4.3 Clinical Implication
There is no specific clinical feature correlated with FV Leiden. The main effect of this mutation is the increase of thrombotic risk, by 10-fold in heterozygotes and by 30–140-fold in homozygotes. Heterozygous carriers have a 10 % lifetime probability of VTE. Homozygotes may experience one or more episodes of VTE over their lifetime and are prone to present VTE at a younger age [8]. FV Leiden could be found in about 25 % of patients presenting with a first idiopathic episode of VTE and up to 50 % of cases with recurrent VTE or concomitant use of estrogens [8].
Although the main manifestation of the thrombotic effect of FV Leiden is deep venous thrombosis (DVT) of the lower limbs, the mutation could be also found in the upper limb thrombosis (in 11.8–20 % of these cases) and less frequent in thrombosis with unusual location such as cerebral, retinal, hepatic, ovarian, and renal veins [8, 22]. This mutation may be associated with superficial thrombophlebitis, but the association is weak, showing that other factors should be taken in consideration in the etiopathogenic background [23].
Interestingly, considering DVT and pulmonary embolism (PE) as manifestations of the same disease- VTE-, FV Leiden is associated predominantly with DVT, with a relative risk of 4.5, whereas the relative risk of PE is 1.7; this is known in the literature as FV Leiden paradox [24]. Some studies show that other factors associated with a higher risk for DVT than for PE also act by increased APC resistance (oral contraceptives or hormonal replacement therapy, pregnancy, puerperium) [24, 25]. Several theoretical mechanisms proposed to explain FV Leiden paradox, such as the location of the thrombus (proximal versus distal veins) and the difference in the thrombus density were recently studied, but with inconsistent results. The hypothesis about the difference in the growth speed of the thrombus was also tested. The mouse models detected a faster growing thrombus in FV Leiden carriers and human studies showed a longer period of time between the occurrence of PE and the diagnosis. However, further studies are required to confirm these findings as pathogenetic background of FV Leiden paradox [26].
The role of FV Leiden in arterial thrombosis (manifested with acute myocardial infarction, stroke, peripheral arterial disease) is less clarified; some studies have shown a weak association between FV Leiden and arterial ischemic events, which could be slightly increased in women and in patients younger than 55 years old [27, 28].
The risk of recurrent VTE is not well established in patients with this mutation: LETS showed a 5-year cumulative recurrence rate of 12.5 % in homozygotes not receiving long-term anticoagulation and a meta-analysis including 3104 patients with VTE found a slightly increased risk in heterozygotes; other studies showed no risk of recurrence in homozygotes as well as in double heterozygous carriers of FV Leiden and prothrombin G20210A mutation [29–31]. The concomitant association between heterozygosity for FV Leiden and other thrombophilic factors may influence the risk of recurrent thrombosis: thus, some studies show an increase of the risk by three to nine-fold when heterozygosity for prothrombin G20210A was also present (in contradiction with previously mentioned data) and, by four- to five-times when hyperhomocysteinemia was associated [8].
The impact of FV Leiden on VTE associated with cancer was recently shown by the results of CATS (Vienna Cancer And Thrombosis Study): the probability of the occurrence of VTE is 13 % in patients with cancer who are also carriers of FV Leiden mutation versus 5.7 % in those with malignancy but without FV Leiden [32]. Previous studies offered contradictory results. This finding may be helpful in the assessment of the individual thrombotic risk in these cases.
Other pathological conditions associated with FV Leiden include the pregnancy complications, with a risk increased by two-to three-fold compared to non-carriers of the mutation: pregnancy loss, preeclampsia, intrauterine growth restriction and placental abruption. An episode of VTE during pregnancy may occur in almost half of the carriers of FV Leiden [33].
Usually, 50 % of VTE events in patients with FV Leiden are triggered by the intervention of an acquired risk factor, the most common being the pregnancy and the use of oral contraception in women [34]. The concomitant presence of another genetic thrombophilic factor will increase supplementary the thrombotic risk, acting synergistically: thus, the double heterozygosity for FV Leiden and prothrombin G20210A will lead to a 20-fold increase in relative risk, whereas the association between FV Leiden allele and hyperhomocysteinemia results in 21.8-fold increase, in a similar manner as for the recurrent thrombotic events previously presented [35, 36].
4.4 Practical Aspects
The American College of Medical Genetics Consensus Statement on Factor V Leiden recommends for detection a DNA-based genotyping test or a FV Leiden-specific functional assay. If the functional assay shows a positive result, a further DNA test is required to confirm it and to identify homozygosity or heterozygosity. The molecular test is recommended for the relatives of a carrier of FV Leiden as well as in cases on heparin therapy or with known lupus anticoagulant [37].
The APC resistance assay analyses the activated partial thromboplastin time (aPTT) in plasma in the presence and absence of a standardized amount of exogenous APC. In case of APC resistance, the response to APC will be a minimal prolongation of the aPTT. The test cannot be used in conditions associated with a prolonged aPTT, such as patients receiving oral anticoagulation or with a lupus anticoagulant. The test cannot distinguish well between heterozygotes and normal cases and measures only the resistance to APC no matter its cause. Thus, the modified assays were developed, which include a pre-dilution in FV-deficient plasma before the addition of APC and calcium, to reduce the confounding factors that might affect the aPTT. These tests could be used in patients receiving anticoagulation, but not in the presence of lupus anticoagulants which may offer false positive results. The chromogenic assay shows the APC resistance APC expressed as a ratio of the factor Xa amidolytic activity without APC to its factor Xa activity with the addition of APC. Russell Viper Venom Assay is another screening test based on the same principle and showing the ratio between dilute Russell Viper Venom Time with and without addition of the venom. For a better discrimination between FV Leiden mutation and protein C deficiency, samples could be pre-diluted with factor V deficient plasma [38].
PCR (Polymerase Chain Reaction) is the method used for the definitive diagnosis of FV Leiden, detecting wild-type or mutant allele (homozygous wild-type, homozygous mutant, heterozygous).
The Consensus Statement indicates the test in at least one of the following situations [37]:
Age <50, any venous thrombosis.
Venous thrombosis in unusual sites (such as hepatic, mesenteric, and cerebral veins).
Recurrent venous thrombosis.
Venous thrombosis and a strong family history of thrombotic disease.
Venous thrombosis in pregnant women or women taking oral contraceptives.
Relatives of individuals with venous thrombosis under age 50.
Myocardial infarction in female smokers under age 50.
FV Leiden could be also tested in female relatives of a patient with this mutation, before considering the pregnancy or the use of oral contraception as well as in women with recurrent pregnancy loss or unexplained pregnancy complications to manage better future pregnancies [37]. Otherwise, the prenatal testing and newborn screening are not recommended by routine. In young patients with acute arterial thrombosis in the absence of the classical risk factors, testing may be also worthwhile [37, 39].
The guidelines issued in 2011 are against FV Leiden and prothrombin G20210A routine testing in adults with idiopathic VTE (for the secondary prophylaxis of recurrent thrombosis) as well as in asymptomatic adult relatives of patients with VTE carrying one of these mutations (for the primary anticoagulation prophylaxis against the first VTE) [40].
5 Prothrombin G20210A
5.1 Gene Mutation (Gene Symbol/ Chromosome Locus: Factor II: F2; 11p11-q12)
Prothrombin is produced by the liver and is activated into thrombin by activated factor X. Thrombin plays a key role in coagulation: it activates platelets as well as factors V, VIII and XI, cleaves fibrinogen to fibrin, modulates fibrinolysis by binding to thrombomodulin. Prothrombin (FII) G20210A mutation, identified by Poort et al. in 1996, is the result of the substitution of guanine to adenine at position 20210, present at 3′ untranslated region of prothrombin gene, leading to an increase of mRNA accumulation and increased prothrombin levels by 133 %. As a consequence, the level of thrombin activatable fibrinolysis inhibitor may increase, contributing to the hypercoagulability state [41]. This mutation represents the second most common cause of inherited thrombophilia.
Recently, other mutations in prothrombin gene were described, such as the substitution of arginine to leucine at position 596 (p.Arg596Leu) (called prothrombin Yukuhashi) associated with an increased trombotic risk [42].
5.2 Prevalence in Healthy Populations and VTE
Similar to FV Leiden, the prothrombin G20210A mutation is found with the highest prevalence in Caucasians, suggesting a single event in a Caucasian ancestor occurring around 24 thousand years ago after the divergence of Africans from Non-Africans and Caucasoids from Mongoloids [41]. The prevalence in general population is 1–4 % with geographical differences: the prevalence in Southern Europe (3 %) is nearly twice as in Northern Europe (1.7 %) whereas in individuals from Asian and African descent, in native Americans and in Australians is very rare [43]. In Mediterranean region, the prevalence is between 1 and 12 % in general population, respective 3–24 % in VTE patients, suggesting the possibility that the mutation occurred here first and then spread to Europe [41]. The prevalence of prothrombin G20210A in VTE cases shows the same geographical differences: 6.5 % in North Europe, 2.7–17.2 % in South Europe, 3.2–9.7 % in Caucasians in USA, 0 in India [41].
The mutation was found in 4.3–11.3 % of unselected cases diagnosed with a first episode of DVT and in 7.9–24.1 % of patients with a personal or family history of VTE or thrombophilia [44].
5.3 Clinical Implication
The main manifestation of this mutation is correlated with the prothrombotic effect, the risk of VTE being increased by two to four-fold [41].
It was shown that children with prothrombin G20210A mutation present predominantly arterial thrombosis and the central nervous system involvement, unlike those with FV Leiden or deficiencies of proteins C and S; when VTE occur, additional risk factors are usually present [45].
Although the carriers of prothrombin G20210A mutation may present a five-fold increase in the risk of a first episode of myocardial infarction, the arterial thrombotic risk is generally low and is manifested before the age of 50 [46].
The episodes of VTE in carriers of prothrombin G20210A mutation younger than 30 years old tend to occur with a higher annual incidence than in carriers of FV Leiden (0.44 % versus 0.25 %) [46]. The risk of VTE is more than double in carriers of prothrombin mutation with a positive family history and may be higher in homozygotes [46].
The risk for recurrent spontaneous VTE in individuals carrying this mutation is not significantly increased, no matter if the first episode was spontaneous or secondary [47].
The risk of VTE is increased in the presence of other acquired risk factors; thus, carriers of prothrombin G20210A mutation taking oral contraceptives may present a seven-fold increased risk, and, respective, 17-fold higher risk in case of concomitant heterozygosity for FV Leiden [35].
Prothrombin G20210A mutation could be found in about 17 % of pregnant women with VTE [48]. However, a family study showed that prothrombin mutation is not a risk factor for pregnancy adverse outcome in female relatives [46]. Although numerous case-control studies have shown adverse obstetric events associated with inherited thrombophilia, including prothrombin mutation, a recent prospective study conducted by Eunice Kennedy Shriver National Institute of Child Health and Human Development, which enrolled more than 4000 women in a low risk cohort, failed to prove a link between pregnancy loss, preeclampsia, placental abruption, intrauterine growth restriction and prothrombin mutation [48]. This study suggests the lack of benefit from thromboprophylaxis in women with thrombophilia, regardless the previous pregnancy complications, as shown by another nonrandomized cohort [49].
5.4 Practical Aspects
The indications for prothrombin mutation testing are the same as previously presented regarding FV Leiden [37, 39, 40].
The mutation is detected using PCR; the amplification of genomic DNA is followed by a number of methods to identify the mutation: the use of restriction enzymes (Mn/I), DNA microarrays, real-time PCR.
6 Protein C (PC) Deficiency
6.1 Gene Mutations
PC is a vitamin K-dependent glycoprotein produced in the liver. It circulates in the blood as an inactive form and combines with thrombomodulin producing activated PC (aPC). The aPC interacts with PS at the platelets level and degrades factor Va and VIIIa which are required for factor X activation and thrombin generation [50]. The deficiency of aPC will create a prothrombotic state, manifested especially in venous circulation. This may occur due to genetic and acquired causes.
The PC gene, located on the long arm of chromosome 2, present numerous mutations leading to a quantitative (type I) or functional (type II) deficiency of PC [50]. The mechanism of inheritance is usually autosomal dominant. Heterozygotes with type I deficiency present half levels of aPC comparatively to normal individuals. Type II deficiency occurs less common than type I and could be involved in about 15 % of symptomatic deficiencies [51]. PC deficiency was first described in 1981 by Griffin et al. who detected heterozygosity in a family with a history of recurrent thrombosis. Few years later, in 1984, homozygous PC deficiency was found to be associated with severe neonatal purpura fulminans and disseminated intravascular coagulation within hours of birth [50].
6.2 Prevalence in General Population and VTE
PC deficiency is found in 0.2 % of the general population. It was initially considered as a rare but strong risk factor for thrombosis; however, it was identified in 1:250 blood donors, who were not found to present a higher incidence of thrombosis as well as their relatives carrying the deficiency [50]. Homozygous or compound heterozygous PC deficiency occurs in approximately 1 in 500,000 to 1 in 750,000 live births [52]. There are no apparent racial or ethnic differences regarding the prevalence of PC deficiency in general populations.
The prevalence of PC deficiency in VTE is about 2–5 % [53].
6.3 Clinical Implication
Clinical manifestations in PC deficiency depend upon the underlying mutation and the levels of PC. Many affected individuals may be asymptomatic.
Homozygosity and compound heterozygosity for PC deficiency are manifested either with disseminated intravascular coagulation either with massive thrombosis. Homozygotes with absent levels of PC in plasma present neonatal purpura fulminans shortly after birth. This is a life threatening condition, manifested with extensive thrombosis of capillaries and venules, necrosis of the tissues and massive bleeding into the skin [54]. Homozygotes may also present milder manifestations. The clinical phenotype of heterozygosity for PC deficiency can range from asymptomatic to warfarin-induced skin necrosis and recurrent VTE with consecutive chronic venous insufficiency. The thrombotic risk is usually increased by seven-fold. The first episode of VTE occurs by the age of 45 in half of the cases [51, 55].
In symptomatic families, PC deficiency is associated with an adjusted relative risk of 11.3 for VTE [56]. Family studies showed the importance of the interaction between PC deficiency and other genetic risk factors. The study of each individual’s plasma composition revealed no direct correlation of thrombin generation with PC levels. Recent data shows the cell adhesion molecule 1 as a possible interacting gene, whereas the role of prostaglandin H synthase 1 gene and alpha2 subunit of platelet-activating factor acetyl-hydrolase Ib has not been confirmed [55].
Although it has not been proved an increased risk for arterial thrombosis, several case reports found an association with stroke and myocardial infarction, suggesting that PC deficiency could be tested in young patients, in the absence of the classical cardiovascular risk factors [57].
PC deficiency does not appear to be associated with adverse pregnancy outcome [58].
6.4 Practical Aspects
PC deficiency is detected using immunologic and functional assays. Immunological assays include ELISA (enzyme-linked immunosorbent assay), RIA (radioimmunoassay) and electroimmunoassays [59]. The levels of PC are age-dependent, with lower values in neonates and infants than in adults; the levels may persist lower than in adults until the age of 16. The functional assays use the venom of the southern copperhead snake which activates the PC that will be measured by clot-based or chromogenic methods. Patients with levels less than 50 % are likely to have a deficiency, whereas levels between 55 % and 65 % may be due to heterozygosity or represent the lower limit of the normal values [56].
The functional test is used for the screening of PC deficiency. If PC deficiency is detected, a PC antigen assay should be considered to detect the type I or II of deficiencies [56, 58]. Since there are numerous mutations (almost 200) involved in PC deficiency, no confirmatory DNA test is readily available at the present.
To confirm hereditary PC deficiency, the test should be performed in the absence of an acquired cause of deficiency, such as: oral anticoagulants, vitamin K deficiency, liver diseases. It is recommended to stop oral anticoagulation 10 days before testing [56]. It is commonly believed that acute phase of VTE produces a decrease of PC and PS. However, a recent study found a false positive rate of only 2.2 % of the tests done within 24 h of the diagnosis of VTE, before the administration of oral anticoagulation. This shows that PC testing during the acute VTE event is a valid approach and a normal result is enough to rule out deficiency [60].
Prophylactic therapy for VTE in PC deficiency could be administrated in cases with a positive family history for VTE, in the presence of an acquired risk factor for VTE such as: pregnancy and puerperium, surgery, trauma. Long-term anticoagulation is often recommended in patients with a first episode of VTE, considering the risk for recurrent VTE of about 60 %. Warfarin- induced necrosis is a medical emergency, requiring the immediate discontinuation of warfarin, the administration of vitamin K, heparin and exogenous PC, in the form of fresh frozen plasma or purified concentrate. Neonatal purpura fulminans is a very severe condition, requiring the normalization of PC levels immediately with PC concentrate and then administration of anticoagulant indefinitely [61].
7 PS Deficiency
7.1 Gene Mutations
PS, a vitamin K–dependent glycoprotein, circulates in plasma in two forms: a form bound to protein (60 % of total PS) and a free form (40 %). The free form serves as cofactor in the inactivation of factor Va and VIIIa by PC, enhancing the cleavage of FVa approximately ten-fold [62].
PS deficiency was first described in 1984 by Schwarz who reported the association with VTE in a family. There are three types of PS deficiency: type I (characterized by a decreased amount of both bound and free forms of PS), type II or type IIb (with normal levels of PS, but with an altered function, unable to interact normally with PC) and type III also called IIa (with a low level of free PS, but a normal amount of total PS). Type I and type III deficiencies have been found concomitantly in many families, suggesting the same genetic disease with phenotypic variants [63].
The inherited PS deficiency is an autosomal dominant condition. Molecular studies identified two genes encoding PS, linked closely on chromosome 3p11.1-3q11.2: the active gene, PROS-b (PROS1) and the other, the pseudogene PROS-a, a nonfunctional gene, similar with the active gene, but without the exon 1. Almost 200 mutations of PROS1 gene have been described, such as: the PS Heerlen mutation (due to the substitution of Ser460 by Pro, consecutive to T→C transition in exon 13) resulting in type III deficiency, the PS Tokushima due to K196E substitution, located in the epidermal growth factor-2 domain and causing type II deficiency [62].
7.2 Prevalence in Healthy Populations and VTE
PS deficiency is a rare disorder; a study including 3788 healthy Scottish blood donors showed a prevalence of hereditary PS deficiency of 0.03–0.13 % [62]. The higher prevalence of PS deficiency in Japanese than in Caucasians (1.12 % in men, 1.6 % in women), especially in case of PS Tokushima, may be explained by racial differences or the use of different assay methods [63].
PS deficiency may be found in about 5 % of VTE patients and 1–2 % of patients with a first episode of VTE [63].
However, the incidence of PS deficiency is not well known, since the inter- and intra-individual variation in plasma levels of PS, making the diagnosis of PS deficiency difficult [63].
7.3 Clinical Implications
The main result of PS deficiency is the increase in thrombotic risk. The risk ratio is 8.5 compared to normal individuals and is also higher than in carriers of FV Leiden mutation. Studies of thrombophilic families revealed that individuals with PS deficiency have a five to ten-fold higher risk for VTE than healthy relatives [63]. The annual incidence of recurrent VTE in patients with positive family history is about 6–10 %. However, the risk of VTE associated with PS deficiency in general population is controversial: LETS has shown that only very low levels of free PS are associated with 5.44 higher risk of VTE and not the low levels of total PS. MEGA study found that decreased free PS (and not total PS) leads to a double risk of unprovoked thrombosis. Previous family studies revealed that the cutoff level of free PS to detect PS deficiency should be very low compared to the range that is commonly used [64]. Thus, the free PS levels appear to be a better indicator for the risk of VTE than total PS. Recent data concluded that low free PS and low total PS levels could rarely identify individuals at risk for VTE in population-based studies and should not be considered for testing in unselected cases [64].
It has been shown that about half of PS deficient patients present VTE till the age of 55 and half of VTE events are unprovoked [62]. The use of oral contraceptives by PS deficient women may increase the risk of VTE by 600-fold compared to healthy subjects. Pregnancy or puerperium represents the trigger factor for VTE in women with PS deficiency in about 20 % of cases; the risk of pregnancy loss is triple in PS deficient patients [62]. Possibly linked to these findings, data showed that PS deficient young women present a higher risk of VTE than young men carrying the deficiency [65].
Homozygous or compound heterozygous deficiency is manifested with massive VTE or neonatal purpura fulminans, which could be life-threatening in the absence of medication. Retinopathy may be the initial manifestation in some cases [62].
In spite of several reports about the association between PS deficiency and arterial thrombosis, the results of the large studies are conflicting.
7.4 Practical Aspects
PS deficiency is detected using tests for PS antigen (total antigen or free PS antigen) measured by ELISA methods and functional tests for PS activity, which are indirect and based on prolongation of blood clotting. Since a false PS deficiency could be found in patients with FV Leiden, the dilution of plasma is required for a more accurate determination [66]. Decreased levels of PS are found in inherited deficiency of PS as well as in some acquired conditions, such as liver diseases, vitamin K deficiency, therapy with vitamin K antagonists, pregnancy, HIV infection, varicella, sickle cell disease, malignancy, nephrotic syndrome [67]. The free PS level is not influenced by age, whereas total PS increases with age. Since from practical point of view the distinction between type I and type III of deficiency is not important (no clinical impact on the management of the case), the free protein S antigen testing should be done in any suspected case of PS deficiency.
The total PS antigen in healthy adults is in average 23 mcg/mL, which corresponds to a value of 100 %, or 1 unit/mL, whereas in individuals treated with warfarin is decreased by half [68].
VTE associated with PS deficiency requires anticoagulation according to the protocols. Warfarin could be administrated lifelong in case of life- threatening VTE, located at multiple and unusual sites and in cases with repetitive episodes of VTE. In asymptomatic individuals with PS deficiency, the prophylaxis of VTE should be done in the presence of a major acquired risk factor for thrombosis: (e.g. surgery, pregnancy and postpartum) [69].
Neonatal purpura associated with severe PS deficiency should be promptly treated with vitamin K antagonists. However, despite maintaining the international normalized ratio of the prothrombin time within the therapeutic range for VTE, warfarin-induced skin necrosis may occur. New anticoagulants targeting FII or FX (dabigatran, rivaroxaban) have been proved to be a valid therapy in cases with severe PS deficiency and warfarin- induced skin necrosis [70].
8 Antithrombin Deficiency (or AT III Deficiency)
8.1 Gene Mutations
Antithrombin (AT), a vitamin K-independent glycoprotein, plays a central role in coagulation system, by the inhibition of thrombin and other serine proteases of the coagulation cascade (serpin) including FXa and FIXa. Its inhibitory function is increased by heparin. AT is found in plasma as two main forms: an active monomer and a latent, inactive form. AT undergoes a slow spontaneous conversion to its latent conformation and a more rapid conversion at higher temperature, when a heterodimer of active and latent antithrombin also appears. Heterozygotes with conformationally unstable antithrombins may present severe episodes of thrombosis [71].
AT deficiency is transmitted in an autosomal dominant pattern, in case the patient inherits one copy of the SERPINC1 gene on chromosome 1q25.1, or could be transmitted in an recessive manner, in case two defective genes are inherited, leading to massive thrombosis in neonates [72]. Beside the genetic causes, there are some acquired conditions associated with AT deficiency: liver diseases, nephrotic syndrome, severe trauma, chemotherapy, disseminated intravascular coagulation. There are two main types of AT deficiency: type I, the most common, due to a decreased level of AT and type II, the result of a decreased AT function with possible normal levels of AT antigen. Type II is subdivided into type IIb deficiency, more common, but less thrombogenic, caused by a defect in the heparin-binding region of AT and type IIa deficiency, less common, but more thrombophilic, caused by mutations in the thrombin-binding site. Type IIc deficiency has been also described, as a combination between the previous two subdivisions. Numerous mutations leading to AT deficiency have been reported (e.g. wibble and wobble, mutation of the shutter region of the gene) [73].
8.2 Prevalence in Healthy Populations and VTE
8.3 Clinical Implications
AT deficiency is associated with a high risk of thrombosis, especially VTE, which occurs spontaneously in about 60 % of cases. The thrombotic risk increases after the age of 20 and by the age of 50, about half of the carriers of this condition present a thrombotic episode. The lowest risk of VTE is associated with type IIb of AT deficiency [73]. DVT involves especially the lower limbs, but it may also occur at unusual location. AT deficiency is associated with a three to sevenfold higher risk of VTE compared to other thrombophilias [75]. The risk of recurrent episodes of VTE is about 2.7 %/year, despite anticoagulation [73].
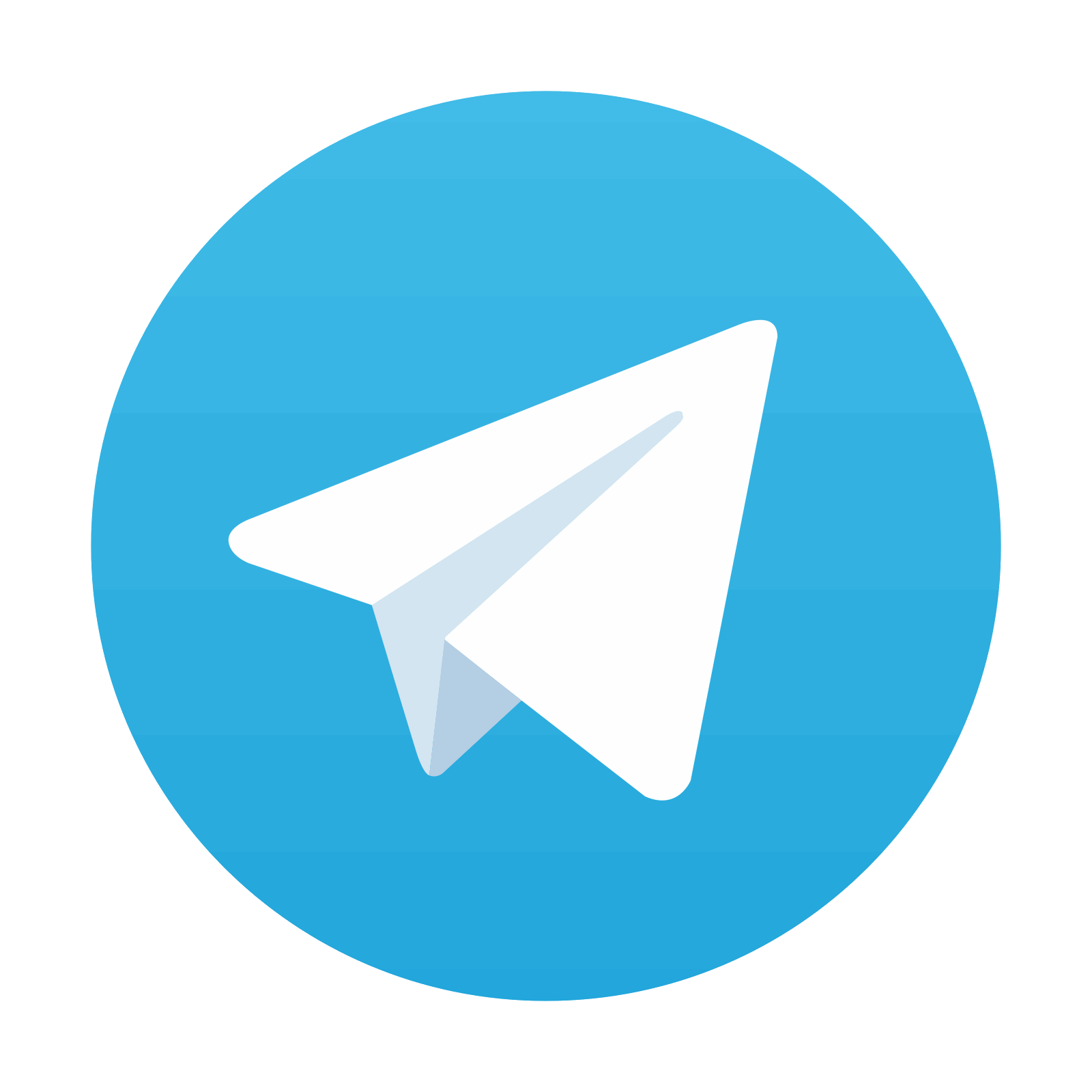
Stay updated, free articles. Join our Telegram channel

Full access? Get Clinical Tree
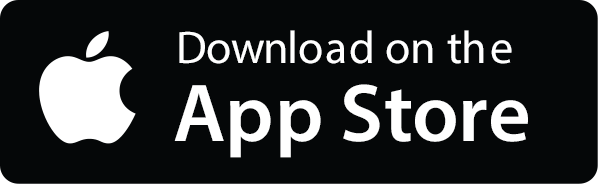
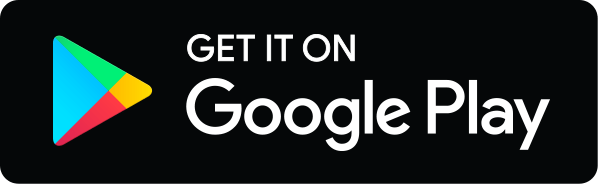