Frequent clinical features
Secretion
Management (first line)
Stomach
Mainly endoscopic discoverya
Rare
Depends on type (see text)
Small intestine
Often discovered on complication (obstruction, perforation, bleeding)
Liver metastasis
Carcinoid syndrome
30 %, mainly serotonin
Surgery
Somatostatin analogues
Cardiac monitoring
Appendix
Mainly incident discovery during surgery
No
Right hemicolectomy should be discussed for tumor > 1 cm
Colon
Mainly endoscopic discoverya
Rare
Endoscopic resection if possible
Surgery
Rectum
Mainly endoscopic discoverya
Rare
Endoscopic resection if possible
Surgery
15.4 Role of Imaging in GI NET Diagnosis and Management
Considering surgery as the only curative treatment in patients without metastases or with limited metastatic spread [11], it is essential to define the real extent of the disease and to identify patients with inoperable disease [12, 13]. Accordingly, medical imaging plays an increasingly crucial role by (a) guiding tumor preoperative assessment (i.e., identification of the primary, assessment of locoregional extension and distant metastases), (b) expanding surgical approaches, and (c) evaluating the therapeutic outcome. In patients with a known or suspected GI NET, a multidisciplinary strategy including both radiological studies and nuclear medicine examinations is usually employed. The former provides detailed anatomical information that is indispensable for primary tumor detection, locoregional and distant metastases identification, surgical planning optimization, and medical treatment evaluation. The latter allows a noninvasive characterization of tumoral functional status and variability at the molecular and cellular level starting from the analysis of uptake intensity and kinetics of some target-specific radiotracers. Molecular imaging techniques are also very sensitive and can detect disease at an early stage. Nuclear medicine provides further details regarding the tumoral extent improving patient staging and therapeutic strategy. In order to improve the diagnostic accuracy, anatomic and functional imaging are usually combined by using « hybrid » modalities as positron emission tomography/computed tomography (PET/CT) and single-photon emission computed tomography/computed tomography (SPECT/CT) devices. Nowadays, PET/MRI hybrid systems are also available and may be effective, although the role of PET/MR in GI NETs needs to be established and its availability in clinical routine is limited.
15.5 Diagnostic Performances of Anatomic Imaging
In the evaluation of patients with suspected or known GI NETs, a number of techniques have been proposed to identify the tumor primary site, to assess locoregional extent, and to assess the presence of distant metastases. A variable combination of endoscopy, ultrasound (US), endoscopic ultrasound (EUS), CT, and magnetic resonance imaging (MRI) is typically adopted to provide optimal patient management, taking into account the clinical context and both strengths and limitations of each diagnostic modality (Table 15.2).
Table 15.2
Comparison of the main anatomical imaging and endoscopic techniques currently available
TAUS | EUS | Video capsule | CT | MRI |
---|---|---|---|---|
Widely available | Highly sensitive technique for the detection of gastric, duodenal, and rectal primary NETs | Attractive technique for the detection of esophageal, gastric, duodenal, and small-bowel primary NETs | Widely available | Multiplanar contrast-enhanced images |
Use limited to solid organs only | Diagnostic biopsy is possible | Analysis of the entire bowel is possible | Multiplanar contrast-enhanced images | First-choice imaging modality for detection and assessment of liver metastases (superior to CT) |
High inter-operator variability | Invasive diagnostic procedure | Moderate sensitivity | First-choice imaging modality for staging and follow-up | High sensitivity for bone marrow metastases |
Limited sensitivity for primary GI NET detection | Identification of tumor primary site, evaluation of local extent, and assessment of metastases | Enterography and enteroclysis are possible and can enhance sensitivity | ||
Enterography and enteroclysis are possible and can enhance sensitivity | Gadolinium chelate more safe than CT iodine-contrast agents as regards allergic reactions and nephrotoxicity | |||
Ionizing-radiation technique | No ionizing radiation |
15.5.1 Primary Tumor Detection
Upper and lower endoscopy enable the detection of esophageal, gastric, colorectal, and some terminal ileal NETs. Double-balloon or push enteroscopy is more efficient for small intestine lesions but remains a time-consuming technique and uncomfortable for the patient [14]. Capsule endoscopy seems an attractive technique for esophagus, stomach, and small intestine examination but does not permit lesion biopsy [15]. The main advantage of video capsule is that it is a noninvasive technique able to analyze the entire small bowel. However, it has only moderate sensitivity (46–65 %) for tumor detection [16]. The use of transabdominal ultrasonography (TAUS) in GI NET diagnosis and staging is mainly limited to the solid viscera and suffers from inter-operator variability. Overall, US has limited sensitivity (13–27 %) for the detection of gastrointestinal NETs [17]. Intraoperative US could be also performed but it prolongs operating time, and it is not free of potential risk of iatrogenic injury during the diagnostic procedure. Conversely, endoscopic US (EUS) has a remarkable role. EUS is a highly sensitive technique for the detection of gastric, duodenal, and rectal NETs permitting not only the identification of small submucosal tumors but even to perform diagnostic procedures such as biopsy or fine-needle aspiration [18]. However, EUS remains a more invasive imaging method. In patients suspected of having small-bowel tumors, the enteroclysis and barium-contrast examinations may show fixation, separation, thickening, and angulation of the bowel loops, but they are rarely diagnostic. Small primary tumors are difficult to visualize unless there is secondary fibrosis. At present, barium-contrast studies have been largely replaced by multi-planar contrast-enhanced CT or MR imaging followed by small-bowel distention before a focused CT or MR enterography or enteroclysis to improve tumor detection. Small-bowel tumors are multifocal in about 30 % of the cases [19], and they manifest as polypoid lesions or hypervascular parietal concentric or asymmetrical thickening. The sensitivity and specificity for tumor detection of CT and MR enterography or enteroclysis have been estimated at 100 % and 86–94 %, and 96 % and 95–98 %, respectively. Overall sensitivity increases with rising tumor size [20–24]. Artifacts from bowel motion and lower spatial resolution compared with CT may be limiting factors of MR enterography or enteroclysis.
15.5.2 Tumor Staging and Metastatic Assessment
CT remains the first-choice diagnostic procedure for tumor staging allowing the assessment of local extent to the mesentery as well as metastases to lymph nodes, liver, and lungs. Typically, the appearance of mesenteric invasion by carcinoid tumor on CT is a spiculated mass with heterogeneous contours, variably associated to central calcifications and usually near the primary tumor (“cartwheel” pattern) [25]. Metastatic lymph nodes are frequently in the mesentery or retroperitoneal and paracardial gastric lymph nodes. For the assessment of metastatic hepatic disease, all available imaging modalities frequently miss lesions sized less than 5 mm [26]. Nevertheless, multiphasic imaging is recommended, with the hepatic arterial phase being the most informative for lesion detection [27]. On multiphasic contrast-enhanced CT or MR images, hepatic lesions are usually hypervascular in the arterial phase and demonstrate washout in the late phase [28, 29]. MRI is a very sensitive technique for the detection of liver metastases, and it is considered more accurate than both US and CT [26]. Multiphasic MRI with fat-suppressed contrast-enhanced T1-weighted imaging provides the best accuracy [29, 30]. Typically, NET lesions show T2 hyperintensity and T1 hypointensity. The use of diffusion-weighted imaging (DWI) and apparent diffusion coefficients (ADC) could improve the detection ability of MRI for malignant liver lesions [31]. An added advantage of using DWI is its ability to reflect lesion changes in response to treatment [32]. Finally, the advantages of MRI over CT are the lack of ionizing radiation and the use of gadolinium chelate contrast agents, which have a better safety profile in terms of allergic reactions and nephrotoxicity than iodine-contrast agents. Metastases to the bone arise more commonly from foregut primary tumors. The preferred site of metastatic spread is the axial skeleton and radiographic signs may be easily missed. On standard radiography and CT, bone metastases frequently demonstrate either an osteosclerotic or a mixed osteolytic–osteosclerotic pattern. MRI is the most sensitive technique for detection of bone marrow metastases [33–35].
15.6 Radionuclide Imaging for Molecular and Metabolic Characterization
Nuclear medicine imaging for NETs exploits some molecular characteristics that are specific for the neuroendocrine phenotype. Hence, the overexpression of specific membrane receptors as well as the ability to take up and decarboxylate amine precursors has been considered for diagnostic radiotracer development. Moreover, the glycolytic metabolism, which is not a specific energetic pathway of NETs, has been also proposed (Table 15.3). Accordingly, a personalized nuclear medicine exploration will be tailored for each patient taking into consideration both clinical symptomatology and the tumor characteristics.
Table 15.3
Comparison of the main functional imaging techniques currently available
111In-pentetreotide SPECT/CT | 68Ga-somatostatin analogues PET/CT | 18F-FDOPA PET/CT | 18F-FDG PET/CT |
---|---|---|---|
Primary staging Restaging Evaluation of uptake before peptide receptor radiotherapy (PRRT) | Primary staging Restaging Unknown primary Evaluation of uptake before PRRT | Primary staging Unknown primary (Restaging?) Carcinoids +++ | Prognostic stratification High-grade G2 and G3 NETs |
Approved nuclear medicine technique for NET imaging | It will replace SRS in the near future | Sensitivity may be lower than 68Ga-PET/CT for non-ileal NETs | Widely available |
Low spatial resolution (>10 mm) | High spatial resolution (5 mm) | High spatial resolution (5 mm) | High spatial resolution (5 mm) |
2-day procedure | 1-day procedure | 1-day procedure | 1-day procedure |
Radiation exposure | Radiation exposure | Radiation exposure | Radiation exposure |
15.6.1 Radiolabeled Somatostatin Analogue Imaging
The somatostatin receptor (SSTR) is a seven-transmembrane G-coupled receptor, of which six human subtypes have been described and which upon activation reduces endocrine secretion and cell growth and increases apoptosis [36]. Upon binding of an agonist to the receptor, the receptor/ligand complex will internalize in the cell, and the receptor will be later recycled to the cell surface. A vast majority (>85 %) of intestinal NETs will have moderate to high overexpression of one of the six SSTR subtypes (1, 2A, 2B, 3, 4, and 5) [36], most frequently subtype 2A [37]. The SSTR has been used as a molecular target in nuclear medicine since more than 25 years [38]. Radioligands binding to the SSTR detected by gamma camera scintigraphy and SPECT were the first to be developed and are based on synthetic somatostatin analogues (SSAs) such as the 8 amino acid derivative octreotide or further optimized chemical variants of this vector backbone. Different chelators have been developed to allow labeling of these vector molecules with a whole range of radioisotopes. One gamma camera radiopharmaceutical is commercially available (111In-pentetreotide; Octreoscan™), and technetium-99 m-labeled tracers have been described as well, using both octreotide and octreotate backbones with a HYNIC chelator/linker. The use of somatostatin receptor scintigraphy (SRS) is recommended in the guidelines at diagnosis [1] and during follow-up [39]. It offers high sensitivity and specificity in detecting tumoral lesions in grades 1 and 2 NET, outperforming other nuclear medicine tracers such as 18F-fluorodeoxyglucose (18F-FDG) and 123I-meta-iodobenzylguanidine (123I-MIBG). In a study by Binderup and colleagues, SRS detected more lesions in all organs studied than 18F-FDG or 123I-MIBG with a sensitivity of 90 % vs. 57 % and 53 %, respectively [40]. Of note, in highly proliferative NET (Ki-67 > 15 %), 18F-FDG had a sensitivity of 92 % vs. 69 % for SRS [40]. Besides tumor detection, SRS allows to determine functional target presence for treatment with cold SSAs. Two randomized controlled trials (RCTs) have shown a prolongation of progression-free survival (PFS) in NET patients treated with SSAs; one trial included well-differentiated metastatic midgut NETs (PROMID) [41], and the other included well-differentiated nonfunctional tumors of the pancreas, midgut, hindgut, or unknown origin (CLARINET) [42]. A positive SRS was an inclusion criterion in the CLARINET study, whereas in the PROMID trial, 73 out of 85 patients had a scintigraphy performed, with 86 % positive and 14 % negative patients [41], highlighting the importance of the scintigraphy as a selection tool for this treatment. For peptide receptor radionuclide therapy (PRRT), good uptake on pre-therapy SRS has always been considered a prerequisite. For example, in the Netter-1 RCT, a positive scintigraphy on all evaluable lesions was an inclusion criterion [43].
Uptake on whole-body planar 111In-pentetreotide scintigraphy is typically scored according to the Krenning scale, with grade 1 representing tumor uptake lower than the liver, grade 2 tumor uptake equal to the liver, grade 3 uptake higher than liver, and grade 4 tumor uptake as the most intense site in the body (usually meaning higher than spleen uptake). A grade 3 uptake is typically required for PRRT candidates.
In the last decade, a novel class of radiopharmaceuticals for SSTR imaging has emerged as the current gold standard (Fig. 15.1). These tracers are based on octreotide derivatives labeled with the positron-emitting radionuclide gallium-68. Gallium-68 is a radioisotope of the metal gallium. It decays primarily through positron emission (abundance 89 %). It has a half-life (T1/2) of 68 min and can be obtained from a germanium-68/gallium-68 generator (T1/2: 271 days), thus allowing in-house production of labeled radiopharmaceuticals. Gallium can be chelated by a number of different chelators to label peptides or other biomolecules for detection with PET, PET/CT, or PET/MR. Labeling has to be done at the site of administration as the yield of the generator, and the half-life usually does not allow for off-site transport.
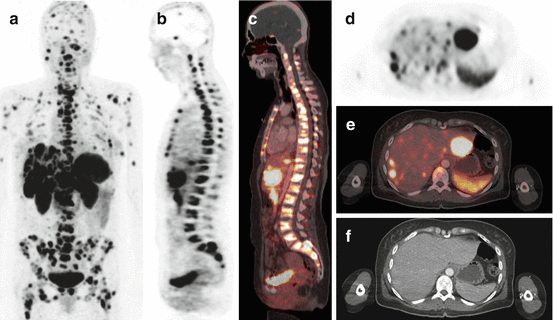
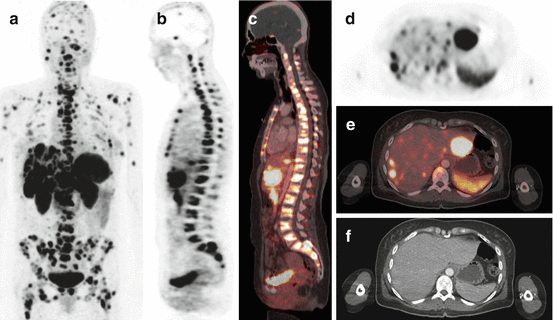
Fig. 15.1
Example of a 48-year-old NET patient with an 11-year disease history with high SSTR expression (SUVmax = 36 in the most intense lesion) and high multifocal tumor burden in the bone, pancreas, soft tissue, and liver on 68Ga-DOTATATE PET/CT. The patient was sent for PRRT eligibility and was considered as candidate. (a) MIP image. (b) Sagittal PET showing diffuse bone involvement and liver metastases. (c) Corresponding PET/CT fusion. (d) Axial view showing multifocal liver metastases with several lesions exceeding the uptake level of the spleen; the lesion in the left liver lobe is the one with the highest uptake of all lesions (SUVmax = 36). Note metastases in both humeri. (e) Corresponding PET/CT fusion, (f) corresponding CT with very little findings, both in the liver (late venous contrast phase) and in the bone
There are several gallium-68-labeled tracers for SSTR imaging that have been described and are in clinical use in Europe of which 68Ga-DOTATOC, 68Ga-DOTATATE, and 68Ga-DOTANOC are the most commonly used. “DOTA” refers to the chelator, which is covalently bound to a slightly modified synthetic octapeptide with high affinity for the SSTR (all have high affinity for SSTR2, the most overexpressed subtype). They are collectively referred to as 68Ga-DOTA-peptides or 68Ga-OctreoPET.
These 68Ga-DOTA-peptides have advantages over gamma scintigraphy-based imaging. They have a much higher affinity for SSTR2 compared to 111In-pentetreotide (IC50 = 2.5 nM for 68Ga-DOTATOC, 0.2 nM for 68Ga-DOTATATE, and 1.9 nM for 68Ga-DOTANOC, vs. 22 nM for 111In-pentetreotide) [44]. This better affinity profile combined with the physical advantages of current clinical PET cameras over gamma cameras, with higher spatial resolution and higher sensitivity (detected events per unit of radioactivity), allows detection of smaller lesions and detection of lesions with even low or moderate SSTR expression, resulting in a higher sensitivity (Fig. 15.2). There is strong scientific data that the 68Ga-DOTA-peptides are significantly better diagnostic agents for performing SSR imaging than 111In-pentetreotide. Gabriel et al. [45] compared 68Ga-DOTATOC with 111In-pentetreotide in 84 NET patients and found a sensitivity of 97 % for 68Ga-DOTATOC vs. 52 % for 111In-pentetreotide. 68Ga-DOTATOC showed better performance for small lesions in lymph nodes and for bone metastases (Fig. 15.3). Buchmann et al. [46] compared 68Ga-DOTATOC with 111In-pentetreotide in 27 NET patients and found a sensitivity of 100 % and 66 %, respectively. They concluded that the PET ligand was superior for detection of lung and bone metastases. Van Binnebeek et al. [47] have recently shown similar results with sensitivity of 99.9 % for 68Ga-DOTATOC with only 60 % for 111In-pentetreotide in metastatic NET patients scheduled for PRRT. Furthermore, they showed that in up to 80 % of the patients, the PET ligand would detect at least 20 % more lesions, showing that the benefit of 68Ga-DOTA-peptide PET reaches a large fraction of patients. Recent data from Northern America have shown a lesion sensitivity of 95.1 % vs. 45.3 % for anatomic imaging and 30.9 % for 111In-pentetreotide SPECT/CT in 131 GEP-NETs or NETs from unknown primary [48]. In four out of 14 patients (29 %), 68Ga-DOTATATE PET/CT found a previously unknown primary tumor. The therapy recommendation was changed on the basis of the 68Ga-DOTATATE PET/CT in one third of the patients [48]. Again, large differences between 68Ga-DOTATOC and 111In-pentetreotide were reported by Morgat and colleagues in the follow-up of MEN1 patients [49].
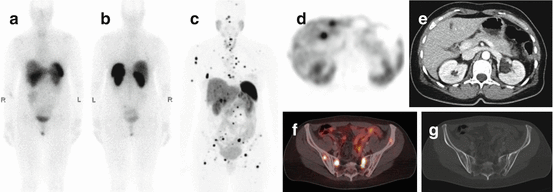
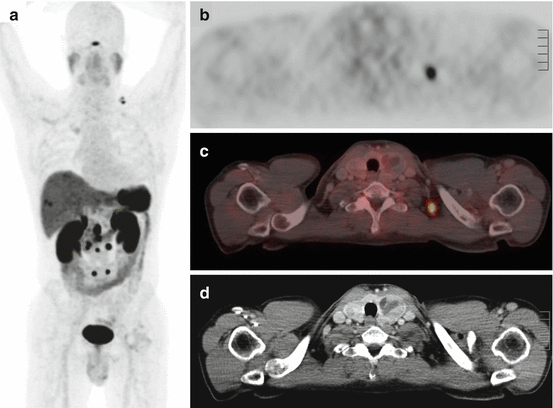
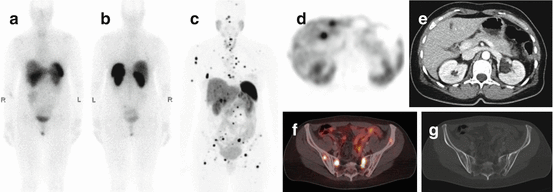
Fig. 15.2
Example of a 50-year-old female NET patient scanned with both 111In-pentetreotide and 68Ga-DOTATATE PET/CT. Anterior (a) and posterior (b) planar projections of 111In-pentetreotide at the time of initial diagnosis. The anterior image does not show any sites of increased uptake. The posterior image shows a small focus with slightly increased uptake in the left hemisacrum. (c) MIP image of 68Ga-DOTATATE PET/CT performed because of suspicious findings on liver MRI and rising serum chromogranin 12 months later. There are multiple small liver and bone metastases with strong to intense tracer uptake. (d) Transverse image showing two liver metastases with strong uptake. (e) Corresponding late venous phase contrast-enhanced CT with the absence of radiological correlate (the hypodense structure in the mid-liver is the falciform ligament). (f) Fusion image showing four pelvic bone metastases with intermediate to strong uptake; one lesion in the left hemisacrum corresponds to the location seen on the posterior 111In-pentetreotide image. (g) CT in bone window showing only subtle changes at the site of the bone metastases seen on the fusion image
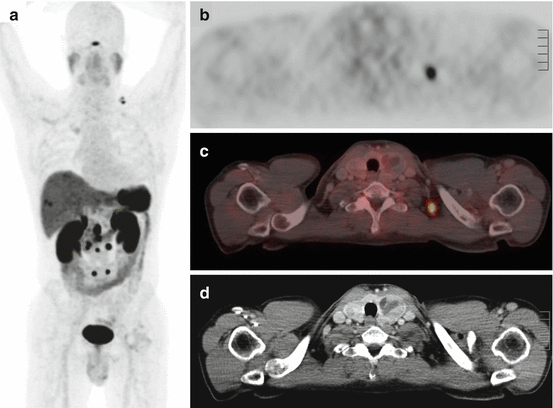
Fig. 15.3
Example of a 54-year-old male patient with a small-bowel NET with known abdominal lymph node metastases and liver metastases under cold SSA therapy. Patient was referred in a potential presurgical setting. (a) MIP image of a 68Ga-DOTATATE PET/CT showing multiple mesenterial and retroperitoneal lymph node metastases with high uptake as well as two right-sided subcapsular liver metastases and a metastasis in the liver dome. There is also a bifocal lesion in the left supraclavicular area. (b) Focal strongly increased uptake in the largest of the two left-sided supraclavicular lesions, with an SUVmax of 8.5 (the smallest lesion – not shown – had an SUVmax of 4.5). (c) Fusion PET/CT image showing the localization of the hot spot. (d) CT-graphic correlate shows a non-enlarged lymph node of 7-mm diameter (the smaller lesion – not shown – has a diameter of 4 mm). In light of the extent of tumor involvement, no surgery was performed. This case illustrates the ability of 68Ga-DOTA-peptide PET/CT to detect metastatic involvement in CT-graphic normal lymph nodes
Although there are some differences in the affinity profiles (68Ga-DOTATOC: SSTR2 > SSTR5; 68Ga-DOTATATE: SSTR2; 68Ga-DOTANOC SSTR2 > SSTR5 > SSTR3), clinical comparative studies have only shown minor if there are any differences in lesion detection rate and lesion uptake when comparing head to head two of these PET SSTR ligands (Fig. 15.4). The consensus is that for the vast majority of clinical indications, these tracers are equivalent [50]. In a head-to-head comparison in the same patients (n = 40) between 68Ga-DOTATOC and 68Ga-DOTATATE, 262 and 254 (97 %) lesions were detected with an average standardized uptake value (SUV) of 20.4 and 16.0, respectively [51].
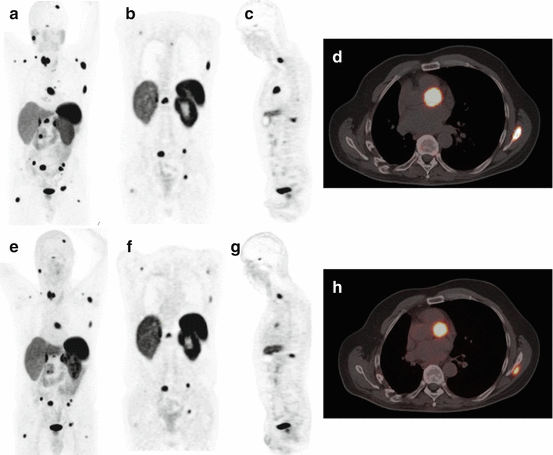
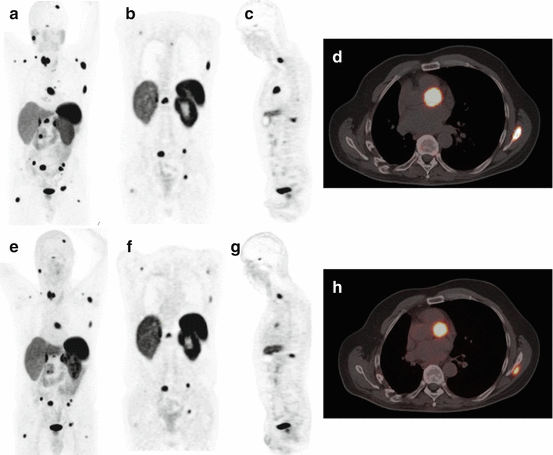
Fig. 15.4
Example of a 47-year-old male patient with a NET scanned with 68Ga-DOTATATE PET/CT 14 months after a 68Ga-DOTATOC PET/CT with very comparable images. (a–d) 68Ga-DOTATATE PET/CT images. (e–h) 68Ga-DOTATOC PET/CT images. (a, e) MIP images showing diffuse metastases with high to intense tracer uptake, with a similar pattern. (b, f) Coronal images showing three pelvic bone metastases with similar uptake and signal to background ratio; the thoracic lesions are different because of different alignment of the transection plane. (c, g) Sagittal images showing two vertebral metastases with similar uptake. (d, h) PET/CT fusion image showing similar aspect of a cardiac metastasis, which are not infrequent in NET patients and can be readily detected by 68Ga-DOTA-peptide PET/CT
The clinical impact of 68Ga-DOTA-peptide PET is mainly due to:
- 1.
Detection of smaller lesions
- 2.
Detection of lesions with low to moderate SSR expression (Fig. 15.5)
Fig. 15.5
Example of a 58-year-old patient with a NET with absent SSTR expression and diffuse liver metastases on 68Ga-DOTATOC PET/CT. The patient was sent for PRRT eligibility and was not retained as a candidate, based on manifest lack of target expression. (a) MIP image, showing a primary lung NET with uptake lower than normal liver parenchyma and a photopenic area in the center of the liver dome, (b) coronal section showing the primary lung NET with rounded photopenic areas within the liver corresponding to liver metastases without SSTR expression, (c, d) sagittal and axial views through the liver showing photopenic metastases, (e) axial view of the liver with corresponding CT and fusion image, (f) axial views of the liver with corresponding CT and fusion image, with the orange crosshair centered on a photopenic liver metastasis, nicely visible on CT and on the fusion image
- 3.
Detection of more lesions that potentially will direct the patient to a different therapeutic choice
- 4.
- 5.
Lower radiation burden to the patient
- 6.
Detection of an occult primary tumor, with yields as high as 30–60 % in patients negative on conventional imaging [54]
When comparing a 68Ga-DOTA-peptide PET image with a previous scan of the same patients, one needs to be careful that visualizing more lesions does not necessarily mean that the patient is progressive.
The physiological biodistribution in normal patients is as follows: high uptake in spleen (hottest organ in normal patient), kidneys, adrenal glands, liver, pituitary gland, and the bladder (tracer excretion). There is also moderate uptake within the pancreas and more notably the uncinate process, the thyroid gland, the intestine. There is low uptake in the lungs, muscles (including myocardium), and the bone marrow.
Typical pitfalls in SSTR PET include misinterpretation of the physiological uptake in the uncinate process of the pancreas as a pancreatic NET, misinterpretation of a small meningioma (which can also have high SSTR expression) as a bone metastasis or brain metastasis, misinterpretation of accessory spleens, intrapancreatic spleen, or splenosis in operated or trauma patients as metastases of a NET, and classifying the mild to moderate uptake seen in inflammatory processes due to activated white blood cells as metastatic disease, in postsurgical scar tissue, or inflammatory or reactive lymph nodes, or in facet joint osteoarthritis, for example.
Several developments are currently ongoing to optimize SSTR PET imaging. A relatively recent paradigm shift is coming from radiolabeled antagonists that allow achieving higher uptake than with all previously mentioned ligands, which are agonists [55, 56]. Because some antagonist analogues are independent of the conformational status of SSTR, they can bind to a much higher fraction of the receptors present than agonists, which only bind certain specific conformations. This could lead to the emergence of second-generation 68Ga-DOTA-peptides with even higher uptake ratios and potentially higher clinical detection rate. Another evolution is optimization of the labeling process. One major drawback of gallium-68-labeled peptides is the necessity to perform an on-site radiolabeling step, which requires specialized radiopharmaceutical personnel that is lacking in most nonacademic PET centers. Some groups are developing SSTR ligands labeled with radioisotopes that would allow centralized production and distribution to peripheral sites, using, e.g., copper-64 (T1/2: 12.7 h) [57] or aluminum-fluoride-18 (T1/2: 110 min) complexes that can be chelated and bound to octreotide derivatives [58]. The latter isotope is produced in curie quantities in cyclotrons throughout the world to produce fluorine-18 for 18F-FDG synthesis and would allow scanning 10–30 patients per production, compared to 1–4 for gallium-68-labeled peptides. For gallium-68, kits are currently being developed that would allow a more facile on-site labeling. It will be interesting to see which of these improvements will be the more adopted in clinical practice.
15.6.2 18F-Fluorodihydroxyphenylalanine PET
The cellular ability to take up, accumulate, and decarboxylate amines and amine precursors has been taken into account for the development of amino acid-based diagnostic radiotracers for nuclear medicine imaging. Accordingly, dihydroxyphenylalanine radiolabeled with fluorine-18 (18F-FDOPA) has been successfully proposed for in vivo nuclear imaging of NETs [59]. Once internalized via the sodium independent system L, 18F-FDOPA is decarboxylated to 18F-dopamine by the aromatic L-amino acid decarboxylase (AADC) enzyme, transported and stored into secretory vesicles. Therefore, the high uptake of 18F-FDOPA in NETs is the result of the cellular increased synthesis, storage, and secretion of biogenic amines [60, 61].
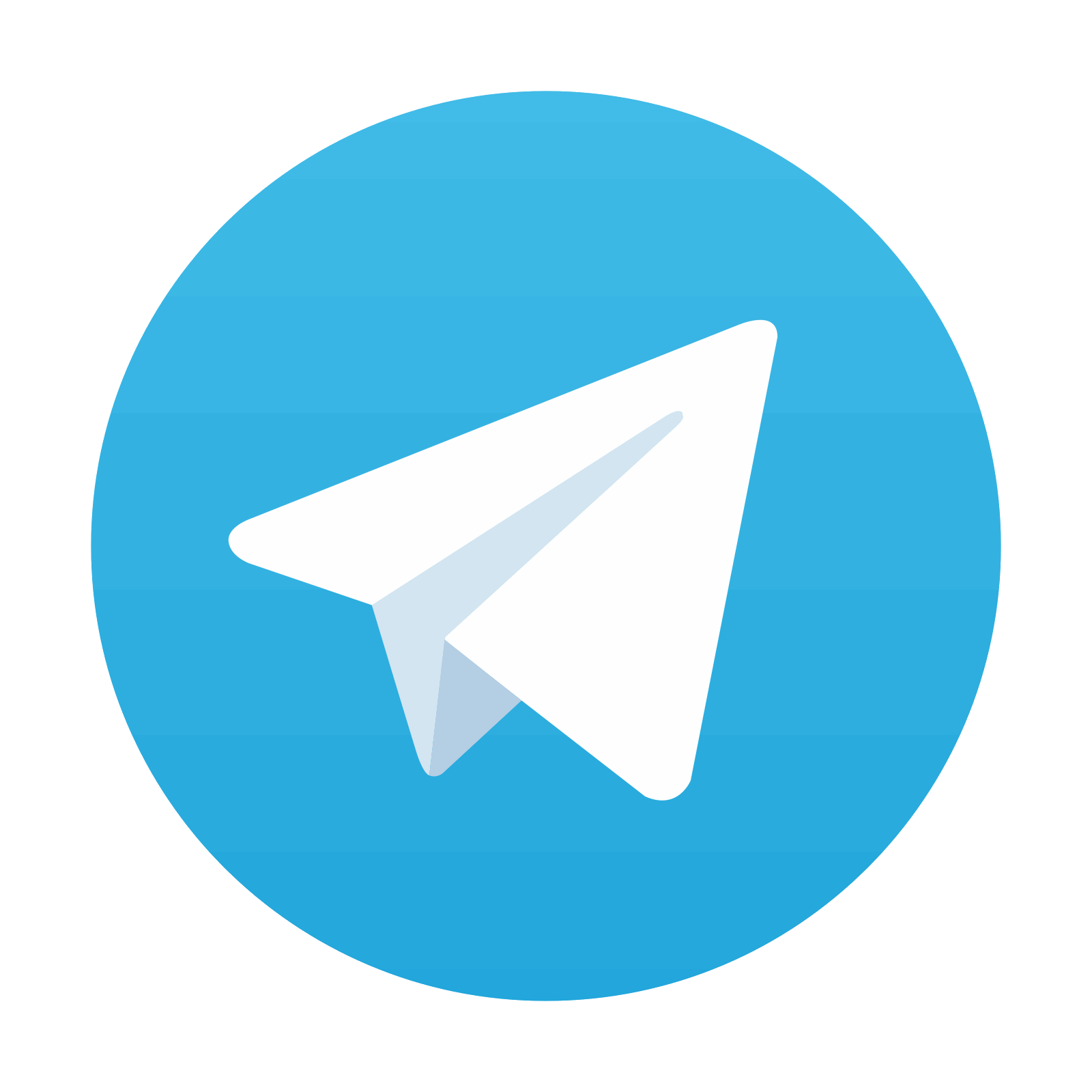
Stay updated, free articles. Join our Telegram channel

Full access? Get Clinical Tree
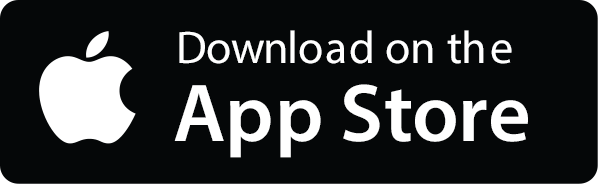
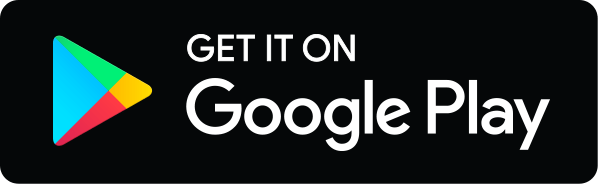