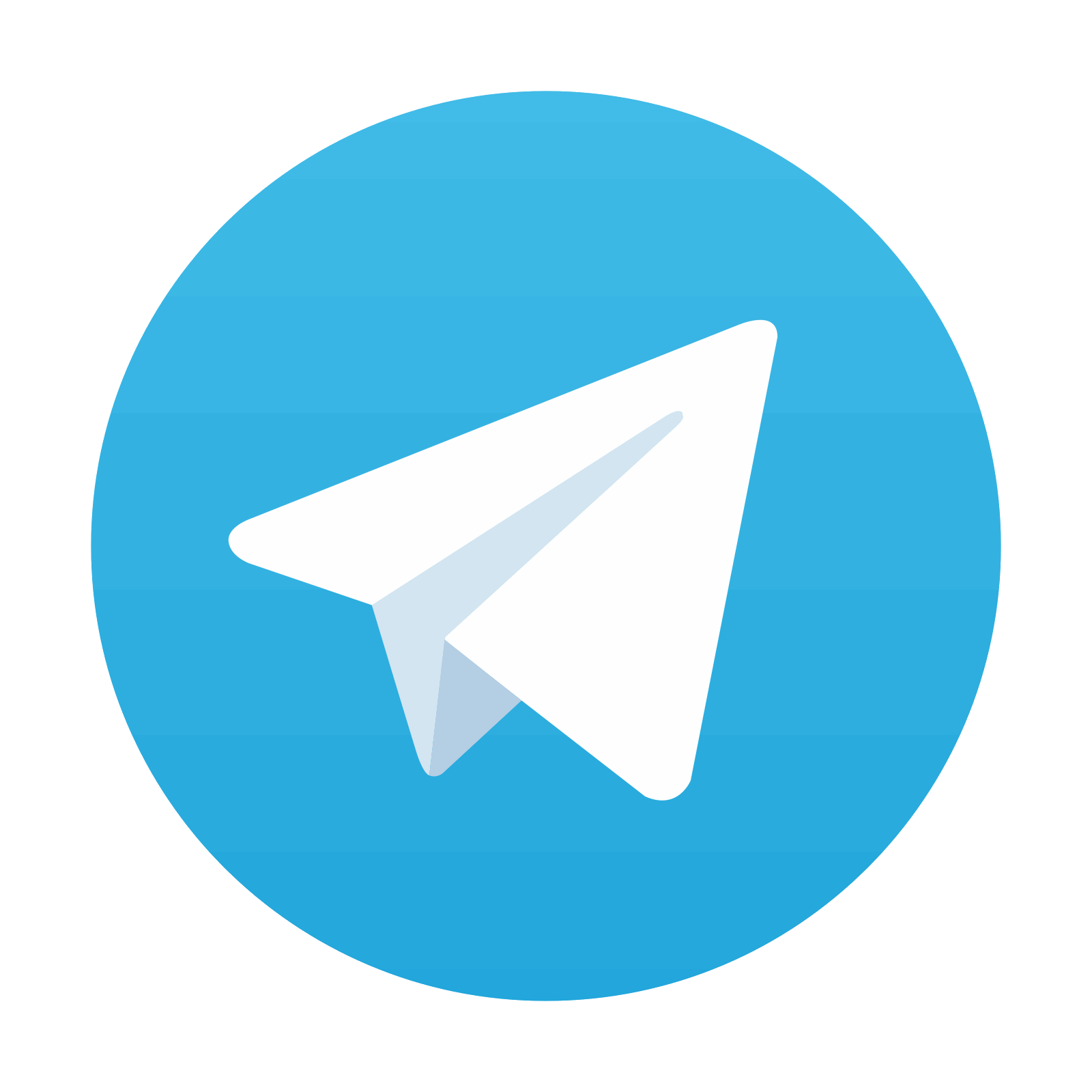
Stay updated, free articles. Join our Telegram channel

Full access? Get Clinical Tree
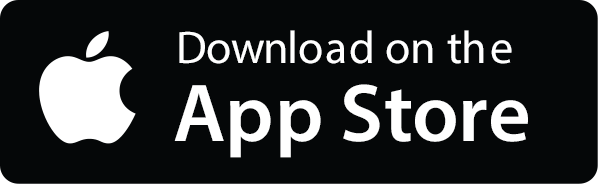
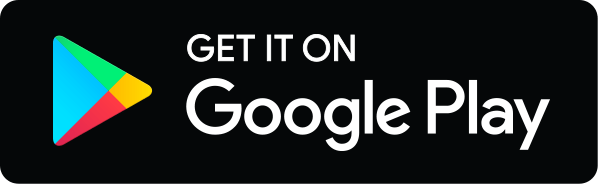