Clinical genetic testing for cancer predisposition syndromes often identifies DNA changes whose effects cannot be interpreted easily. These changes, often referred to as variants of uncertain significance (VUS), are not useful for clinical management. In contrast with clearly pathogenic mutations, VUS do not firmly diagnose a specific syndrome at the molecular level and cannot be used to identify with certainty which relatives are mutation carriers and which relatives are free of the syndrome. This article discusses the approach to evaluating VUS and how clinicians can play a key role in advancing the field to benefit all patients.
Key points
- •
Variants of uncertain significance (VUS) comprise a significant and growing number of results from germline testing associated with many hereditary cancer syndromes.
- •
The biological interpretation and clinical significance of VUS are complex and challenging.
- •
Unlike pathogenic germline DNA variants, VUS are generally not useful for clinical management; however, the goal of the genetics community is to gather different types of evidence that will eventually lead to the classification of all VUS as either pathogenic or neutral.
- •
It is important for clinicians, and surgical oncologists in particular, to understand that they can contribute to VUS classification efforts by documenting clinicopathologic data, referring patients and their families to genetic counselors, and encouraging patients to share data and participate in cancer genetics research studies.
- •
Management of patients at increased cancer risk, and evaluation of difficult genetic variants and their cancer risks, are both complex tasks that rely on combining multiple lines of evidence.
Terminology and definitions in cancer genetics
Clinicians in the coming era will need to be familiar with the basics of genetic terminology and principles, so this article reviews some terms and principles that apply to DNA variations. Some of the nomenclature of DNA variant interpretation is new and confusing to clinicians, and often to researchers as well. Ongoing international efforts exist to standardize genetic and phenotypic terminology so that the field is more accessible to researchers, clinicians, and patients.
Basic Genetics
Only about 2% of human DNA codes for genes that produce proteins. Much of the remaining 98% of the genome’s noncoding DNA is now known to have regulatory functions that are incompletely understood but are the subject of intense study. Current genetic testing for cancer susceptibility generally deals almost exclusively with protein-coding genes. However, it is anticipated that in the future more regulatory regions will be associated with hereditary diseases, including cancer susceptibility syndromes.
A gene is composed of DNA sequences that usually span thousands of base pairs (adenine [A] pairing with thymine [T], guanine [G] pairing with cytosine [C]). The cell reads the DNA code of genes, first producing a complementary strand of messenger RNA (mRNA), by the process of gene transcription. mRNA is then converted into a chain of amino acids that forms a cellular protein by the process of translation using the triplet nucleotide code (3 nucleotide bases translate into 1 amino acid). Genes contain several regions with different functions. In the coding sequences, called exons, the genetic code is read by cellular machinery and converted into a protein. Coding exons are short stretches of DNA separated by sequences called introns, which are stretches of DNA ranging from dozens to thousands of DNA bases that do not code for protein. They are transcribed into mRNA, but are spliced out before mRNA is translated. In addition, there are regulatory sequences that indicate the beginning and end of a gene, and others that code for whether the cell should produce the protein and in what amounts. The consensus normal sequence of a gene and protein is called the wild type. DNA can be altered (mutated) in any gene region, and any of these alterations may result in (1) abnormal protein structure, function, and/or expression, causing increased disease susceptibility; or (2) protein structure, function, and expression that is not significantly changed from the normal sequence and does not cause disease.
Clinicians interpret the effects of a DNA change based on knowledge of genetic principles and on observed effects in vivo and in vitro. Reports from clinical genetic testing may indicate abnormalities caused by changes in amino acid, truncation of protein length, abnormal splicing, large gene deletion, and other mechanisms. Interpretation may be uncertain regarding any of these mechanisms. The different types of variants that are seen are:
Missense
Change from one amino acid to another without changing protein length.
Nonsense
Creation of a premature stop codon, coding for a shortened protein that may or may not be transcribed by the cell.
Frameshift
Deletion or insertion of a few DNA nucleotides, not in multiples of 3, that changes the reading frame of the mRNA message. This variant almost always results in a few new incorrect amino acids followed by a premature stop codon.
Insertion or deletion
Insertion or deletions (indels) can be large or small. Small indels are deletions or insertions of a few DNA nucleotides in multiples of 3, which result in the addition or subtraction of 1 or a few amino acids from the protein chain. Large deletions can involve many thousands of DNA nucleotides, resulting in the loss of large regions or an entire protein.
Splicing
Splicing is deletion of a full exon or exons, resulting in a deletion of a segment of the protein, and possibly also a frameshift.
Regulatory
Change in a DNA nucleotide in a region that controls whether a gene is successfully translated into a protein by the cell.
Silent
A change in DNA nucleotide that results in a different DNA code for the same amino acid. Silent mutations sometimes affect splicing or regulatory functions.
Genetic Variation Nomenclature
Clinical reports from genetic testing can be confusing. In the past, a genetic change that was seen commonly in populations and presumed to be benign was called a polymorphism, and one that was thought to cause disease was called a mutation. The term variant is now often used to refer to any DNA change. With the recognition of many variants of uncertain significance (VUS), the term mutation has become ambiguous and cannot be assumed to mean that the change is associated with disease. The clearest terminology is now to refer to all DNA changes as variants, and to classify them as pathogenic (disease causing) or neutral (benign) when the association with disease has been assessed. Other terms have been borrowed from different areas of variant science. Important examples that are seen and are related to, but not identical to, pathogenic or neutral include damaging, which is the preferred way to refer to a change that alters a protein’s function versus mutation; and deleterious, referring to a change that is harmful to an organism and therefore is not seen in any species through evolution. Both of these terms apply to specific types of nonclinical evidence, and do not reflect clinical interpretation.
Terminology and definitions in cancer genetics
Clinicians in the coming era will need to be familiar with the basics of genetic terminology and principles, so this article reviews some terms and principles that apply to DNA variations. Some of the nomenclature of DNA variant interpretation is new and confusing to clinicians, and often to researchers as well. Ongoing international efforts exist to standardize genetic and phenotypic terminology so that the field is more accessible to researchers, clinicians, and patients.
Basic Genetics
Only about 2% of human DNA codes for genes that produce proteins. Much of the remaining 98% of the genome’s noncoding DNA is now known to have regulatory functions that are incompletely understood but are the subject of intense study. Current genetic testing for cancer susceptibility generally deals almost exclusively with protein-coding genes. However, it is anticipated that in the future more regulatory regions will be associated with hereditary diseases, including cancer susceptibility syndromes.
A gene is composed of DNA sequences that usually span thousands of base pairs (adenine [A] pairing with thymine [T], guanine [G] pairing with cytosine [C]). The cell reads the DNA code of genes, first producing a complementary strand of messenger RNA (mRNA), by the process of gene transcription. mRNA is then converted into a chain of amino acids that forms a cellular protein by the process of translation using the triplet nucleotide code (3 nucleotide bases translate into 1 amino acid). Genes contain several regions with different functions. In the coding sequences, called exons, the genetic code is read by cellular machinery and converted into a protein. Coding exons are short stretches of DNA separated by sequences called introns, which are stretches of DNA ranging from dozens to thousands of DNA bases that do not code for protein. They are transcribed into mRNA, but are spliced out before mRNA is translated. In addition, there are regulatory sequences that indicate the beginning and end of a gene, and others that code for whether the cell should produce the protein and in what amounts. The consensus normal sequence of a gene and protein is called the wild type. DNA can be altered (mutated) in any gene region, and any of these alterations may result in (1) abnormal protein structure, function, and/or expression, causing increased disease susceptibility; or (2) protein structure, function, and expression that is not significantly changed from the normal sequence and does not cause disease.
Clinicians interpret the effects of a DNA change based on knowledge of genetic principles and on observed effects in vivo and in vitro. Reports from clinical genetic testing may indicate abnormalities caused by changes in amino acid, truncation of protein length, abnormal splicing, large gene deletion, and other mechanisms. Interpretation may be uncertain regarding any of these mechanisms. The different types of variants that are seen are:
Missense
Change from one amino acid to another without changing protein length.
Nonsense
Creation of a premature stop codon, coding for a shortened protein that may or may not be transcribed by the cell.
Frameshift
Deletion or insertion of a few DNA nucleotides, not in multiples of 3, that changes the reading frame of the mRNA message. This variant almost always results in a few new incorrect amino acids followed by a premature stop codon.
Insertion or deletion
Insertion or deletions (indels) can be large or small. Small indels are deletions or insertions of a few DNA nucleotides in multiples of 3, which result in the addition or subtraction of 1 or a few amino acids from the protein chain. Large deletions can involve many thousands of DNA nucleotides, resulting in the loss of large regions or an entire protein.
Splicing
Splicing is deletion of a full exon or exons, resulting in a deletion of a segment of the protein, and possibly also a frameshift.
Regulatory
Change in a DNA nucleotide in a region that controls whether a gene is successfully translated into a protein by the cell.
Silent
A change in DNA nucleotide that results in a different DNA code for the same amino acid. Silent mutations sometimes affect splicing or regulatory functions.
Genetic Variation Nomenclature
Clinical reports from genetic testing can be confusing. In the past, a genetic change that was seen commonly in populations and presumed to be benign was called a polymorphism, and one that was thought to cause disease was called a mutation. The term variant is now often used to refer to any DNA change. With the recognition of many variants of uncertain significance (VUS), the term mutation has become ambiguous and cannot be assumed to mean that the change is associated with disease. The clearest terminology is now to refer to all DNA changes as variants, and to classify them as pathogenic (disease causing) or neutral (benign) when the association with disease has been assessed. Other terms have been borrowed from different areas of variant science. Important examples that are seen and are related to, but not identical to, pathogenic or neutral include damaging, which is the preferred way to refer to a change that alters a protein’s function versus mutation; and deleterious, referring to a change that is harmful to an organism and therefore is not seen in any species through evolution. Both of these terms apply to specific types of nonclinical evidence, and do not reflect clinical interpretation.
Classifying variants
Until the last few years, there were no standard systems for reporting the classification of genetic variants. Variants were generally reported in 3 categories: (1) pathogenic mutations that cause disease; (2) benign or neutral, and (3) VUS. Genetic testing laboratories tend to be conservative in classifying variants, wanting to avoid errors because of the important decisions that result from these conclusions. There has been considerable variation among researchers and laboratories regarding how much evidence should be required in order to classify a given variant. National and international organizations are attempting to develop standardized systems for interpreting and reporting such results.
A 5-tiered classification system that can be used to define clinical management of variants in hereditary cancer genes was proposed by the International Association for Research on Cancer (IARC) Working Group on Unclassified Genetic Variants, and has been applied to several syndromes in clinical cancer genetics (hereditary breast and ovarian cancer [HBOC], Lynch syndrome [LS], familial melanoma). When possible, each class is associated with a probability that a variant is pathogenic, derived from statistical studies that incorporate data from various independent sources that are important to disease pathology ( Table 1 ). The American College of Medical Genetics and Genomics (ACMG) and the Association for Molecular Pathology have jointly recommended guidelines that closely parallel the IARC scheme. Both systems include 5 classes of variants, and the IARC system links these to probabilities of pathogenicity (class 5, pathogenic, >99%; class 4, likely pathogenic, 95%–99%; class 3, uncertain, 5%–95%; class 2, likely neutral, 0.1%–5%; and class 1, neutral, <0.1%) and recommendations for management of HBOC and LS. It is hoped that the presence of guidelines from these major organizations will lead to standardization of the terminology and processes, which will assist in both clinical management and further research.
Class | Quantitative or Qualitative Probability of Pathogenicity | Clinical Testing of Relatives | Surveillance for Relatives | Research Testing |
---|---|---|---|---|
5 | >0.99 | Yes | Yes: high risk | No |
4 | 0.95–0.99 | Yes | Yes: high risk | Yes |
3 | 0.05–0.949 | No | Based on family history and so forth | Yes |
2 | 0.001–0.049 | No | Treat as no mutation detected | Yes |
1 | <0.001 | No | Treat as no mutation detected | No |
Variants in common and rare hereditary cancer syndromes
When interpreting genetic testing results, cancer geneticists must consider typical versus atypical presentations of the clinical syndrome in question. Atypical presentations could represent a variant of the common syndrome or a different condition that mimics the classic syndrome. The most commonly seen hereditary cancer syndromes are HBOC and LS; more than 50 other syndromes have been described. Genetic testing in these syndromes can be difficult to interpret because many cancers are commonly seen as sporadic cases, and it may not even be clear whether a hereditary syndrome is present in a family. New genetic technologies will identify many new genes that rarely cause a hereditary cancer syndrome, and many that confer intermediate (not high) risk for cancer, leading to new layers of uncertainty in variant interpretation. LS usually results from mutation to one of the 4 mismatch repair (MMR) genes, but can also be caused by an inherited deletion of the end of the EPCAM gene, just upstream of the MSH2 gene, which changes regulation of MSH2 and results in loss of MSH2 protein. The identification of other unusual examples of genetic mechanisms of pathogenicity is anticipated.
Definitive proof of hereditary cancer syndromes is sought in the DNA from normal cells, usually peripheral blood lymphocytes, looking for an inherited pathogenic mutation in a relevant gene. DNA changes that are inherited are present in every somatic cell of the body. For cancer to develop, the inherited gene mutation is followed by a second mutation in the copy of the gene in a relevant somatic cell (eg, colon, breast, ovary). In this clone of cells, both copies of the gene (eg, BRCA, MMR) are thus mutated. As a result, no normal proteins that the gene encodes are produced. The second abnormality abrogates the physiologic process that these proteins perform (eg, DNA MMR in LS, homologous recombination repair in HBOC). Evidence of these abnormal processes can sometimes be found in cells or tissues of patients.
VUS have been a large part of genetic testing since its inception in the 1990s. Their frequency varies by gene and syndrome. For HBOC, the fraction of BRCA1 and BRCA2 variants that are VUS has steadily decreased in recent years, but new, rare VUS in these genes are still identified with significant frequency. However, the frequency of VUS in intermediate-risk breast cancer genes is high. The database of MMR gene variants associated with LS includes more than 2000 VUS, about 20% to 30% of variants ( http://insight-group.org/variants/database/ ).
Interpreting genetic testing results
Some changes found in germline DNA almost certainly affect protein or cellular function; others almost certainly do not. Interpreting genetic testing results can be complicated, and potential results are not limited to positive or negative. In general, genetic testing can result in one of 3 possible interpretations of the molecular findings. These molecular interpretations must then be applied by clinicians to manage patients and their families properly.
Potential interpretations of molecular genetic testing for cancer predisposition syndromes are:
Pathogenic Variant Detected
There is an increased hereditary predisposition to cancer because of the DNA variant that can be clearly classified as pathogenic. A classification of pathogenic may mean that the variant has been seen before and is clearly associated with the corresponding condition. Some uncommon variants are easy for the laboratory to recognize as a mutation that disrupts the gene’s normal function. The most common types of variants for which pathogenicity is implied by the type of mutation involve a change in the DNA code that is predicted to result in the production of no protein or a very abnormal protein. These variants include (1) a nonsense mutation resulting in a stop codon; (2) a large deletion or insertion; (3) a frameshift deletion or insertion of a small number of nucleotides not divisible by 3; (4) some changes in which exons and introns meet and mRNA splicing is almost certainly altered, leading to an aberrant protein missing 1 or more of its critical parts. If any of these types of mutations are detected in a patient, the laboratory report will state that an inherited pathogenic mutation has been identified. Management of the patient can be planned accordingly, and testing of the patient’s relatives becomes an option.
Pathogenic DNA Variant Not Detected
The important caveat for this result is that it applies only to the genes examined, based on the specific technology applied. If only a variant that is known to be neutral is identified, this is considered a negative result. If no pathogenic variant is identified, this result must be interpreted cautiously; it does not automatically mean that there is no hereditary cancer risk present. Factors that affect interpretation of negative results are:
Who was tested?
Did the tested individual have cancer, and, if so, how likely is it that this individual carries the hereditary syndrome that was tested? If an unaffected relative was tested, then it is not possible to be certain that there is no pathogenic mutation present in others in the family. It is possible that no pathogenic mutation exists in the family, but it is also possible that other relatives carry a pathogenic mutation but this individual does not. Even if the tested individual has had cancer, the full personal and family history must be carefully evaluated. It is possible that the tested individual had a sporadic cancer but other relatives had a hereditary cancer (especially in the case of common cancers such as breast and colon). Several models have been developed to predict the likelihood of mutations in MMR or BRCA genes, but all of them have limitations. Each model represents a single tool that may or may not assist the surgical oncologist to navigate a difficult clinical scenario.
What test was done?
Which gene or genes was tested? It is possible that a mutation exists in a gene that was not tested. It is possible that not every relevant known gene was tested, and it is possible that a pathogenic mutation exists in a gene that is not yet known to be associated with hereditary cancer. Which parts of the gene were studied? It is possible that a variant exists in a regulatory region that was not tested. Some genetic technologies can miss large deletions. In addition, sometimes even the full coding region of a gene is not checked. For example, if a specific variant is known to be carried by a family, this specific variant can be sought at low cost without studying the rest of the gene. However, personal and family histories are critical in order to properly interpret the results of such site-specific testing.
Identification of a Variant of Uncertain Significance
This result usually means that further work is required by the clinical and/or research laboratories before the result can be used clinically. VUS can be in either regulatory or coding regions of the gene. A large body of scientific literature exists regarding how to interpret VUS, mostly on missense variants but also on some regulatory variants. Clinicians are not expected to perform these interpretations, but they should be aware of the principles regarding underlying variant analysis, and how clinicians can help advance the field for the benefit of patients and their families.
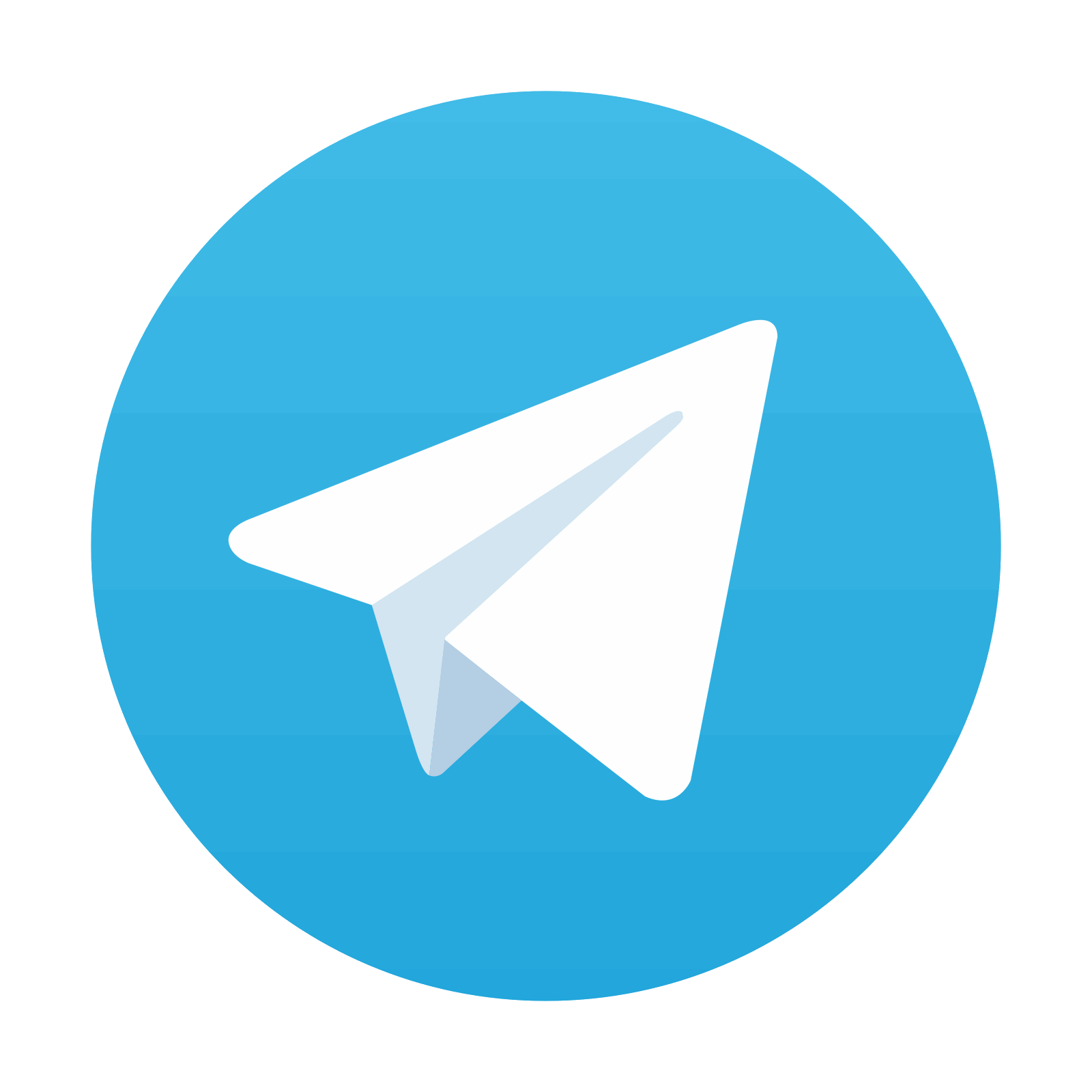
Stay updated, free articles. Join our Telegram channel

Full access? Get Clinical Tree
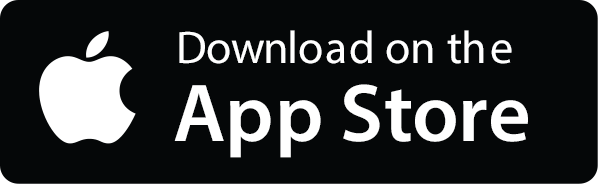
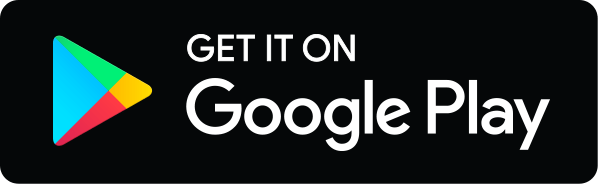