(1)
Institute of Oncology “Prof. Ion Chiricuță”, Nuclear Medicine & Endocrine Tumors, Cluj-Napoca, Romania
“Primum non nocere”
–First do not harm—Hippocrates
6.1 Introduction
The use of specific radiotracers called radiopharmaceuticals for imaging organ function and disease states is a unique capability of nuclear medicine. Unlike other imaging modalities, such as computed tomography (CT), magnetic resonance imaging (MRI), and ultrasonography (US), nuclear medicine procedures are able of mapping physiological or pathological activities and thereby giving more specific information about the organ function and dysfunction. The widespread utilization and growing demands for these techniques are directly attributable to the development and availability of a vast range of specific radiopharmaceuticals.
Radiopharmaceuticals are medicinal formulations containing radioisotopes that are safe for administration in humans, for diagnosis, or for therapy. The diagnosis radiopharmaceuticals usually have no pharmacologic effect because in most cases they are used in trace quantities; they should be sterile and pyrogenic-free. Although radiotracers were tried as a therapeutic medicine immediately after the discovery of radioactivity, the first significant applications came much later with the availability of cyclotrons for acceleration of particles to produce radioisotopes. Subsequently, nuclear reactors realized the ability to prepare larger quantities of radioisotopes. The most commonly used “reactor” to produce isotopes in medical applications is molybdenum-99 generator (for the production of technetium-99m).
The early use of cyclotron in radiopharmaceutical field was for the production of long-lived radioisotopes that can be used to prepare tracers for diagnosis imaging. For this, medium- to high-energy (20–70 MeV) cyclotrons with high beam currents were needed. With the advent of positron emission tomography (PET), there has been a surge in the production of low-energy cyclotrons (9–19 MeV) exclusively for the production of short-lived PET radionuclides such as fluorine-18 (F-18), carbon-11 (C-11), nitrogen-13 (N-13), and oxygen-15 (O-15). The majority of the cyclotrons worldwide are now used for the preparation of fluorine-18 for making radiolabeled glucose for medical imaging.
Currently there are over 100 radiopharmaceuticals developed using either reactor or cyclotron-produced radioisotopes which are used for the diagnosis of several common diseases and the therapy of a few selected diseases, including cancer.
Radiopharmaceuticals production involves handling of large quantities of radioactive substances and chemical processing.
The last decade has seen an increase in the use of PET in regular diagnosis imaging and a commensurate use of PET radiopharmaceuticals, particularly fluorine-18 in the form of fluorodeoxyglucose (18F-FDG). The associated 511 keV high-energy radiations need thicker shielding and more sophisticated handling devices. Regarding the short half-lives, the emphasis is also increasing on the validation process and strict adherence to approved procedures in handling all steps of the manufacture, rather than relying on the final quality control (QC) test results alone.
The specific features of the ideal radiopharmaceutical are summarized in Table 6.1. It must be emphasized, however, that there is no radiopharmaceutical that has all these properties. For the definition of this radiopharmaceutical, there are radionuclide criteria, radiopharmaceutical, radiobiological, and economical criteria.
Table 6.1
The ideal radiopharmaceutical
Radionuclide criteria | Radio pharmacokinetic criteria | Radiobiological criteria | Economic criteria |
---|---|---|---|
Gamma or X-ray emission detectable outside the body | Maximum ratio target organ/surrounding tissues | In diagnostic tests, no corpuscular emission | Low cost |
Optimal photonic energy (50–300 keV) | Optimal effective halftime (T 1/2); it must be eliminated from the body with a T 1/2 similar to the duration of the diagnostic test | Short halftime and effective halftime | Availability at hospital |
Halftime (T 1/2) short, but sufficient till the end of the diagnostic test | The radionuclide should be chemically suitable for incorporating it into the pharmaceutical, without altering its biological behavior | Adequate equipment | |
Absence or as low as possible of alpha, beta particles, Auger electrons | Easy to prepare |
Regarding the radiopharmaceuticals, the presence of radionuclide gives the level of radiotoxicity to the entire product. Radiotoxicity refers to radioactive materials that are toxic to living cells or tissues.
Radiotoxicity results from the:
Type of radiation
The radionuclide half-life
The biological half-life in the tissues
The dose absorbed in the organ
There are four groups of toxicity. The radiotoxicity of most commune radionuclides is presented as follows:
Group 1: Very High Radiotoxicity
Pb-210 Po-210 Ra-223 Ra-226 Ra-228 Ac-227 Th-230 Pa-231 Pu-238 Am-241 Am-243 Cm-242 Cm-243 Cm-244 Cm-245 Cm-246 Cf-249 Cf-250 Cf-252 Ra-226
Group 2: High Radiotoxicity
Na-22 Cl-36 Ca-45 Sc-46 Mn-54 Co-56 Co-60 Sr-89 Sr-90 Y-91 Zr-95 Ru-106 Ag-110m Cd-115m In-114m Sb-124 Sb-125 Te-127m Te-129m I-124 I-125 I-126 I-131 I-133 Cs-134 Cs-137 Ba-140 Ce-144 Eu-152 (13 y) Eu-154 Tb-160 Tm-170 Hf-181 Ta-182 Ir-192 Tl-204 Bi-207 Bi-210 At-211 Pb-212 Ra-224 Ac-228 Pa-230
Group 3: Moderate Radiotoxicity
Be-7 C-14 F-18 Na-24 C1–38 Si-31 P-32 S-35 Ar-41 K-42 K-43 Ca-47 Sc-47 Sc-48 V-48 Cr-51 Mn-52 Mn-56 Fe-52 Fe-55 Fe-59 Co-57 Co-58 Ni-63 Ni-65 Cu-64 Zn-65 Zn-69m Ga-72 As-73 As-74 As-76 As-77 Se-75 Br-82 Kr-85m Kr-87 Rb-86 Sr-85 Sr-91 Y-90 Y-92 Y-93 Zr-97 Nb-93m Nb-95 Mo-99 Tc-96 Tc-97m Tc-97 Tc-99 Ru-97 Ru-103 Ru-105 Rh-105 Pd-103 Pd-109 Ag-105 Ag-111 Cd-109 Cd-115 In-115m Sn-113 Sn 125 Sb-122 Te-125m Te-127 Te-129 Te-31m Te-132 I-130 I-132 I-134 I-135 Xe-135 Cs-131 Cs-136 Ba-31 La-140 Ce-141 Ce-143 Pr-142 Pr 143 Nd-147 Nd-149 Pm-147 Pm-149 Sm-151 Sm-153 Eu-152 Eu-155 Gd-153 Gd-159 Dy-165 Dy-166 Ho-166 Er-169 Er-171 Tm-171 Yb-175 Lu-177 W-181 W-185 W-187 Re-183 Re-186 Re-188 Os-185 Os-191 Os-193 Ir-190 Ir-194 Pt-l91 Pt-193 Pt-197 Au-196 Au-198 Au-l99 Hg-197 Hg-197m Hg-203 Tl-200 Tl-201 Tl-202 Pb-203 Bi-206 Bi-212 Rn-220 Rn-222
Group 4: Low Radiotoxicity
H-3 O-15 Ar-37 Co-58m Ni-59 Zn-69 Ge-71 Kr-85 Sr-85m Rb-87 Y-9lm Zr-93 Nb-97 Tc-96m Tc-99m Rh-103m In-113m I-129 Xe-131m Xe-133 Cs-134m Cs-135 Sm-147 Re-187 Os-191m Pt-193m Pt-197m
Considering this fact, in nuclear endocrinology, there are not used radiopharmaceuticals with very high radiotoxicity; the iodine isotopes I-125 and I-131 belong to the group of high radiotoxicity (group 2), and this is important to keep in mind, in order to limit the unjustifiable indication of diagnostic tests. The most frequent used in diagnosis is Tc-99m, which is included in the group 4 of radiation toxicity, of low risk.
6.2 Radiopharmaceuticals in Endocrinology
In nuclear endocrinology, radiopharmaceuticals may be classified according to their purpose: diagnosis or therapy. Even if the compounds are multiple, the radionuclides which are linked to this substances are relatively limited, many of them laying on some physiological features and proprieties of the endocrine system (Table 6.2).
Table 6.2
The most common radiopharmaceuticals used in nuclear endocrinology
Radiopharmaceutical | Usage |
---|---|
F-18 fluorodeoxyglucose (18F-FDG) | Tracer for PET (thyroid, endocrine tumors) |
F-18 choline (18-FCH) | Tracer for PET parathyroid |
16α-(18F)Fluoro-17β-Estradiol (18F-FES) | Tracer for PET tumors expressing estrogen receptors |
Gallium-67 citrate | Infection and inflammation |
Gallium-68 DOTA (Ga-68 DOTA) | Neuroendocrine tumors |
Iod-123 as sodium iodide (I-123 NaI) | Thyroid, adrenal glands |
Iod-124 as sodium iodide (I-124 NaI) | Tracer for PET (thyroid, endocrine tumors) |
Iod-125 as sodium iodide (I-125 NaI) | Thyroid |
Iod-131 as sodium iodide (I-131 NaI) | Thyroid, adrenal glands |
Iod-123 as metaiodobenzylguanidine (I-123 MIBG) | Thyroid, adrenal glands, neuroendocrine tumors |
Iod-131 as metaiodobenzylguanidine (I-131 MIBG) | |
Indium-111 pentetreotide (In-111 pentetreotide) | Endocrine tumors |
Technetium-99m pertechnetate (Tc-99m Pt) | Thyroid |
Technetium-99m sestamibi (Tc-99m MIBI) | Parathyroid |
Technetium-99m tetrofosmin (Tc-99m tetrofosmin) | Parathyroid |
Technetium-99m Tekrotyde | Neuroendocrine tumors |
Technetium-99m dimercaptosuccinic acid (Tc-99m DMSA) | Thyroid |
Thallium-201 chloride (Tl-201 chloride) | Thyroid |
Other peptide receptor radiotracers (PRR)—(lutetium-177, yttrium-90, etc.) | Endocrine tumors |
6.2.1 Fluorine-18 Fluorodeoxyglucose (18F-FDG)
Fluorine-18 is a positron-emitting radioisotope. The application of 18F-FDG was aimed for mapping glucose metabolism in tumors considered to be highly glucose consuming. The major use of 18F-FDG subsequently emerged in the detection, staging, and treatment response monitoring of various types of cancers. Currently, in PET studies, the use of 18F-FDG accounts for the majority of all images performed with radiopharmaceuticals. A number of other fluorine-18-labeled radiopharmaceuticals have been developed, and many more are under clinical investigations.
Increasing clinical demand for 18F-FDG has triggered technological advances in various fields such as accelerator technology, radiochemistry, automated processing modules, detector systems, and imaging software.
Dispensers and injectors perform the most critical steps in the radiopharmaceutical handling processes. In Fig. 6.1 the equipment for loading, dispensing, and calibrating the dose of F18-FDG ready for injection for PET/CT imaging is presented.
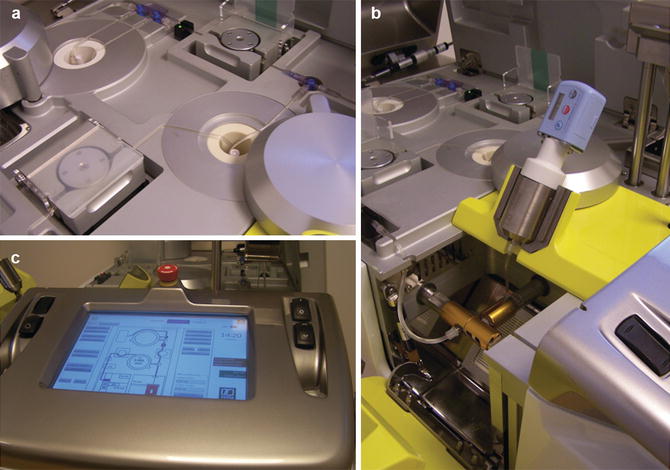
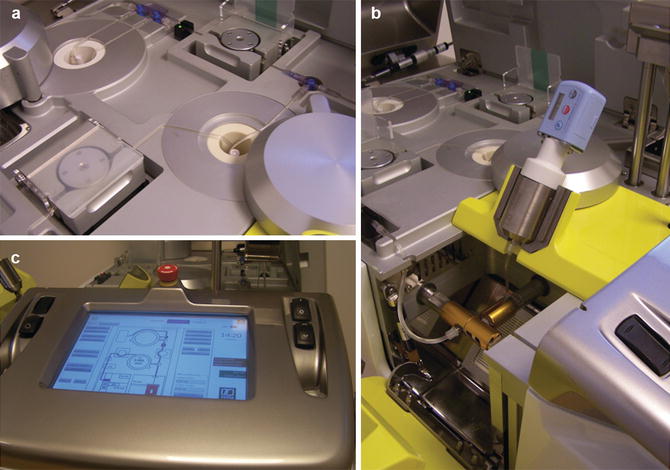
Fig. 6.1
The 18F-FDG calibrator used for loading and calibrating 18F-FDG for PET or PET/CT imaging, model Karl 100 (Tema Sinergie): dispersing (a), loading in the syringe (b), and calibrating (c)
The use of 18F-FDG in endocrinology was not particularly interesting, until the experience brought the necessary knowledges, especially in endocrine cancers. Nevertheless PET studies are still indicated in well-defined clinical situations, due to some less invasive and more accessible methods that might be used in the frequent pathologies, such as those of the thyroid gland; regarding the diagnosis of the endocrine tumors, the role of PET/CT is clear and will be discussed in the following chapters of the book.
6.2.2 Fluorine-18 Fluorocholine (18F-FCH)
F18-Fluorocholine PET/CT appears to be a promising, effective imaging method for localization of hyperfunctioning parathyroid tissue. The performance of 18F-FCH PET/CT was superior to Tc-99m MIBI standard methods, particularly in patients with multiple lesions or hyperplasia. The tracer was first used in prostate cancer, where the mechanism consists in the following mechanism: FCH enters cell through choline transporters with accumulation in tumors in part due to malignancy-induced overexpression of choline kinase (CK) that catalyzes the phosphorylation of choline to form phosphorylcholine followed by generation of phosphatidylcholine in the tumor cell membrane.
The lack of availability and the costs are limiting seriously the use of this tracer in parathyroid pathology, being necessary larger studies to conclude on the superiority of the method, over the standard MIBI imaging.
6.2.3 Radiopharmaceuticals with Gallium-68 and Gallium-67
Gallium-67 is produced by bombardment of Zn-68 in a cyclotron. Ga-67 decays by electron capture to Zn-67, with a half-life of about 78 h. There are three gamma emissions at 93, 185, and 300 keV. Following intravenous injection, gallium distributes widely in the body. It binds to circulating proteins, fact that leads to long persistency in the plasma. Gallium has a wide normal distribution throughout the soft tissues, mainly in the liver, spleen, bone, bone marrow, and bowel. There is a rapid uptake in the zones with high rate of inflammation, due mainly to the increased capillary permeability occurring in these processes.
Somatostatin receptors are expressed by many neuroendocrine and non-neuroendocrine cells of the body, so different organs may be imaged by somatostatin receptor scintigraphy, including the liver, spleen, pituitary gland, thyroid, kidneys, adrenal glands, salivary glands, stomach wall, and bowel.
Ga-67 citrate was considered the master radiopharmaceutical for tumor imaging and detection of inflammatory sites. Its role is highly affected after the introduction of PET tracers.
Gallium-68 (halftime is 68 min) is available from the Ge-68/Ga-68 generator. In terms of radiochemistry, labeling with metallic nuclides is based on chelating systems which are coupled to biomolecules or which have interesting biological properties themselves. A new tracer is Ga-68 DOTA (Ga-68-labeled 1,4,7,10-tetraazacyclododecane-N, N′,N″,N‴-tetraacetic acid). Ga-68 DOTA-conjugated peptides (DOTA-TOC, DOTA-NOC, DOTA-TATE) are rapidly cleared from the blood. Arterial activity elimination is bi-exponential, and no radioactive metabolites are detected within 4 h in serum and urine. Excretion is almost entirely through the kidneys.
Just recently the Ge-68/Ga-68 generators and the DOTA-conjugated peptide obtained a marketing authorization, until now, and they have to be prepared taking into account national regulations and good radiopharmaceutical practices (GRPP) as outlined in specific European Association of Nuclear Medicine (EANM) guidelines. PET with Ga-68-DOTA-conjugated peptides has brought dramatic improvement in spatial resolution and is being used more and more often in specialized centers. All of them can bind to some of the somatostatin receptors, and they also present different affinity profile for other somatostatin receptor subtypes. In particular, PET clearly offers higher resolution and improved pharmacokinetics as compared to somatostatin receptor scintigraphy, with promising results for the detection of somatostatin receptor expressing tumors, and provides prognostic information and selection for targeted specific treatments.
6.2.4 Radiopharmaceuticals with Radioiodine
6.2.4.1 Radioiodine I-123 Sodium Iodide (I-123 NaI)
The isotope iodine-123 (I-123) with a half-life of 13.22 h is used as a tracer, in order to evaluate the anatomic and physiologic function of the thyroid. It decays by electron capture to tellurium-123 and emits gamma radiation with predominant energies of 159 and 127 keV. This is the most suitable isotope of the iodine for the diagnosis study of thyroid diseases. The half-life of approximately 13.2 h is ideal for the 24-h iodine uptake test and also diagnostic scanning imaging of the thyroid tissue.
The energy of the photons, 159 keV, is ideal for the NaI (sodium iodide) crystal detector of current gamma cameras. It has a much higher photon flux than I-131. It gives approximately 20 times the counting rate of I-131 for the same administered dose. The radiation burden to the thyroid is far less (1%) comparing to I-131 mainly because of the absence of beta radiation compound. This is the reason why this isotope is reserved only for diagnosis, not being suitable for treatment.
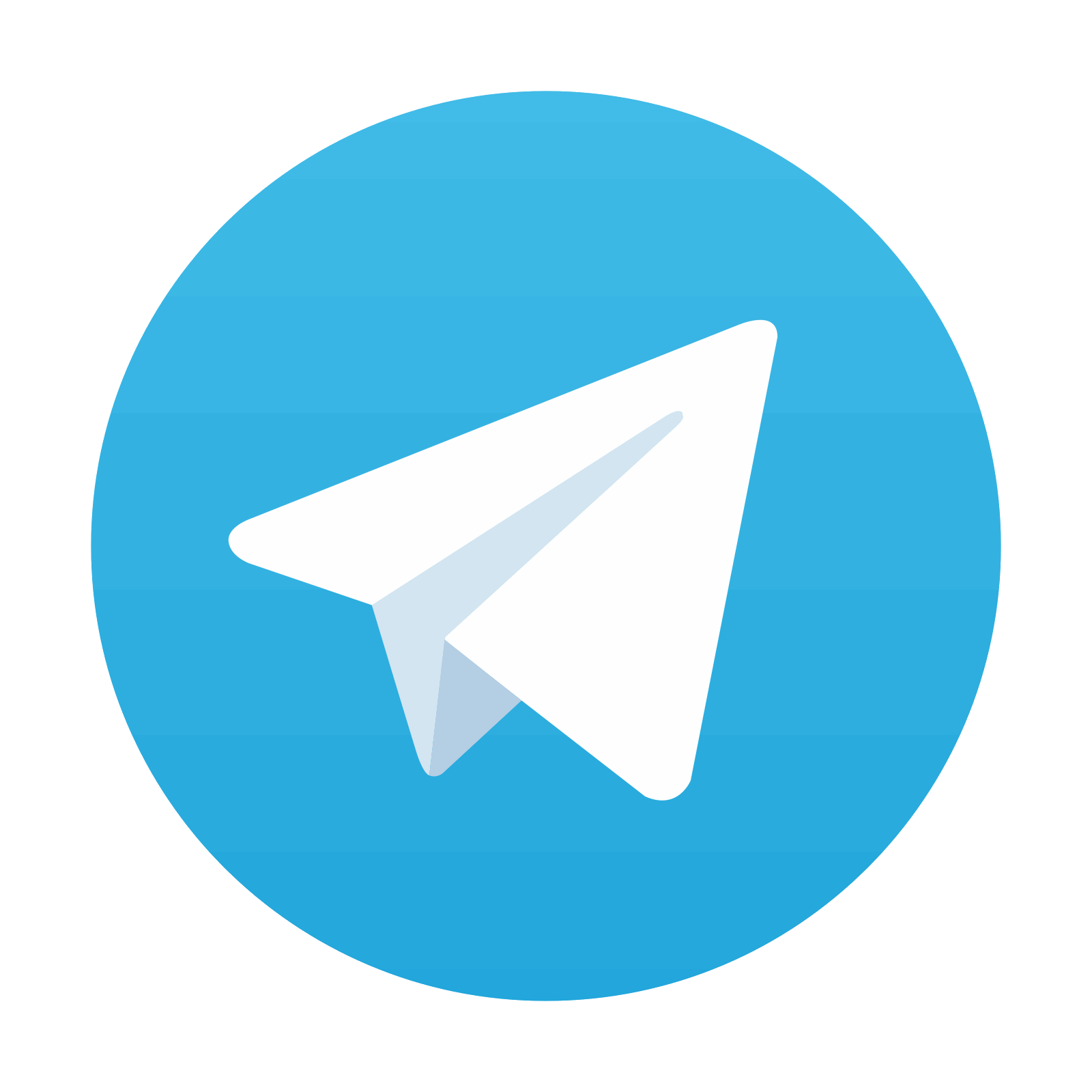
Stay updated, free articles. Join our Telegram channel

Full access? Get Clinical Tree
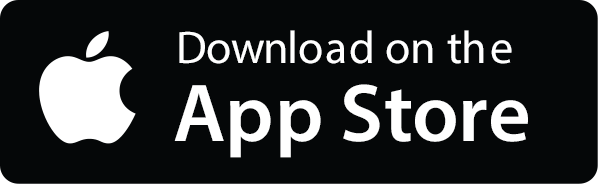
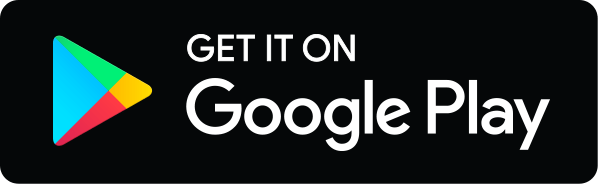
