Role of ABC Transporters in Fluoropyrimidine-Based Chemotherapy Response
Anne T. Nies*,1; Tarek Magdy*,2; Matthias Schwab*,†; Ulrich M. Zanger* * Dr. Margarete Fischer-Bosch Institute of Clinical Pharmacology, Stuttgart, Germany
† Department of Clinical Pharmacology, Institute of Experimental and Clinical Pharmacology and Toxicology, University Hospital, Tübingen, Germany
1 Corresponding author: email address: anne.nies@ikp-stuttgart.de
2 Present address: Department of Pharmacology and Toxicology, Faculty of Pharmacy, Pharos University, Alexandria, Egypt.
Abstract
Since over 50 years, 5-fluorouracil (5-FU) is in use as backbone of chemotherapy treatment regimens for a wide range of cancers including colon, breast, and head and neck carcinomas. However, drug resistance and severe toxicities such as mucositis, diarrhea, neutropenia, and vomiting in up to 40% of treated patients often lead to dose limitation or treatment discontinuation. Because the oral bioavailability of 5-FU is unpredictable and highly variable, 5-FU is commonly administered intravenously. To overcome medical complications and inconvenience associated with intravenous administration, the oral prodrugs capecitabine and tegafur have been developed. Both fluoropyrimidines are metabolically converted intracellularly to 5-FU, which then needs metabolic activation to exert its damaging activity on RNA and DNA. The low response rates of 10–15% of 5-FU monotherapy can be improved by combination regimens of infusional 5-FU and leucovorin together with oxaliplatin (FOLFOX) or irinotecan (FOLFIRI), thereby increasing response rates to 30–40%. The impact of metabolizing enzymes in the development of fluoropyrimidine toxicity and resistance has been studied in great detail. In addition, membrane drug transporters, which are critical determinants of intracellular drug concentrations, may play a role in occurrence of toxicity and development of resistance against fluoropyrimidine-based therapy as well. This review therefore summarizes current knowledge on the role of drug transporters with particular focus on ATP-binding cassette transporters in fluoropyrimidine-based chemotherapy response.
1 Introduction: The Use of Fluoropyrimidines in Cancer Chemotherapy
1.1 Introduction
Since its first synthesis in 1957 by Charles Heidelberger (Heidelberger et al., 1957), the fluorinated pyrimidine analog fluorouracil (5-FU; Fig. 1A) is in use as backbone of chemotherapy treatment regimens for a variety of solid tumors including gastrointestinal carcinoma, breast carcinoma, and head and neck carcinoma (Longley, Harkin, & Johnston, 2003; Meyerhardt & Mayer, 2005; Wilson, Danenberg, Johnston, Lenz, & Ladner, 2014). Approximately, 2 million patients are annually treated with 5-FU worldwide (Ezzeldin & Diasio, 2004). Because the oral bioavailability is unpredictable and highly variable, 5-FU is commonly administered intravenously (Hoff, Cassidy, & Schmoll, 2001; Lamont & Schilsky, 1999). The low response rates of 10–15% of 5-FU monotherapy can be improved by combination regimens of infusional 5-FU and leucovorin together with oxaliplatin (FOLFOX, de Gramont et al., 2000) or irinotecan (FOLFIRI, Douillard et al., 2000), thereby increasing response rates to 30–40% (Longley et al., 2003). To overcome medical complications and inconvenience associated with intravenous administration, the oral prodrugs capecitabine and tegafur have been developed (Fig. 1A) (Hoff et al., 2001; Milano, Ferrero, & Francois, 2004). Both fluoropyrimidines are metabolically converted intracellularly to 5-FU, which then needs metabolic activation (Fig. 2) (Grem, 2000; Thorn et al., 2011; Wilson et al., 2014). There are two major clinical problems associated with the use of fluoropyrimidines: (i) severe toxicity including mucositis/stomatitis, diarrhea, neutropenia, nausea, vomiting, as well as hand and foot syndrome may occur in 10–40% of treated patients often leading to dose limitation or treatment discontinuation (Caudle et al., 2013; Hoff et al., 2001; Meta-Analysis Group In Cancer, 1998; Rosmarin et al., 2014) and (ii) development of resistance by the tumor leads to reduced efficacy as reflected by the low response rates of 5-FU monotherapy (Longley et al., 2003). The impact of metabolizing enzymes in the development of fluoropyrimidine toxicity and resistance has been studied in great detail and summarized previously (Amstutz, Froehlich, & Largiader, 2011; Rosmarin et al., 2014; Wilson et al., 2014). In addition, membrane drug transporters, which are critical determinants of intracellular drug concentrations (Degorter, Xia, Yang, & Kim, 2012; Giacomini et al., 2010), may play a role in occurrence of toxicity and development of resistance against fluoropyrimidine-based therapy as well. This review therefore aims to summarize current knowledge on the role of drug transporters with particular focus on ATP-binding cassette (ABC) transporters in fluoropyrimidine-based chemotherapy response.
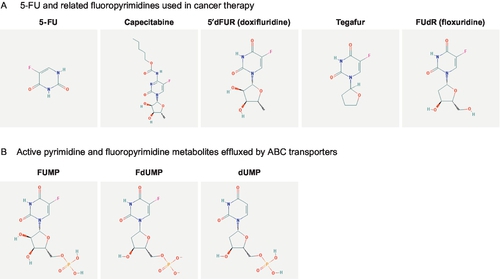
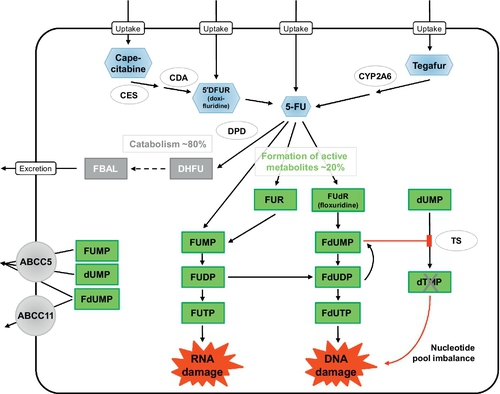
1.2 Pharmacokinetics of 5-FU
Following intravenous injection of 5-FU, the mean half-life of elimination from plasma is only about 13 min, with a range of 5–27 min, due to rapid metabolic elimination (Grem, 2000; Heggie, Sommadossi, Cross, Huster, & Diasio, 1987; Lamont & Schilsky, 1999). 5-FU readily penetrates into tissues and is also distributed into the extracellular space and cerebrospinal fluid. 5-FU plasma concentrations > 1 μM considered to be required for therapeutic efficacy are maintained for less than 2 h. Approximately 60–90% of an administered 5-FU dose is excreted into urine within 24 h, mainly as the metabolite fluoro-β-alanine (FBAL; Fig. 2) and only 5–10% is eliminated unchanged. Biliary excretion accounts for less than 3%.
1.3 Pathways of fluoropyrimidine metabolism and mechanism of action
Fluoropyrimidine metabolism involves many different enzyme reactions and intermediates (Grem, 2000; Lamont & Schilsky, 1999; Wilson et al., 2014) (Fig. 2). Following 5-FU administration, more than 80% of the administered dose is primarily catabolized by dihydropyrimidine dehydrogenase (DPD), the initial and rate-limiting enzyme for 5-FU catabolism (Ezzeldin & Diasio, 2004; Thorn et al., 2011). DPD catabolizes 5-FU to dihydrofluorouracil (DHFU), which is then degraded to fluoroureidopropionic acid and to FBAL by dihydropyrimidinase and β-ureidopropionase, respectively (Daher, Harris, & Diasio, 1990). The two oral prodrugs of 5-FU, capecitabine and tegafur, require metabolic activation by carboxylesterase (CES)/cytidine deaminase (CDA) and cytochrome P450 2A6 (CYP2A6), respectively, as initial steps to form 5-FU (Lamont & Schilsky, 1999; Thorn et al., 2011).
Only 1–3% of the administered 5-FU dose is anabolized intracellularly into active metabolites targeting RNA or DNA (Daher et al., 1990; Eliason & Megyeri, 2004; Longley et al., 2003; Thorn et al., 2011; Wilson et al., 2014) (Fig. 2). 5-FU is activated through thymidylate phosphorylase-catalyzed conversion to fluorodeoxyuridine (FUdR, floxuridine), which is then converted to fluorodeoxyuridine monophosphate (FdUMP) by thymidine kinase. FdUMP forms a stable ternary complex with thymidylate synthase (TS) and 5,10-methylene tetrahydrofolate, thereby inhibiting TS and DNA synthesis (Eliason & Megyeri, 2004). Alternatively, 5-FU can be activated through conversion to fluorouridine monophosphate (FUMP; Fig. 1B), either directly by orotate phosphoribosyltransferase, which uses phosphoribosyl pyrophosphate as a cofactor, or indirectly by sequential conversion to 5-fluouridine (FUR) and then to FUMP by the action of uridine phosphorylase and uridine kinase, respectively. FUMP is then phosphorylated by nucleoside monophosphate kinase to FUDP, which can be either phosphorylated by nucleoside diphosphate kinase to yield fluorouridine triphosphate (FUTP) or converted to fluorodeoxyuridine diphosphate (FdUDP) by ribonucleotide reductase. FUTP is incorporated into RNA thus disrupting RNA integrity and stability (Longley et al., 2003). FdUDP can be either dephosphorylated into FdUMP or phosphorylated into fluorodeoxyuridine triphosphate (FdUTP), which is incorporated into DNA, thereby leading to DNA damage (Eliason & Megyeri, 2004).
1.4 Limitations of fluoropyrimidine-based therapy: Toxicity and resistance
As it is the case for other chemotherapeutic drugs, fluoropyrimidines have a very narrow therapeutic index and toxicity during treatment is a major clinical challenge. Metabolizing enzymes, particularly DPD, and variants in the genes encoding these enzymes have been identified as clinically significant predictors of fluoropyrimidine toxicity (Amstutz et al., 2011; Loganayagam et al., 2013; Rosmarin et al., 2014; Schwab et al., 2008). Because DPD activity is clearly related to 5-FU clearance and 5-FU exposure is associated with its toxic effects, pharmacogenetics studies have led to dosing recommendations for fluoropyrimidines based on nonfunctional variants of DPD (Caudle et al., 2013).
In addition to adverse drug reactions, resistance to fluoropyrimidine-based therapy is also an important clinical issue. Resistance can develop by numerous mechanisms including decreased drug uptake, increased drug efflux, activation of detoxifying systems, activation of DNA repair mechanisms, and evasion of drug-induced apoptosis in the tumor cells (Baguley, 2010; Gillet & Gottesman, 2010; Moitra, Lou, & Dean, 2011). Reduced cellular uptake or increased cellular efflux mediated by membrane transporters may lead to resistance because these transporters prevent anticancer drugs from reaching target intracellular concentrations. An enhanced drug efflux is most often mediated by ATP-dependent ABC transporters such as the extensively studied MDR1 P-gp (encoded by the ABCB1 gene), multidrug resistance proteins (MRPs) of the ABCC subfamily (encoded by ABCC1, ABCC2, ABCC3, ABCC4, ABCC5, ABCC10, ABCC11) and BCRP (breast cancer resistance protein, encoded by ABCG2) (Deeley, Westlake, & Cole, 2006; Gillet & Gottesman, 2010; Keppler, 2011; Nies & Lang, 2014; Robey et al., 2009; Sarkadi, Homolya, Szakacs, & Varadi, 2006; Slot, Molinski, & Cole, 2011; Szakacs, Paterson, Ludwig, Booth-Genthe, & Gottesman, 2006). Uptake transporters of the solute carrier (SLC) superfamily may also accept drugs as substrates and may therefore contribute to cellular drug sensitivity (Hediger, Clemencon, Burrier, & Bruford, 2013).
2 Overview of Transporters Involved in Cellular Uptake and Efflux of Fluoropyrimidines and Their Metabolites
2.1 Uptake transporters
5-FU and its prodrugs capecitabine and tegafur require cellular uptake before they can be intracellularly converted to active metabolites causing cytotoxicity. Within the large superfamily of SLC transporters (Hediger et al., 2013), members of two families are discussed to mediate uptake of 5-FU. While organic anion transporter 2 (OAT2, encoded by the SLC22A7 gene) mediates uptake of 5-FU with high affinity (Michaelis–Menten constant Km 54 nM; Kobayashi et al., 2005), the two equilibrative nucleoside transporters (ENT) 1 and ENT2 (encoded by SLC29A1 and SLC29A2) transport 5-FU with Km values of 2.3 and 2.6 mM, respectively (Yao, Ng, Cass, Baldwin, & Young, 2011). ENT1 and ENT2 also transport 5′dFUR (synonyms: 5′-deoxy-5-fluorouridine, 5′-DFUR, or doxifluridine; Figs. 1A and 2) (Damaraju et al., 2013), which is a metabolite of capecitabine and also clinically used as an oral prodrug of 5-FU (Budman, 2000). The SLC28 family is also known to transport nucleoside and nucleobase drugs and the three members CNT1 (SLC28A1), CNT2 (SLC28A2), and CNT3 (SLC28A3) transport 5′dFUR as well (Errasti-Murugarren & Pastor-Anglada, 2010; Mata et al., 2001; Young, Yao, Baldwin, Cass, & Baldwin, 2013). Also, all three CNTs transport 2′-deoxy-5-fluorouridine (synonym: floxuridine; Fig. 1A), which is used like 5-FU as an intravenous agent (Power & Kemeny, 2009). No studies are currently available that have identified uptake transporters for tegafur.
2.2 Efflux transporters
From the 48 known human ABC transporters (Moitra & Dean, 2011), members of three subfamilies are generally considered as important for drug efflux from cells: (i) MDR1 P-glycoprotein (ABCB1) from the “B” subfamily, which was the first identified ABC drug efflux transporter and so far the most intensely characterized (Gottesman & Ling, 2006); (ii) several MRP transporters from the “C” subfamily (ABCC1, ABCC2, ABCC3, ABCC4, ABCC5, ABCC10, ABCC11) (Keppler, 2011; Nies & Lang, 2014; Slot et al., 2011) and (iii) ABCG2/BCRP from the “G” subfamily (Robey et al., 2009). Therefore, the vast majority of studies have investigated whether these transporters play a role in fluoropyrimidine-based therapy. Figure 3 shows predicted topology models of ABC transporters related to drug resistance.
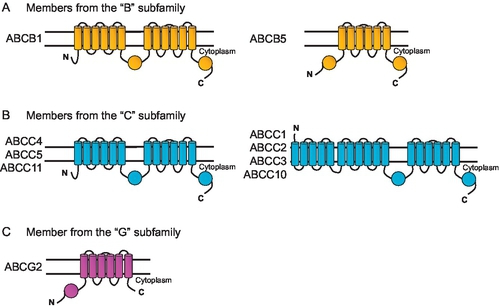
As detailed in the following paragraphs, different lines of evidence are available, which implicate several ABC transporters in fluoropyrimidine-based chemotherapy response, either based on in vitro studies using carcinoma cell lines (Section 3), or based on correlations of ABC transporter expression with resistance in clinical specimens of cancer patients (Section 4) or based on pharmacogenetics studies, in which genetic variants of ABC transporter genes have been correlated with treatment outcome and/or occurrence of toxicity (Section 5).
Of interest, no transporters have been identified so far that transport FBAL, the end product of 5-FU catabolism (Fig. 2).
2.3 Other transport mechanisms
Efflux mechanisms for fluoropyrimidines independent from ABC efflux transporters may exist as well. Of note, the transcriptional status of the Cu2 +-transporting ATPase, ATP7B, together with three other key genes (TS, MORF-related gene X, Bcl2-antagonist/killer) in single colon cancer cells from patients was predictive for the response to 5-FU (Pezo et al., 2008). In an in vitro study using the colorectal cancer cell line LS180, components of the FOLFOX regime were tested for their ability to affect drug transporter expression (Theile, Grebhardt, Haefeli, & Weiss, 2009). Here, 5-FU significantly suppressed ATP7B expression, which was suggested to contribute to the synergistic action of 5-FU, leucovorin, and oxaliplatin of the FOLFOX combination.
In addition, in a systematic approach correlating the expression of transporters and channels to drug resistance of 119 standard anticancer drugs in 60 human cancer cell lines of the National Cancer Institute (NCI-60 panel) further transporters and channels were identified to correlate with 5-FU resistance (e.g., SLC23A2, ATP1B3, ATP2A1, ATP2A4), which have not been explored yet (Huang et al., 2004).
3 Cell-Based Evidence for the Role of ABC Transporters in 5-FU Pathways
Most studies have investigated ABC transporters that have been previously identified to be involved in drug efflux. From these cell-based studies, analyzing whether expression of a specific ABC transporter confers resistance to 5-FU or whether 5-FU affects expression of ABC transporters in carcinoma cells, ABCB5, ABCC5, and ABCC11 have emerged as transporters with relevance for cellular resistance against 5-FU.
3.1 ABCB1
ABCB1 confers resistance to a large number of structurally diverse drugs (Ambudkar et al., 1999; Szakacs et al., 2006), however not to 5-FU (Mechetner & Roninson, 1992). Nevertheless, several studies using gastrointestinal, breast, or esophageal carcinoma cell lines report that ABCB1 mRNA levels are correlated with resistance against 5-FU (Minegaki, Takara, Hamaguchi, Tsujimoto, & Nishiguchi, 2013; Pandey, Chaube, & Bhat, 2011; Takechi, Koizumi, Tsujimoto, & Fukushima, 2001). Since these studies also report altered expression of other drug transporters (e.g., ABCC2) and metabolizing enzymes, it remains questionable whether 5-FU resistance can indeed be attributed to ABCB1 expression.
3.2 ABCB5
ABCB5 was initially identified to mediate doxorubicin resistance in human malignant melanoma (Frank et al., 2005). ABCB5 is apparently also involved in chemoresistance against 5-FU because shRNA-mediated gene silencing of ABCB5 endogenously expressed in human colorectal cancer cells decreased chemoresistance to 5-FU (Wilson et al., 2011).
3.3 ABCC1
ABCC1 also confers resistance to a large number of structurally diverse drugs such as anthracyclines, camptothecins, and Vinca alkaloids (Cole, 2013; Deeley et al., 2006; Nies & Lang, 2014), but not to 5-FU (Mirski, Gerlach, & Cole, 1987). Correlation analyses indicated that gene expression of ABCC1 in gastrointestinal cancer cell lines may be related to 5-FU resistance (Nishiyama et al., 1999). However, in another study a negative correlation of ABCC1 expression and 5-FU resistance was observed in a panel of 14 human cancer cell lines (Bracht, Liebeke, Ritter, Grunert, & Bednarski, 2007). Yet another study reported no correlation between ABCC1 expression and 5-FU sensitivity in head and neck carcinoma cells (Tsuzuki et al., 1998). Thus, it remains open whether ABCC1 indeed contributes to 5-FU resistance.
3.4 ABCC2
Similar to ABCB1 and ABCC1, ABCC2 also confers resistance to a number of different drugs including cisplatin, anthracyclines, and Vinca alkaloids (Cui et al., 1999; Keppler, 2011; Nies, 2013; Nies & Lang, 2014). However, in studies using human hepatoma HepG2 cells, in which ABCC2 was silenced by ABCC2 antisense cDNA, resistance against cisplatin and doxorubicin was decreased as expected, however, there was no effect on resistance against 5-FU (Koike et al., 1997). Therefore, ABCC2 is apparently not involved in cellular resistance to 5-FU.
3.5 ABCC4
ABCC4 confers resistance to several anticancer drugs such as camptothecins, topotecan, and 6-mercaptopurine, but not to 5-FU (Nies & Lang, 2014; Tian et al., 2005). Of note, ABCC4, together with ABCC3 and ABCC5, is upregulated on mRNA and protein level in pancreatic carcinoma cell lines treated with 5-FU (Hagmann, Jesnowski, Faissner, Guo, & Lohr, 2009). Whether this upregulation of ABCC4 contributes to 5-FU resistance is currently unclear.
3.6 ABCC5
The discovery of ABCC5 as an efflux transporter for cyclic nucleotides and nucleotide analogs (Jedlitschky, Burchell, & Keppler, 2000; Wijnholds et al., 2000) suggested that ABCC5 also confers resistance to 5-FU. However, this could not be shown in the initial studies by Wijnholds et al. (2000). On reexamination of the role of 5-FU using ABCC5-transfected cells with high expression levels of ABCC5, it could be demonstrated that ABCC5 confers resistance to 5-FU (Pratt et al., 2005). This was further supported by subsequent work using pancreatic cancer cell lines (Hagmann et al., 2009; Nambaru et al., 2011). siRNA-mediated silencing of endogenous ABCC5 expression significantly increased cellular cytotoxicity of 5-FU and enhanced accumulation of 5-FU and its metabolites.
When inside–out membrane vesicles isolated from cells expressing recombinant ABCC5 were used, which is a generally accepted system to study substrate specificity and affinity of ABC transporters (Brouwer et al., 2013), 5-FU could not be identified as ABCC5 substrate; instead, the fluoropyrimidine metabolites FUMP, dUMP, and FdUMP (Fig. 1B) were identified as substrates transported by ABCC5 (Pratt et al., 2005).
3.7 ABCC11
ABCC11 has been identified as a cyclic nucleotide efflux transporter and as a resistance factor for fluoropyrimidines (Guo et al., 2003). Similar to ABCC5, ABCC11 is not able to transport 5-FU, but rather its metabolite FdUMP (Fig. 1B) is transported (Guo et al., 2003). By in silico modeling and ligand docking, putative binding sites for FdUMP have been recently localized in ABCC11 (Honorat et al., 2013). A role of ABCC11 in cellular resistance to 5-FU is supported by studies using the 5-FU resistant small cell cancer cell subline PC-6/23-26. Here, siRNA-mediated silencing of endogenous ABCC11 expression led to decreased resistance against 5-FU (Oguri et al., 2007).
3.8 ABCG2
Overexpression of ABCG2 renders cells resistant to several anticancer drugs such as mitoxantrone, anthracyclines, methotrexate, and topotecan (Dean, Fojo, & Bates, 2005; Robey, Ierano, Zhan, & Bates, 2011). However, ABCG2 has no effect on resistance to 5-FU in ABCG2-transfected cells (Bram et al., 2006).
4 Association of ABC Transporter Expression with Resistance in Clinical Specimens
In addition to the above-described studies analyzing in vitro chemoresistance, several studies also investigated whether expression of ABC transporters is associated with resistance to 5-FU using clinical specimens from patients. In general, these studies are difficult to interpret since patients are usually not treated with 5-FU monotherapy but in combination with other anticancer drugs. Moreover, in most cases it is not a single ABC transporter whose expression is investigated (or affected) but rather a pattern of transporters or other genes known to be involved in resistance to 5-FU. Therefore, it appears to be rather difficult to predict outcome of fluoropyrimidine-based therapy based on individual markers such as ABC transporters, and more complex methods are required to improve response rates.
4.1 ABCB1
In one study, expression of ABCB1 protein and of lung cancer associated resistance protein (LRP, the major vault protein) was investigated in breast cancer specimens from patients who had received standard 5-FU, doxorubicin, and cyclophosphamide (FAC) chemotherapy. In a logistic regression multivariate model, several factors including histology, Ki67 labeling, and the combination of LRP and ABCB1 positive immune reaction in clinical specimens were identified as independent predictors of axillary node invasion at the time of rescue mastectomy (Schneider et al., 2001). In another study, ABCB1 expression increased on mRNA and protein level in esophageal carcinoma specimens after patients had received epirubicin, cisplatin, and 5-FU (ECF) chemotherapy (Di Nicolantonio et al., 2005).
4.2 ABCB5
Together with the identification of ABCB5 as being involved in cellular resistance to 5-FU, increased levels of ABCB5 protein were also observed in clinical specimens from colorectal cancer patients who had residual disease after 5-FU-based chemoradiation therapy (Wilson et al., 2011). ABCB5 may therefore be a novel molecular marker of therapy-refractory tumor cells in colon cancer patients.
4.3 ABCC1
ABCC1 protein expression was associated with an increased risk for failure in 259 breast cancer patients receiving cyclophosphamide, methotrexate, and 5-FU (CMF) therapy (Nooter et al., 1997). Here, ABCC1 was frequently overexpressed in primary breast cancer specimens and suggested a prognostic significance in a certain subgroup of patients. These findings were corroborated by a subsequent study with 516 breast cancer patients, in which ABCC1 expression appeared to play an important role in the clinical resistance to adjuvant CMF chemotherapy as well (Filipits et al., 2005). In esophageal adenocarcinoma patients, high pretherapeutic TS and ABCC1, but not ABCB1, protein levels were associated with nonresponse to cisplatin and 5-FU-based neoadjuvant chemotherapy (Langer et al., 2010). On the contrary, resistance to anticancer drugs including 5-FU in head and neck squamous cell carcinoma was apparently not dependent on ABCC1 protein expression (Tsuzuki et al., 1998). In addition, patients with high pretherapeutic ABCC1 levels had a survival benefit after surgery of Barrett carcinoma and cisplatin and 5-FU therapy with or without additional paclitaxel (Langer et al., 2005).
4.4 ABCG2
ABCG2 expression has been associated with 5-FU resistance using clinical breast cancer tissue specimens (Wang, Wang, Yuan, & Guo, 2013) and using specimens from patients with metastatic colorectal cancer receiving first-line FOLFOX therapy (Lin et al., 2013).
4.5 Combined analyses of ABC transporters
In a pilot study, mRNA expression of selected drug resistance genes (ABCB1, ABCC1, ABCC2, ABCG2, LRP) was determined in primary operable breast cancer and ABCB1 was found to be inversely correlated with the efficacy of first-line chemotherapy (CMF or anthracycline-based FAC/FEC therapy), i.e., a high expression level of ABCB1 was a significant predictor of poor prognosis for patients with advanced disease (Burger et al., 2003). In subsequent studies, comprehensive gene expression profiling of all 48 ABC transporters was performed in samples from patients with breast cancer before and after treatment with paclitaxel/FEC (5-FU, epirubicin, and cyclophosphamide) neoadjuvant chemotherapy (Park et al., 2006) and in samples from patients with colorectal cancer before the first-line treatment with a 5-FU-containing regimen (Hlavata et al., 2012). Both studies revealed an unique pattern of up- or downregulated ABC transporter expression. In the breast cancer patients, several ABC transporters, including ABCC5, ABCA12, ABCA1, ABCC13, ABCB6 and ABCC11, were significantly upregulated in the patients with residual disease (Park et al., 2006). In the colon cancer patients, the disease-free interval of patients treated with adjuvant chemotherapy was significantly shorter in patients with low transcript levels of ABCA7, ABCA13, ABCB4, ABCC11 and ABCD4 (Hlavata et al., 2012). Moreover, the authors suggest that ABCC11 may be a promising candidate marker for a validation study on 5-FU therapy outcome in colon cancer patients. On the contrary, in patients with gastric cancer treated with 5-FU and doxorubicin-based adjuvant chemotherapy high expression of ABCB1, ABCC1, and TS protein did not predict poor prognosis of patients (Choi et al., 2002).
5 Genotype–Phenotype Correlations of ABC Transporters and Fluoropyrimidine-Based Therapy Response
Pharmacogenetic analyses are commonly used to identify genes involved in therapy response. Using this approach, variants in the genes encoding DPD (encoded by DPYD), CDA (CDA), and TS (TYMS) provide strong evidence that these metabolizing enzymes are significant predictors of fluoropyrimidine toxicity (Amstutz et al., 2011; Loganayagam et al., 2013; Rosmarin et al., 2014; Schwab et al., 2008). In addition to these, ABC transporters may be candidate genes of toxicity/adverse drug reactions and efficacy as well. Table 1 summarizes current knowledge of variants in ABCB1, ABCC1, ABCC2, and ABCC11 genes and their effect on efficacy or toxicity. For more detailed discussions on pharmacogenomics of these transporters, the reader is referred to recent reviews (e.g., Cascorbi, 2011; Chinn & Kroetz, 2007; Franke, Gardner, & Sparreboom, 2010; Kerb, 2006; Nies & Lang, 2014; Noguchi, Katayama, & Sugimoto, 2014). Only one example should be mentioned here: one genetic variant in the ABCB1 gene, the synonymous SNP rs1045642 (c.3435C>T), has received considerable attention because it was associated with altered P-gp activity and function (Hoffmeyer et al., 2000). ABCB1 c.3435C>T is part of a common haplotype with c.1236C>T (rs1128503) and c.2677G>T (rs2032582) (Kim et al., 2001). Despite discordant results, numerous studies reported that c.3435C>T (alone or in combination) is associated with altered mRNA expression, in vitro substrate efflux from lymphocytes, pharmacokinetics of drug substrates in clinical studies, and drug response in HIV or epilepsy patients (Chinn & Kroetz, 2007; Kerb & Schwab, 2010). The synonymous variant apparently alters kinetics of protein translation leading to a change in protein folding and function (Fung et al., 2014; Kimchi-Sarfaty et al., 2007).
Table 1
Genotype–phenotype correlations of genetic variants in ABC transporter genes and response to fluoropyrimidine-based therapy
Gene | Variant ID Genomic location | Effect on | Tumor type | N | Observed associations | References |
ABCB1 | rs1045642 g.87509329A>G | Toxicity/ADR | Colorectal | 67 | Genotype GG associated with decreased risk of diarrhea in patients treated with 5-FU as compared to genotype AA When patients treated with irinotecan/5-FU were deducted from the analysis, the association was no longer significant, likely due to sample size (n = 50) No significant association with risk of neutropenia or hand-foot syndrome | Gonzalez-Haba et al. (2010) |
ABCB1 | rs1045642 g.87509329A>G | Toxicity/ADR | Colorectal | 74 | Genotype GG associated with increased risk of hand-foot syndrome in patients treated with capecitabine as compared to genotype AA No significant association with risk of neutropenia or diarrhea | Gonzalez-Haba et al. (2010) |
ABCB1 | rs1045642 g.87509329A>G | Toxicity/ADR | Colorectal | 150 | Genotype AA associated with higher risk of early toxicity in patients treated with irinotecan/5-FU | Glimelius et al. (2011) |
ABCB1 | rs1045642 g.87509329A>G | Toxicity/ADR | Gastric | 43 | Genotypes AG + AA associated with mucositis in patients treated with paclitaxel, 5-FU, and leucovorin | Chang et al. (2010) |
ABCB1 | rs1045642 g.87509329A>G | Efficacy | Esophageal | 262 | Genotypes AA + AG associated with decreased risk of lymph node metastases in patients treated with cisplatin and 5-FU and radiotherapy as compared to genotype GG | Narumiya et al. (2011) |
ABCB1 | rs1045642 g.87509329A>G | Efficacy | Esophageal | 146 | Genotype GG associated with decreased survival rate in patients treated with cisplatin and 5-FU and radiotherapy as compared to genotypes AA + AG | Narumiya et al. (2011) |
ABCB1 | rs1045642 g.87509329A>G | Efficacy | Gastric | 100 | Genotype GG associated with longer progression free survival in patients treated with 5-FU/fluoropyrimidine with or without cisplatin as compared to genotypes GA + AA | Shitara et al. (2010) |
ABCB1 | rs1045642 g.87509329A>G | Efficacy | Breast | 96 | Patients with genotype AA had higher chance of responding to 5-FU, doxorubicin, and cyclophosphamide treatment | George et al. (2009) |
ABCB1 | rs1045642 g.87509329A>G | Efficacy | Colorectal | 95 | Survival probability of patients treated with 5-FU/leucovorin and with G-allele higher than among patients without this allele | Panczyk et al. (2009) |
ABCB1 | rs2032582 g.87531302A>C | Toxicity/ADR | Colorectal | 74 | Genotype CC associated with increased risk of hand-foot syndrome in patients treated with capecitabine as compared to genotype AA No signficant association for risk of neutropenia or diarrhea | Gonzalez-Haba et al. (2010) |
ABCB1 | rs2032582 g.87531302A>C | Toxicity/ADR | Gastric | 43 | Genotype GG associated with less severe diarrhea in patients treated with paclitaxel, 5-FU, and leucovorin | Chang et al. (2010) |
ABCB1 | rs2032582 g.87531302A>C | Efficacy | Colorectal | 250 | Genotype CC associated with overall survival in patients treated with 5-FU, irinotecan and leucovorin as compared to genotypes CT + TT | De et al. (2013) |
ABCB1 | rs1128503 g.87550285A>G | Toxicity/ADR | Colorectal | 74 | Genotype AA associated with decreased risk of neutropenia (grade II or above) and hand-foot syndrome in patients treated with capecitabine as compared to genotype GG When patients treated with both irinotecan and capecitabine were deducted from the analysis, the association with neutropenia remained significant, but not with hand-foot syndrome No significant association with risk of diarrhea | Gonzalez-Haba et al. (2010) |
ABCB1 | Haplotype: rs1045642 + rs1128503 + rs2032582 | Efficacy | Colorectal | 150 | Carriers of the haplotype rs1045642 (A-allele)/rs1128503 (A-allele)/rs2032582 (A-allele) responded to treatment less frequently and survived shorter time when treated with irinotecan/5-FU | Glimelius et al. (2011) |
ABCC1 | rs2074087 g.16090375C>G | Toxicity/ADR | Colorectal | 144 | C-allele associated with decreased risk of neurotoxicity syndromes grade ≥ 2 in patients treated with 5-FU, leucovorin and oxaliplatin as compared to G-allele | Cecchin et al. (2013) |
ABCC1 | rs4148350 g.16076620G>T | Toxicity/ADR | Breast | 1012 | T-allele associated with febrile neutropenia as compared to homozygous carriers of the G-allele in patients treated with 5-FU, epirubicin, and cyclophosphamide | Vulsteke et al. (2013) |
ABCC2 | rs2273697 g.99804058G>A | Efficacy | Colorectal | 50 | Genotypes GA + AA associated with higher overall survival in patients treated with 5-FU, leucovorin, and oxaliplatin as compared to genotype GG | Mirakhorli et al. (2013) |
ABCC2 | rs717620 g.99782821C>T | Efficacy | Colorectal | 61 | Genotype CC associated with longer progression free survival in patients treated with 5-FU, leucovorin, and irinotecan as compared to genotypes CT + TT | Akiyama et al. (2012) |
ABCC2 | rs717620 g.99782821C>T | Toxicity/ADR | Colorectal | 144 | T-allele associated with increased risk of neurotoxicity syndromes grade ≥ 2 in patients treated with 5-FU, leucovorin, and oxaliplatin | Cecchin et al. (2013) |
ABCC2 | rs3740066 g.99844450C>T | Toxicity/ADR | Colorectal | 144 | T-allele associated with increased risk of neurotoxicity syndromes grade ≥ 2 in patients treated with 5-FU, leucovorin, and oxaliplatin | Cecchin et al. (2013) |
ABCC2 | rs4148396 g.99832187 T>C | Toxicity/ADR | Colorectal | 144 | T-allele associated with increased risk of neurotoxicity syndromes grade ≥ 2 in patients treated with 5-FU, leucovorin, and oxaliplatin | Cecchin et al. (2013) |
ABCC2 | rs1885301 g.99781296A>G | Toxicity/ADR | Colorectal | 144 | A-allele associated with increased risk of neurotoxicity syndromes grade ≥ 2 in patients treated with 5-FU, leucovorin, and oxaliplatin | Cecchin et al. (2013) |
ABCC11 | rs7194667 g.48208987T>G | Toxicity/ADR | Gastrointestinal or breast | 672 | Genotypes GG + GT associated with increased risk of leukopenia in patients treated with 5-FU monotherapy | Magdy et al. (2013) |
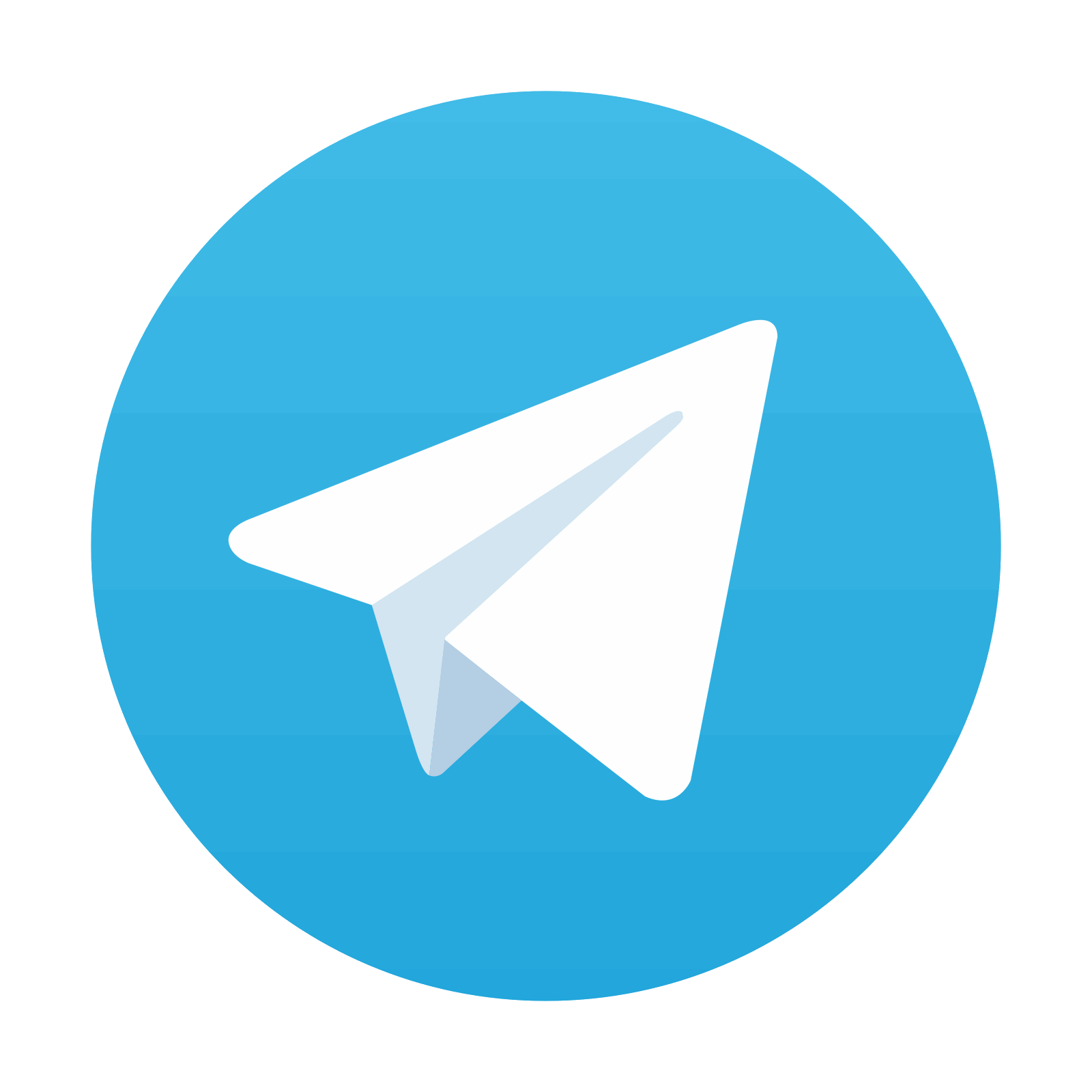
Stay updated, free articles. Join our Telegram channel

Full access? Get Clinical Tree
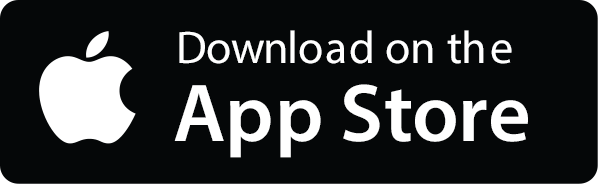
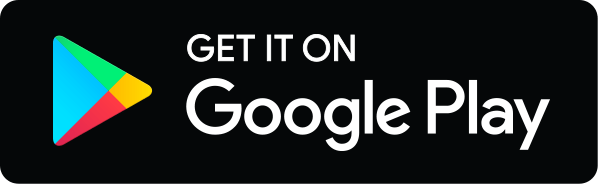