individual receptors’ relative abundance and activation status vary with specific cells, tissues, and physiological or pathological conditions.
![]() FIGURE 30-1 The insulin receptor family includes the IR (in isoforms IR-A and IR-B), the type 1 insulin-like growth factor (IGF-IR), and the mannose-6-phosphate receptor (IGF-IIR). IR and IGF-IR, both tyrosine kinase receptors, are expressed as preformed dimers, either as homodimers or heterodimers (IR/IGF-IR). IGF-IIR is a non-signaling receptor that acts as a “sink” for IGF-II. Insulin binds primarily to the two isoforms of the IR receptor, but also it has weak affinity for IR/IGF-IR heterodimer. IGF-I and IGF-II are ligands for the IGF-IR and IR/IGF-IR hybrid receptor; IGF-II also binds to IR-A isoform. IGF-BPs bind to and prevent IGF-I and -II from activating the receptor signaling cascades. (Please see Color Insert.) |
TABLE 30.1 Monoclonal antibodies IGF-1R or IGF (status as of 7/09) in clinical trials | ||||||||||||||||||||||||||||||||||||||||||||||||||||||||||||||||||||||||||||||||||||||||
---|---|---|---|---|---|---|---|---|---|---|---|---|---|---|---|---|---|---|---|---|---|---|---|---|---|---|---|---|---|---|---|---|---|---|---|---|---|---|---|---|---|---|---|---|---|---|---|---|---|---|---|---|---|---|---|---|---|---|---|---|---|---|---|---|---|---|---|---|---|---|---|---|---|---|---|---|---|---|---|---|---|---|---|---|---|---|---|---|
|
mAbs against the ligand (IGF-I and II), and 3) IGF-1R TKIs. Clinical data are available for IGF-1R mAbs and TKIs.
TABLE 30.2 Small molecule tyrosine kinase inhibitors against IGF-1R | ||||||||||||||||||||||||||||||||||||||||||||||||||||||||||||||
---|---|---|---|---|---|---|---|---|---|---|---|---|---|---|---|---|---|---|---|---|---|---|---|---|---|---|---|---|---|---|---|---|---|---|---|---|---|---|---|---|---|---|---|---|---|---|---|---|---|---|---|---|---|---|---|---|---|---|---|---|---|---|
|
potentially block all receptors responsible for IGF/insulin signaling. The differing spectrum of target blockade may potentially translate into different toxicity and/or activity profiles.
TABLE 30.3 Main features of mAbs and small molecule TKIs against the IGF/IGF-1R pathway | ||||||||||||||||||||
---|---|---|---|---|---|---|---|---|---|---|---|---|---|---|---|---|---|---|---|---|
|
inhibitory effect on the IR. For example, OSI-906 has a half maximal inhibitory concentration (IC50) of 0.018 μM (micromolar) versus 0.054 μM against the IGF-1R and IR, respectively.39,40 At the clinically relevant doses, this agent is expected to inhibit IGF-1R and IR simultaneously. Coinhibition of IR in addition to IGF-1R could confer therapeutic advantage and potentially increase toxicities. IR signaling by insulin or IGF-II has been implicated in a number of preclinical tumor models,41 and IR overexpression is common in breast cancer.42 Furthermore, when the IGF-1R signaling is disrupted, cells may respond with an increase in IR signaling.43
ongoing in CRC, NSCLC, and head and neck cancer. In addition, NCI/CTEP is sponsoring randomized phase 2 trials for lapatinib (GSK-57216, Tykerb) with or without IMC-A12 in HER-2-positive (by gene amplification) breast cancer and erlotinib (OSI-774, Tarceva)/gemcitabine with or without IMC-A12 in patients with pancreatic cancer. Results of clinical testing are not yet available.
Tumor genomic alternations within the IGF-1R axis;
Genetic alterations outside the IGF-1R axis that may affect the IGF-1R signaling (e.g., chromosomal translocations resulting in transcriptional modulations of the IGF-ligand or receptors);
Level and phosphorylation status of IGF-1R and IR;
Bioavailability of the ligand (including IGFBP3, IGF-I/II, and decoy receptor IGF-2R);
Activation mutations of the downstream molecules such as AKT, PI3K, RAS, and RAF; markers related to parallel pathways such as EGFR and vascular endothelial growth factor (VEGF); and
Markers related to epithelial-mesenchymal transition.
other important downstream targets. Phosphoprotein profiling and functional genomic studies demonstrate that many cancer cell lines and human breast tumors with mutations of the catalytic (p110α) subunit of PI3K (PI3KCA) have only minimal AKT activation and little requirement of AKT for anchorage-independent growth.68 These cells, instead, are characterized by robust PDK1 activation and have dependency on the PDK1 substrate serum/glucocorticoid regulated kinase 3 (SGK3). SGK3 undergoes PI3K- and PDK1-dependent activation in PIK3CA-mutant cancer cells. Therefore, PI3K activation may promote cancer through both AKT-dependent and -independent mechanisms, with important implications for the treatment of patients with these tumors.
![]() FIGURE 30-2 Activation of integrin receptors, growth factor receptors, and cytokine receptors activates the PI3K/AKT signaling pathway, leading to the activation of the mTOR (part of the mTORC1 complex). Activation of mTORC1 leads to the phosphorylation of S6K1 and 4E-BP1. S6K1 phosphorylates rpS6, while phosphorylated 4E-BP1 dissociates from eIF-4E. Both rpS6 and eIF-4E promote mRNA transcription and protein translation, leading to cell growth and proliferation, angiogenesis, and the prevention of apoptosis. mTOR inhibitors, such as temsirolimus, bind to FK506-binding protein (FKBP) and inhibit the kinase activity of the mTORC1 complex. (Please see Color Insert.) |
threonine protein kinase, which acts to control protein translation in response to nutrients, hypoxia, energy levels, hormones, and growth factors, all modulated through the PI3K/AKT pathway. AKT can directly activate mTOR through phosphorylation but also causes indirect activation of mTOR by inhibitory phosphorylation of tuberous sclerosis complex 2 (TSC2). TSC2 inhibits mTOR through the guanosine triphosphate (GTP)-binding protein, Ras homolog enriched in brain (Rheb). Inhibitory phosphorylation of TSC2 by AKT results in conversion of Rheb to an active form that phosphorylates and activates mTOR. mTOR exists in two complexes: target of rapamycin complex 1 (TORC1) of mTOR, which is bound to the regulatory-associated protein of mTOR (Raptor), and the TORC2 complex, in which mTOR is bound to Rictor. The TORC1 complex includes a novel component, proline-rich AKT substrate (PRAS40), and TORC2 includes the proteins stress-activated map kinase-interacting protein 1 (SIN1) and proline-rich protein 5 (PRR5).78 PRAS40 acts as an inhibitor of mTOR kinase activity in a phosphorylation-dependent manner.77
TABLE 30.4 Components of the mTOR pathway with evidence of involvement in human cancers | ||||||||||||||||
---|---|---|---|---|---|---|---|---|---|---|---|---|---|---|---|---|
|
It is currently being tested in oral formulations in several early phase trials in various cancers in daily and weekly dosing schedules.117
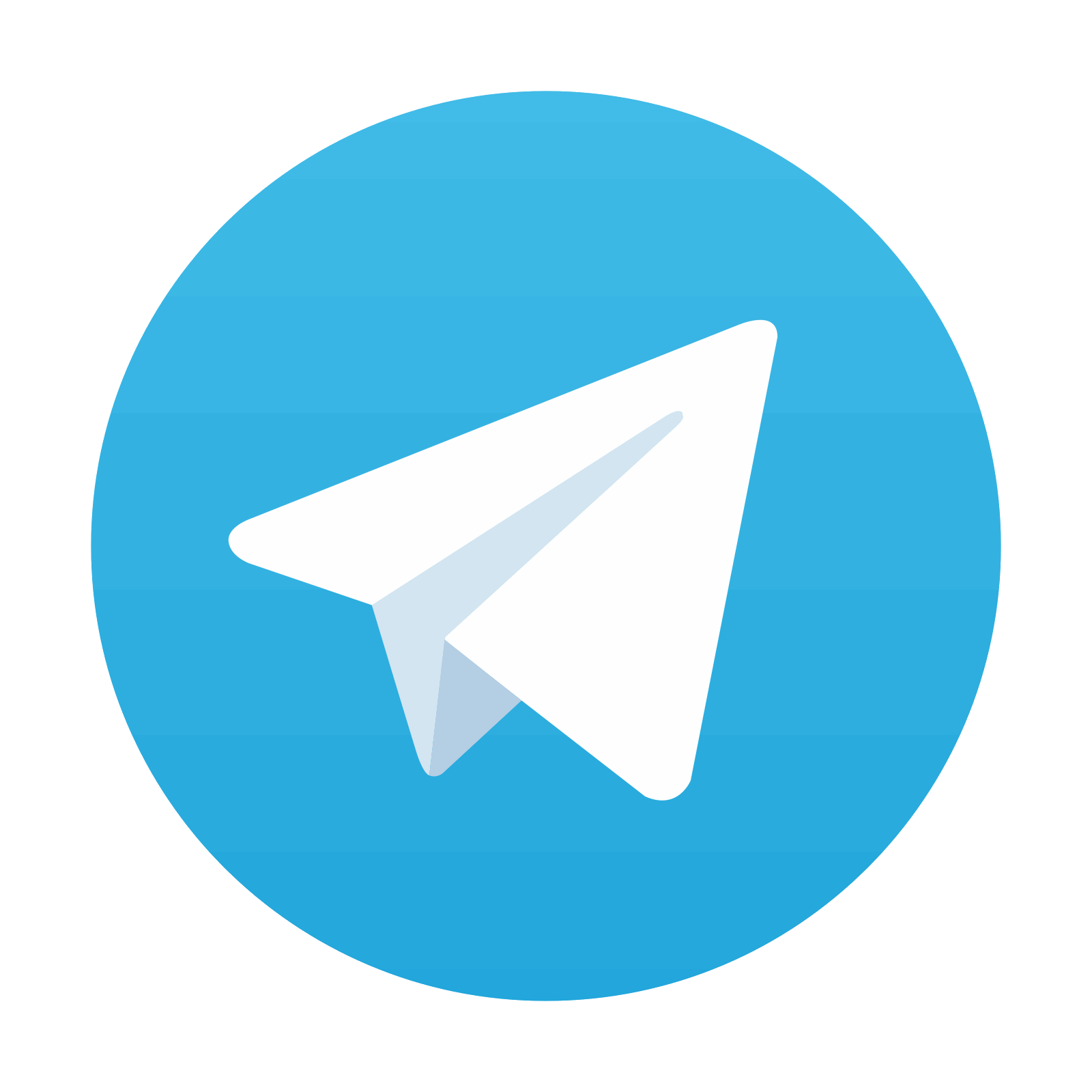
Stay updated, free articles. Join our Telegram channel

Full access? Get Clinical Tree
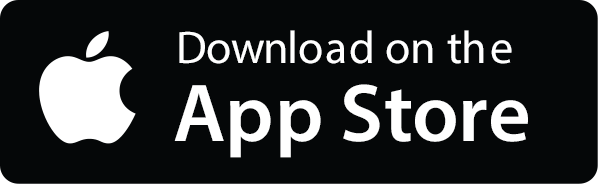
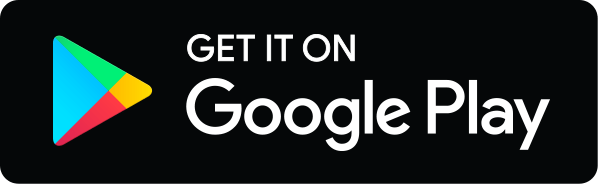
