Skeletal Muscle Anatomy, Physiology, and Mechanics
Bryan T. Kelly
William J. Robertson
Muscle Structure and Function
Myofibrils and Connective Tissue
Myofibrils represent the basic structural element of skeletal muscle (Fig. 26-1).
Along with their innervating nerves, myofibrils are responsible for the contractile function of skeletal muscle.
Myofibrils fuse together to form ribbon-like multinucleated cells called muscle fibers or myofibers.
Muscle fibers varying in length from a few millimeters to 50 cm.
Muscle is structurally organized by three connective tissue layers: the endomysium, perimysium, and epimysium (Table 26-1).
Endomysium: a delicate connective tissue layer (basement membrane) that surrounds each muscle fiber
Perimysium: an enveloping connective tissue layer that bundles multiple muscle fibers to form larger fascicles
Epimysium: a stronger, thick connective tissue sheath that combines and surrounds muscle fascicles to form the entire muscle belly
This connective tissue framework allows individual muscle cells, nerves, and capillaries to work together during muscle contraction.
Intracellular Organelles and Architecture
Below the layer of endomysium that covers each muscle fiber sits a plasma membrane called the sarcolemma (Fig. 26-2 and Table 26-2).
The ends of each fiber are attached to the extracellular matrix (ECM) through the sarcolemma by way of protein molecules called integrins.
Table 26-1 Muscle Structural Organization
Structural Units of Skeletal Muscle Organized from Smallest to Largest
Separating Structures Between Structural Elements of Skeletal Muscle
Myofibrils
Muscle fibers
Endomysium
Muscle fascicles
Perimysium
Muscle belly
Epimysium
Table 26-2 “Sarco” Terminology
Term
Definition
Sarcolemma
Limiting plasma membrane surrounding individual muscle fibers (myofibers)
Sarcoplasm
Fluid material within the sarcolemma surrounding the myofibrils
Contains Golgi apparatus, mitochondria, ribosomes, sarcoplasmic reticulum, glycogen, and lipid droplets
Sarcoplasmic reticulum
Network of closed sacs, rich in calcium ions, coursing around myofibrils in a longitudinal orientation
Communicate with cell membrane by way of transverse tubules
Sarcomere
Repeating structural units of the myofibril that span between Z lines
Integrins are a family of adhesions receptors that provide muscle fibers with the strength to withstand tensile forces at the myotendinous junction (MTJ), while also playing vital roles such as cell-to-cell signaling, cell-to-ECM interactions, and signal transduction.
Within the sarcolemma there exists a fluid material, or sarcoplasm, that encompasses large numbers of longitudinally oriented myofibrils and numerous nuclei that compose each muscle fiber.
The sarcoplasm contains a Golgi apparatus, many mitochondria, ribosomes, sarcoplasmic reticulum, glycogen, and lipid droplets.
The sarcoplasmic reticulum is a network of closed sacs that course around the myofibrils in a primarily longitudinal direction.
These sacs are rich in calcium ions.
They communicate with the cell membrane by way of transverse tubules.
Myofibril Structural Composition (see Fig. 26-1)
Each myofibril exhibits periodic cross-striations.
These striations are alternating light and dark bands of isotropic and anisotropic materials, respectively, composed of repeating units called sarcomeres that spans from one Z line to the next Z line.
The light and dark bands of a sarcomere are created by overlapping thin and thick filaments, also termed myofilaments.
These thin and thick filaments are made up of specific proteins that, in the presence of calcium, are responsible for force production within the muscle.
Thin and Thick Filaments
Thin Filaments
Thin filaments consist of three proteins (actin, tropomyosin, and troponin), which form the I band of a myofibril (see Fig. 26-2).
Major component of the thin filament is actin, consisting of two polymers:
G-actin, or globular actin
On binding with ATP, it polymerizes to the F form, or fibrous actin.
F-actin binds calcium tightly.
Actin has long, thin fibers attached to its surface that inhibit contraction by blocking actin and myosin cross-bridges. These fibers are called tropomyosin fibers and are attached to actin by troponin molecules.
Troponin is a three-subunit molecule (troponin-I, troponin-T, and troponin-C) that can bind actin, tropomyosin, and calcium, respectively. In the absence of adequate calcium levels, troponin binds tropomyosin to actin and inhibits contraction.
A high enough concentration of calcium within the sarcoplasm will allow calcium to bind to the troponin-C subunit. A conformational change then results in the other troponin subunits. These changes release the inhibitory effect of tropomyosin and allow actin and myosin interaction.
As the calcium concentration falls, the conformation of troponin reverts back to its inhibitory form, shifting tropomyosin to again stearicly inhibit cross-bridges.
Thick Filaments
Formed by the muscle protein myosin
Thick filaments span the midportion of the sarcomere forming the A band of a myofibril.
I bands contain only thin filaments, whereas A bands contain both thick and thin filaments, except in the central H zone, where only thick filaments are present (see Fig. 26-1).
Myosin is the largest of the myofibril proteins, making up >50% of muscle mass.
Myosin molecule resembles a thin rod with two small, globular heads.
This globular end has significant ATPase activity in the presence of ionic calcium and serves as a cross-bridge, binding myosin with actin during muscle contraction.
Changes During Contraction
When a muscle fiber contracts, each fiber and each sarcomere shortens.
Thick and thin filaments are arranged so that they can slide past each during contraction.
With maximal contraction a sarcomere shortens 20% to 50% of its normal resting length.
The I band becomes shorter and the H zone usually disappears.
The A band does not change in length during contraction or relaxation.
A sarcomere can extend to 120% of its length during passive stretching, and the I band becomes longer.
Neuromuscular Interaction
Each nerve cell axon branches many times, providing each muscle fiber with a point of contact called the motor endplate.
This single nerve axon and all of the muscle fibers it innervates constitute a motor unit.
Motor Unit
Both the size of the motor unit and the number of motor units within a given muscle are variable.
Muscles that require fine motor control and coordinated movements, such as the extraocular muscles, have small motor units.
Larger, more powerful muscles, such as the gastrocnemius muscle, have large motor units.
Stimulus for Muscle Contraction
The arrival of an electrical impulse at the terminal axon leads to an inflow of calcium ions.
Acetylcholine-containing vesicles fuse with the axon membrane and release acetylcholine into the synaptic cleft.
Acetylcholine binds to receptors on the muscle cell membrane, causing an inflow of current and depolarizing the motor endplate.
Sodium, potassium, and chloride ions move about
within transverse tubules to spread the impulse to the sarcoplasmic reticulum (see Fig. 26-2).
Depolarization of the sarcoplasmic reticulum causes a release of calcium ions into the sarcoplasm.
This in turn causes all filaments within a muscle fiber to contract together.
Muscle Contraction
Muscle contraction is initiated by the release of ionic calcium into the sarcoplasm.
In absence of calcium, troponin and tropomyosin interfere with the formation of active complexes between actin and myosin.
With the binding of free calcium, troponin undergoes a conformational change, releasing tropomyosin from actin and allowing the formation of cross-bridges between actin and myosin (see Fig. 26-2).
Intracellular calcium also activates the myosin—ATP complex, which motors the sliding action between actin and myosin.
Energy provided by ATP drives the pulling of the actin molecule past myosin, shortening the fibril.
As long as the calcium concentration in the cell is maintained at a high enough level, the myosin ATPase remains active, permitting the fibril to stay in its contracted form.
When the energizing impulse terminates, calcium is rapidly pumped out of the sarcoplasm.
As the calcium ion concentration drops, the calcium—troponin interaction is uncoupled.
Tropomyosin can again bind to actin, and the troponin—actin—tropomyosin B complex impedes cross-bridging between actin and myosin.
Calcium reuptake by the sarcoplasmic reticulum will not occur if there is insufficient ATP available, which may lead to muscle contraction without electrical stimulation, as is seen in rigor mortis.
Fiber Types and Adaptability
In lower mammals and other animals, muscles are generally composed entirely of either type I or type II fibers.
Human muscle is made up of a mixture of type I and type II fibers.
Muscle fiber type depends not on any intrinsic feature of the fiber itself, but on the motor neuron supplying that fiber.
All muscle fibers supplied by one particular axon will be of either type I or type II.
There are significant differences in the fiber types.
Type I Fibers
Type I muscle fibers are rich in the enzymes necessary for oxidative metabolism and are darker in appearance.
Contain a higher concentration of mitochondria and more capillaries per fiber than type II muscle fibers
When stimulated, they have a slow contraction or “twitch” time.
These fibers have increased resistance to fatigue. * Type I fibers are well suited for activities related to physical effort requiring strength and endurance that depend on the metabolism of oxidative processes for energy.
Type II Fibers
Type II fibers obtain their energy through a much faster glycolytic process.
As glycogen stores are more rapidly depleted than oxygen supplies, type II fibers are less suited to continuous types of activity and are more suited to rapid alternating effort.
Those muscles most accustomed to slow, continuous work have a lower percentage of type II muscle fibers.
Type II fibers may be more prone to anatomic changes following altered energy demands than are type I.
Type II fibers tend to be smaller than type I in children and in adults who do not carry out strenuous physical exercise, although they increase in size with repeated physical demands on the muscle.
Type II fibers are subdivided into types IIa and IIb.
Type IIa fibers have an admixture of glycolytic and oxidative enzymes and show an intermediate twitch time.
Type IIb fibers (fast glycolytic fibers) have the largest motor unit size, have the fastest rate of contraction, and are most susceptible to fatigue.
Fiber Type Interconversion
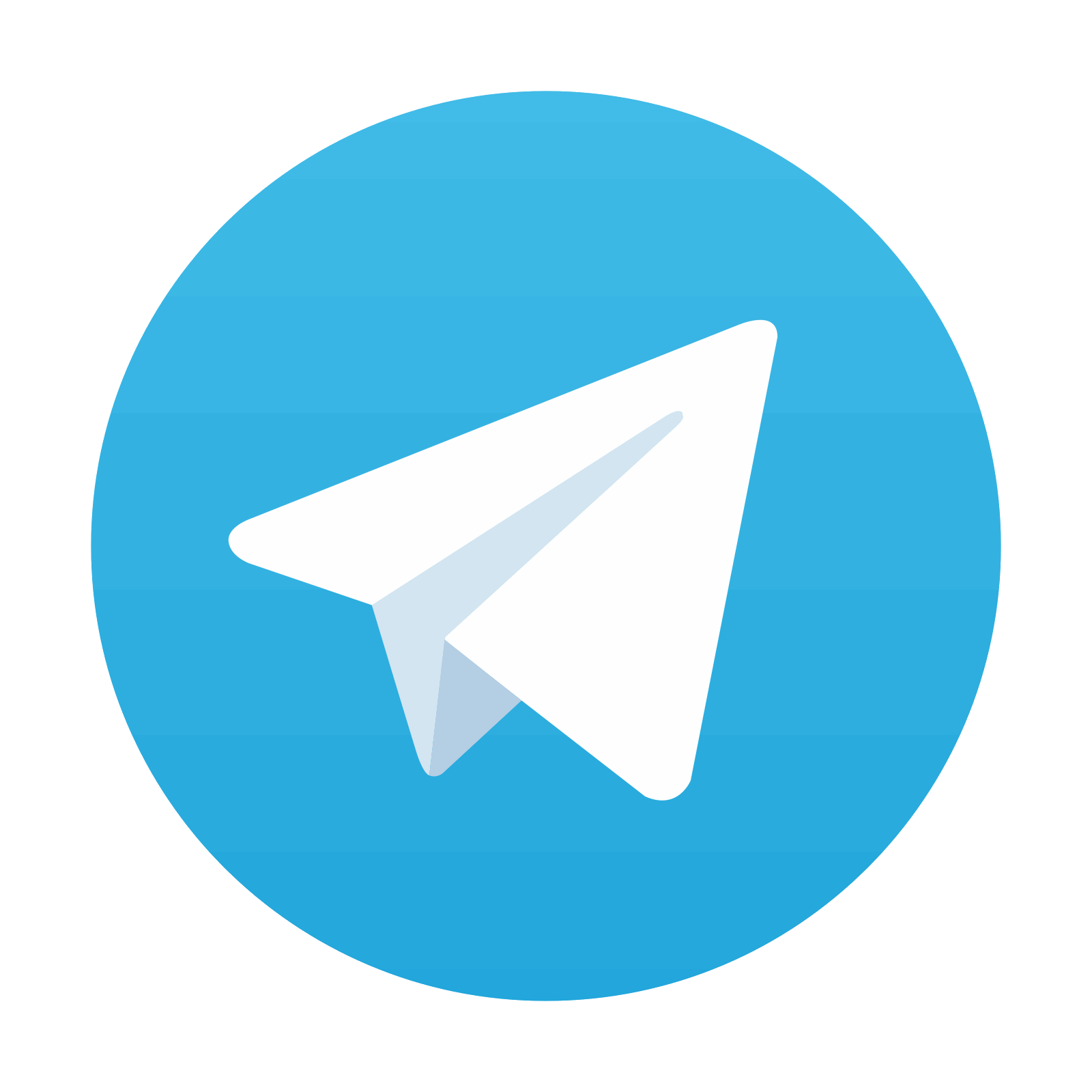
Stay updated, free articles. Join our Telegram channel

Full access? Get Clinical Tree
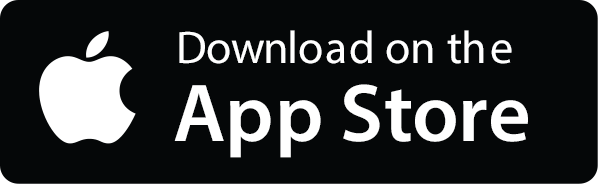
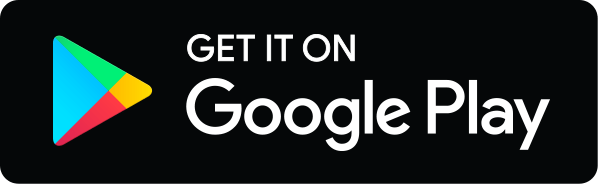