Fig. 20.1
Distribution of neuroendocrine tumors
Except for the undisputed role of surgery in the management of neuroendocrine tumors, the development and registration of the somatostatin analogs (congeners) octreotide and lanreotide have been critical in the management of NETs, both in symptom control and in delaying disease progression. Equally relevant, these analogs have helped unmask the unique, highly sensitive, and specific properties of most NETs, namely, somatostatin-octreotide/lanreotide cell membrane receptor (somatostatin receptor subtype 2, sstr2) kinetic [8]. As well, we now know that both octreotide and lanreotide have antiproliferative (therapeutic) action [9, 10]. Octreotide has a “mild antiproliferative” action shown with a placebo-controlled, double-blind randomized noncrossover trial [9]. The CLARINET Trial was a randomized placebo versus lanreotide-controlled double-blind study (cross = over design) that demonstrated clear antiproliferative action of lanreotide [10]. Lanreotide has been recently registered in the USA with specific indication as an antiproliferative drug for NETs.
In order to better understand the rationale for somatostatin analogs’ use in both NETs diagnosis and therapy, it is necessary to briefly review the properties of the somatostatin analogs. The development of a synthetic analog for somatostatin (originally termed “somatotropin release-inhibiting factor, SRIF”) began shortly after its isolation in 1973 [11]. The first analog such compound to become clinically available was octreotide (SMS 201–995) followed by lanreotide. The striking similarities between the two synthetic analogs can be appreciated in Fig. 20.2. As can be noted, both synthetic somatostatin analogs have achieved reproducing the biologically active portion of native somatostatin in the ringed amino acid residues (eight amino acids) without needing to synthetize the whole 1–14 residue native compound. Equally important is the maintenance of the lysine residue within the synthetic ring. Lysine is the purported binding unit to the somatostatin membrane receptor, sstr2 [12]. Another observation can be made from an exam of Fig. 20.2 is that the synthetic somatostatin analog tested and available for both diagnosis and therapy is a slightly modified octreotide. [Tyr(3)]-octreotide (TOC) represents the replacement of phenylalanine residue in position 3 with the tyrosine moiety. When the chelator, DOTA, is attached to TOC (DOTATOC), it can be labeled with the radiometal gallium-68 (68Ga) for diagnosis and detection of sstr2 receptor avid NETs and Yttrium-90 (90Y) or lutitium-177 (177Lu) for therapy. As well, octreotate (TATE) is tyrosine(3)-octreotide but with an amidated form of threonine rather than threoninol. It is linked to the chelator, DOTA, and is labeled with 68Ga for diagnosis and 90Y or 177Lu for therapy. Lanreotide has also been linked to a chelator and used for therapy with 177Lu/90Y, but the modified octreotide forms are now fully developed, and 68Ga-DOTATE has been approved by the United States Food and Drug Administration for PET imaging of somatostatin receptor-positive tumors.
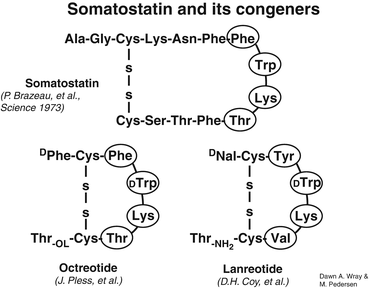
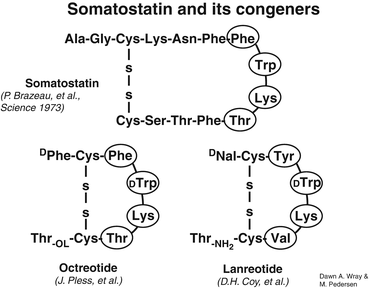
Fig. 20.2
Peptide structure of somatostatin and its congeners
Two important works from JC Reubi helped to establish the predominate somatostatin subtype receptor 2 to be present on at least 80 % of all neuroendocrine tumors [13]. He also demonstrated the high affinity and specificity of the labeled synthetic analogs for the somatostatin receptor subtype 2. Table 20.1 has been modified from Reubi’s work which compares the affinity profiles (IC50) of a series of synthetic somatostatin analogs to the subtypes of human somatostatin receptors, sstr2-5 [14]. The sstr1 responses have been deleted for clarity since all forms had IC50s greater than 10,000. Further, only the mean value for each analog for the respective receptor is shown, and the SDs were deleted for clarity. Cell lines were transfected with each somatostatin receptors. As can be noted, when compared to the IC50 of octreotide, the affinities of the synthetic analogs DOTATOC and DOTATATE were variously improved when radiolabeled with 68Ga or 90Y.
Table 20.1
Affinity profiles (IC50) of a series of somatostatin analogs to the subtypes of human somatostatin receptors (sstr2–5) modified from JC Reubi et al. [14]
Peptide | sstr2 | sstr3 | sstr4 | sstr5 |
---|---|---|---|---|
SS-28 | 2.7 | 7.7 | 5.6 | 4.0 |
Octreotide | 2.0 | 187 | >1000 | 22 |
DTPA-Octreotide | 12 | 376 | >1000 | 299 |
DOTATOC | 14 | 27 | >1000 | 103 |
68Ga-DOTATOC | 2.5 | 613 | >1000 | 73 |
90Y-DOTATOC | 11 | 389 | >10,000 | 114 |
DOTATATE | 1.5 | >1000 | 453 | 547 |
68Ga-DOTATATE | 0.2 | >1000 | 300 | 377 |
90Y-DOTATATE | 1.6 | >1000 | 523 | 187 |
20.2 Peptide Receptor Radionuclide Therapy (PRRT): Rationale and Patient Selection
PRRT for NETs uses radiolabeled octreotide analogs to deliver targeted radiation to somatostatin receptor (primarily sstr2)-positive tumor tissue. Success of PRRT is based upon delivering high radiation dose to tumors while minimizing harmful radiation effects to nontarget tissues. Achievement of a high therapeutic index requires selection of patients with disease with high expression of somatostatin receptor subtype 2 (sstr2) which binds the octreotide analog component of the radiolabeled PRRT compound [15]. Imaging with 111In-DTPA-octreotide (Octreoscan®) or the 68Ga-labeled octreotide analogs can gauge the degree of sstr2 expression in tumors and identify patients appropriate for PRRT [15]. Quantification of sstr2 expression by histology alone is limited to the site of the sample and may misgauge the sstr2 receptor expression at the remainder of disease sites [15].
PRRT agents are composed of a radionuclide bound to a somatostatin receptor analog by a chelator. Diethylenetriamine pentaacetic acid (DTPA) has been used as the chelator to label octreotide with 111In; however, the current PRRT agents labeled with 90Y and 177Lu utilize 1,4,7,10-tetra-azacyclododecane-N,N’,N”,N”’-tetra-acetic acid (DOTA) because of its improved stability of the radionuclide-peptide complex [16]. The stability of labeling is critical as free 177Lu and 90Y accumulate in the skeleton increasing radiation dose to the bone marrow [17]. Initial attempts in PRRT were performed with 111In-DTPA-octreotide, which emits—in addition to gamma rays used for imaging—Auger and conversion electrons that have cytotoxic effects. These particles have tissue penetration of 0.02–10 μm and 200–500 μm, respectively [18]. Objective responses were rare with 111In- DTPA-octreotide [19], which led to efforts in developing beta-emitter PRRT agents. Beta particles have higher energy and longer range tissue penetration, which increases cytotoxicity. 90Y has tissue penetration of 11 mm, while 177Lu has a shorter maximal tissue penetration of 2 mm. Although different somatostatin analogs have been developed for 90Y and 177Lu DOTA-chelated peptides, 90Y-DOTATOC and 177Lu-DOTATATE have emerged as the primary radiopharmaceuticals for PRRT for NETs.
Consensus guidelines for indications, preparation, administration, and follow-up for PRRT in NETs have been published [20]. PRRT is indicated for treatment of metastatic or inoperable NETs with positive expression of sstr2 [20]. After histologic confirmation of NET, candidacy for PRRT requires demonstration of the presence of sstr2 receptors on target tumors with somatostatin receptor imaging. As primary toxicities associated with PRRT are renal and bone marrow toxicity, poor renal function and inadequate bone marrow reserve are relative contraindications to PRRT. Given the more severe renal toxicity associated with 90Y-DOTATOC, normal renal function is generally required for 90Y-DOTATOC, whereas patients with mild/moderate renal impairment may be safely treated with 177Lu [20]. The following hematological parameters are suggested prior to PRRT: white blood cell count >3000/μl, absolute neutrophil count >1000/μl, RBC >3,000,000/μl, platelets >75,000/μl for (177Lu), and >90,000/μl for (90Y) [20]. Patient with diffuse bone/bone marrow metastases should not be treated with 90Y-DOTATOC but may be treated with 177Lu-DOTATATE [21].
An amino acid co-infusion for renal radioprotection is now a standard practice for PRRT. The mechanism of action of amino acid infusion will be discussed later in the chapter. The recommended amino acids are lysine and/or arginine diluted in large volumes of saline, typically 25 g of amino acid in 1 L of normal saline with the large saline volume used for protection against electrolyte disturbances. A minimum of 50 g of arginine and/or lysine (typically given as 25 g of lysine and 25 g of arginine) should be administered, and the amino acid infusion needs to be started 30–60 min prior to the administration of the radiopharmaceutical. Currently there is no commercially available preparation of an amino acid solution in the USA that contains only arginine and lysine; the commercially available amino acid solutions contain a multitude of amino acids with a significantly higher osmolarity, which is associated with a higher incidence of nausea and vomiting. The amino acid infusion takes approximately 4–6 h depending on the infusion rate. As the amino acid infusion is frequently accompanied by nausea and vomiting, antiemetic therapy (such as a 5-HT3 antagonist) should be given [20].
20.3 Efficacy of Peptide Receptor Radionuclide Therapy (PRRT)
20.3.1 90Y-DOTATOC
[90Y-DOTA0,Tyr3]-octreotide (90Y-DOTATOC) was the first beta-emitter PRRT agent. 90Y is a pure β-emitter, with a maximum energy of 2.3 MeV and maximum range of 11 mm and a half-life of 64 h. The relatively long range of the β-particle of 90Y allows for a bystander effect in heterogeneous tumors in which some cells may not express somatostatin receptors. 90Y-DOTATOC is generally administered in fixed doses of 2.78–4.44 GBq every 6–12 weeks over 2–4 cycles [20]. 90Y-DOTATOC has been investigated in several phase 1 and 2 studies; there are currently no randomized trials comparing 90Y-DOTATOC with accepted standard therapy agents for NETs.
The largest study with 90Y-DOTATOC in NETs was reported by Imhof et al. in 1109 patients with tumor progression in the preceding last 12 months prior to PRRT [22]. There were 821 patients with gastroenteropancreatic NETs, 185 patients had unknown primaries, and 103 with other less common NETs. Patients were treated with 3.7 GBq/m2 per cycle, with additional treatment cycles administered if they showed stable or improving disease at follow-up; additional treatment was withheld if they showed progression or permanent toxicity. Morphologic response (any change in tumor size) was observed in 34 % of patients, although complete response was only seen in 0.6 %. Drop in tumor markers was noted in 15 %, and clinical response (improvement in symptoms and/or weight gain) was observed in 60 % of patients. Overall median survival after 90Y-DOTATOC PRRT was 94.6 months, which was 2.9 times longer than historic data for metastatic NETs treated with other modalities [22]. The efficacy of 90Y-DOTATOC in advanced NETs was also reported in two multicenter studies [23, 24]. The first study was a phase 1 study that included 58 patients, 47 of them with progressive disease, who were treated with a wide range of cumulative doses between 1.7 and 32.8 GBq of 90Y-DOTATOC. There were no complete responses in this group; partial or minimal response was seen in 21 %, and stable disease was reported in 45 % of the patients who initially presented with progression. Symptomatic response was observed in 57 % of patients [24]. In another multicenter phase 2 study, Bushnell et al. reported the results of 90 patients with symptomatic carcinoid tumor treated with 4.4 GBq of 90Y-DOTATOC, every 6 weeks over 3 cycles. Although only 4 % showed a partial response (no complete response) and 70 % had stable disease after therapy, 51–60 % of patients reported durable improvement in carcinoid symptoms including diarrhea, flushing, and abdominal pain, with an average length of symptomatic response between 8 and 12 weeks [23]. These studies along with other single-center studies show that 90Y-DOTATOC therapy may provide objective disease control by stabilizing patients with progressive disease, however, rarely leads to complete or partial responses. 90Y-DOTATOC therapy provides durable symptomatic relief in majority of patients and may also improve overall survival. The response to PRRT is generally worse in patients with higher tumor burden in the liver, more advanced disease, and higher grade NETs [25]. In children and young adults, a phase 1 study in 17 patients with a variety of somatostatin receptor-positive tumors including neuroblastoma, NETs, and brain tumors, PRRT with 90Y-DOTATOC was safe without dose-limiting toxicities and an overall response rate of 41 % [26].
20.3.2 177Lu-DOTATATE
177Lu-[DOTA0,Tyr3] octreotate (177Lu-DOTATATE) is both a β-emitter and γ-emitter. The 177Lu β particles have maximum energy of 0.5 MeV and maximum range of 2 mm and a half-life of 6.7 days. The lower β particle energy as compared with 90Y improves irradiation of smaller-size tumors [16]. 177Lu also emits γ-rays of energy 208 and 113 keV with relative abundance of 10 % and 6 %, respectively, which allows for imaging and dosimetry in addition to therapy [16]. Similar to 90Y-DOTATOC, standard protocols for 177Lu-DOTATATE therapy follow a fractionated fixed dosing regimen consisting of 3–5 cycles with a per cycle dose of 5.55–7.4 GBq [20]. Per cycle activity is reduced if dosimetry indicates radiation dose to the kidneys exceeding 23 Gy [27]. In addition to several phase 1 and 2 non-randomized/non-controlled trials, early data is now available for 177Lu-DOTATATE from the first prospective randomized/controlled phase 3 trial (NETTER-1), the first of its kind for a PRRT agent. The experience of several of the largest studies and the preliminary data from the NETTER-1 clinical trial are summarized.
The largest prospective study to date reported phase 2 efficacy results for 177Lu-DOTATATE in 310 patients with various types of GEP-NETs (carcinoid n = 188, nonfunctional pancreatic n = 72, unknown origin n = 31, gastrinoma n = 12, insulinoma n = 5, VIPoma n = 2), 43 % of them with disease progression prior to therapy. Overall objective tumor response rate was 46 %, including complete response (CR) in 2 %, partial response (PR) in 28 %, and minor response (MR) in 16 %. Median overall survival (OS) from the start of treatment was 46 months [27]. The pancreatic NET subgroup was the focus of two recent studies. In a prospective phase 2 trial of 52 patients with pancreatic NETs, where 26 patients received 27.8 GBq and 26 received 18.5 GBq, the high-dose cohort showed a complete response of 12 %, partial response of 27 %, and stable disease of 46 %, compared to 4 %, 15 %, and 58 %, respectively, for the low-dose group [28]. Another retrospective analysis of 68 patients with pancreatic NETs showed comparable results with overall disease control rate of 85 % in patients with baseline progression [29]. 177Lu-DOTATE was also effective in small-bowel NETs with an overall objective disease control rate of 91.8 % (PR and SD) [30]. Another study in 265 patients also demonstrated the symptomatic improvement after PRRT, with improvement in pain, nausea/vomiting, and diarrhea, observed in 53–70 % of patients regardless of the objective disease outcome [31]. In two pilot studies of pediatric patients, treatment with 177Lu-DOTATE has shown objective responses in children with refractory neuroblastoma [32, 33].
The NETTER-1 study is the first phase 3 multicenter stratified randomized controlled trial of PRRT with parallel group design comparing 177Lu-DOTATATE with octreotide LAR (Sandostatin® LAR Depot). The study evaluated 230 patients with inoperable progressive metastatic somatostatin receptor-positive grade 1–2 midgut NETs. Patients were randomized to either PRRT plus supportive octreotide (177Lu-DOTATATE 7.4 GBq every 8 weeks for four total administrations with 30 mg Sandostatin® LAR every 4 weeks) versus octreotide alone (Sandostatin® LAR 60 mg every 4 weeks). At the time of this writing, results (abstract only) showed statistically significant favorable results for the intent-to-treat (ITT) arm including longer progression-free survival (median not reached for ITT versus 8.4 months for control) and larger objective radiographic complete and partial response rate (18 % versus 3 %). Overall survival data could not be definitively evaluated at the time of this writing, but early analysis suggests a possible improvement in survival for ITT group. The safety profile for 177Lu-DOTATATE was favorable with dose-modifying toxicity occurring in only 5 % and grade 3 or 4 hematologic toxicity occurring in 9 % versus none of the controls [34, 35].
In summary, similar to 90Y-DOTATOC, 177Lu-DOTATATE is highly effective in achieving disease control, albeit with few partial/complete responses, and provides symptomatic relief. It improves progression-free survival compared to the standard therapy with octreotide. There are no randomized trials comparing 90Y-DOTATOC with 177Lu-DOTATATE in patients with neuroendocrine tumors. The reader is directed to a tabular summary of study results with both radiopharmaceuticals in the excellent review paper by Bodei et al. 2016 [25].
20.4 Toxicity from PRRT
PRRT labeled with 90Y and 177Lu has been proven to be generally safe and well tolerated. The most common adverse effect during administration of PRRT is acute mild-moderate nausea (25–80 %) and less often vomiting (10 %) relating to coadministration of amino acids [27, 36]. Hormonal crisis occurs in about 1 % of patients receiving PRRT [37]. Although the mechanism of hormonal crisis is not well understood, manipulation of the tumor as with PRRT, chemotherapy, induction of anesthesia, surgery, or endoscopy can result in a sudden large release of metabolically active compounds [37]. Symptoms of hormonal excess include severe flushing, hypotension, blood pressure fluctuation, bronchoconstriction, and arrhythmia [37, 38]. These patients may benefit from subcutaneous or IV octreotide administration.
Long-term moderate and severe adverse effects are uncommon. Renal and hematologic toxicity represent the two major long-term potential adverse effects associated with PRRT. For many radiotherapies, marrow is the dose-limiting organ; however, for 90Y- and 177Lu-labeled PRRT agents, the kidneys are the critical dose-limiting organs as these agents are primarily cleared through the kidneys [39–41]. These radiolabeled small peptides are first filtered through the glomeruli, and because of their relatively small size (<70 kDa) are reabsorbed through the proximal tubules, a process mediated by the membrane protein megalin [40, 42]. Renal parenchymal retention of radiolabeled peptides increases radiation dose to the kidneys and can transiently and permanently degrade renal function and even lead to end-stage renal disease [43, 44]. Renal biopsies in patients with chronic renal failure after 90Y PRRT therapy showed thrombotic microangiopathy as a cause of renal dysfunction [45].
Rates of renal failure after PRRT are low, especially when kidney dosimetry and amino acid administration are followed. In a retrospective series of 807 patients receiving various combinations of 90Y-octreotide or 177Lu-octreotate, nephrotoxicity of any grade and duration occurred in 34.6 %, although severe nephropathy (grade 3 or 4) was only noted in 1.5 % of patients, occurring only in the patients receiving 90Y-DOTATOC [46]. In a prospective series of 1109 patients receiving 90Y-DOTATOC, the nephrotoxicity was higher with 9.2 % of developed grade 4 or 5 permanent renal toxicity; the relatively higher administered activity of 90Y-DOTATOC in this study per cycle, at 3.7GBq/m2 per cycle, may have contributed to the relatively higher incidence of toxicity in this group [22]. In a series of 504 patients receiving 177Lu-DOTATATE, no renal toxicity attributable to the therapy was observed [27]. Overall, 177Lu-labeled PRRT shows lower rates of nephrotoxicity than 90Y-labeled PRRT, which is likely due to the lower energy β particle emission of 177Lu, which results in lower tissue penetration [16].
The maximum permissible cumulative absorbed dose to the kidney appears to be ~23–27 Gy [47, 48]. Coadministration of highly concentrated amino acids with PRRT has long been recognized as a method of reducing renal radiation dose and toxicity by competitively blocking the renal tubular reabsorption and retention of the radiolabeled peptides, resulting in a renal absorbed dose reduction of 9–53 % [39, 47, 49, 50]. The positively charged amino acids D-lysine and L-arginine have been particularly effective [47, 49, 51]. Amino acid administration is responsible for the most frequently encountered acute side effects of PRRT. Rates of gastrointestinal toxicity following amino acid infusion have ranged from 25 to 69 % depending on the infusion protocol, with higher infusion rates associated with more nausea/vomiting [27, 47]. These symptoms have been attributed to the level of osmolarity and, in particular, to the arginine component of the solution [47]. The nausea and vomiting can be successfully controlled with antiemetics [25, 27]. Another potential renoprotective agent is Gelofusine, which is a plasma tissue expander that has been shown to disrupt protein reabsorption in the kidneys, reducing renal radiation dose by 45 %, comparable to amino acid infusion [52]. A summary of studies reporting on severe renal toxicity is presented in Table 20.2.
Table 20.2
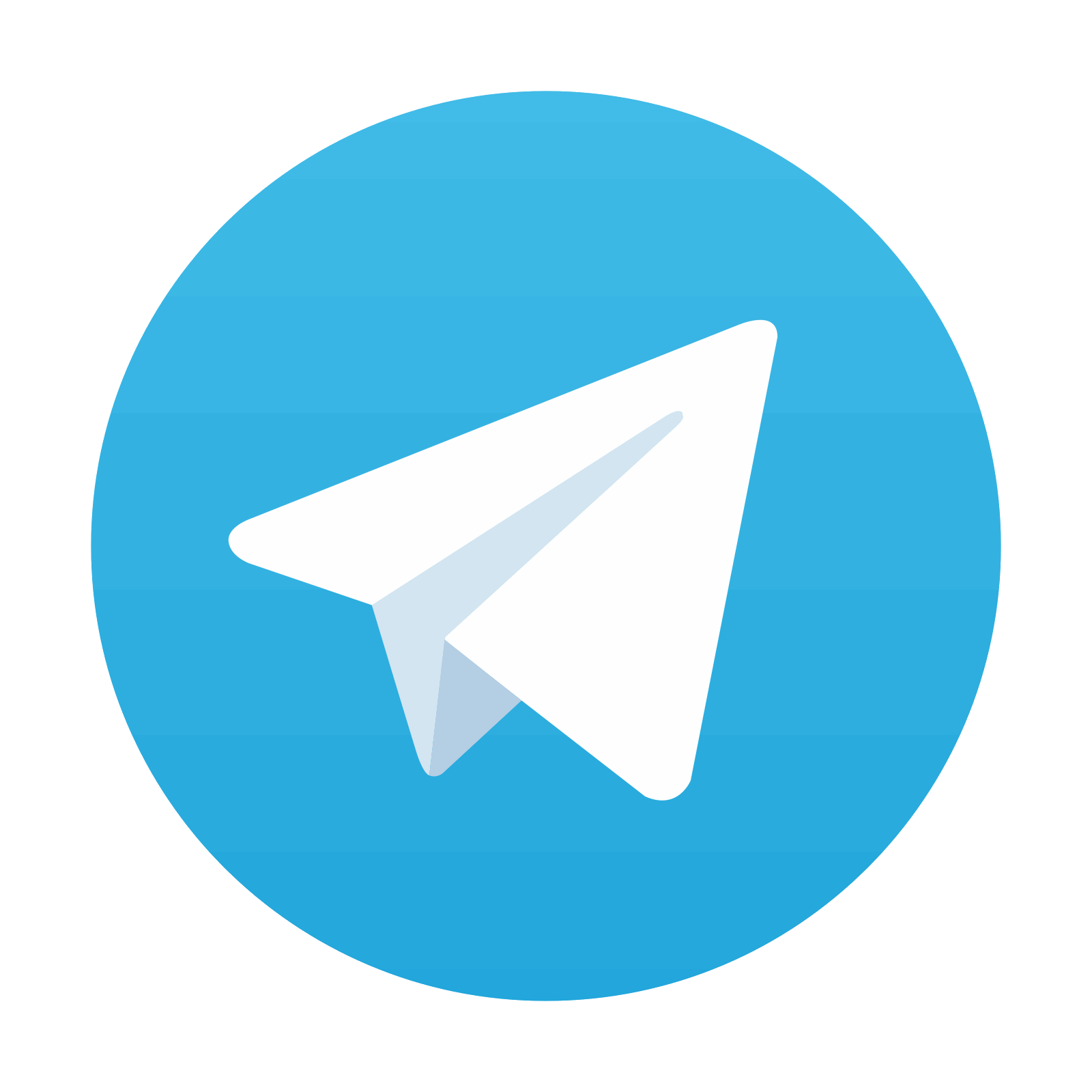
Grade 3–4 renal toxicity after PRRT
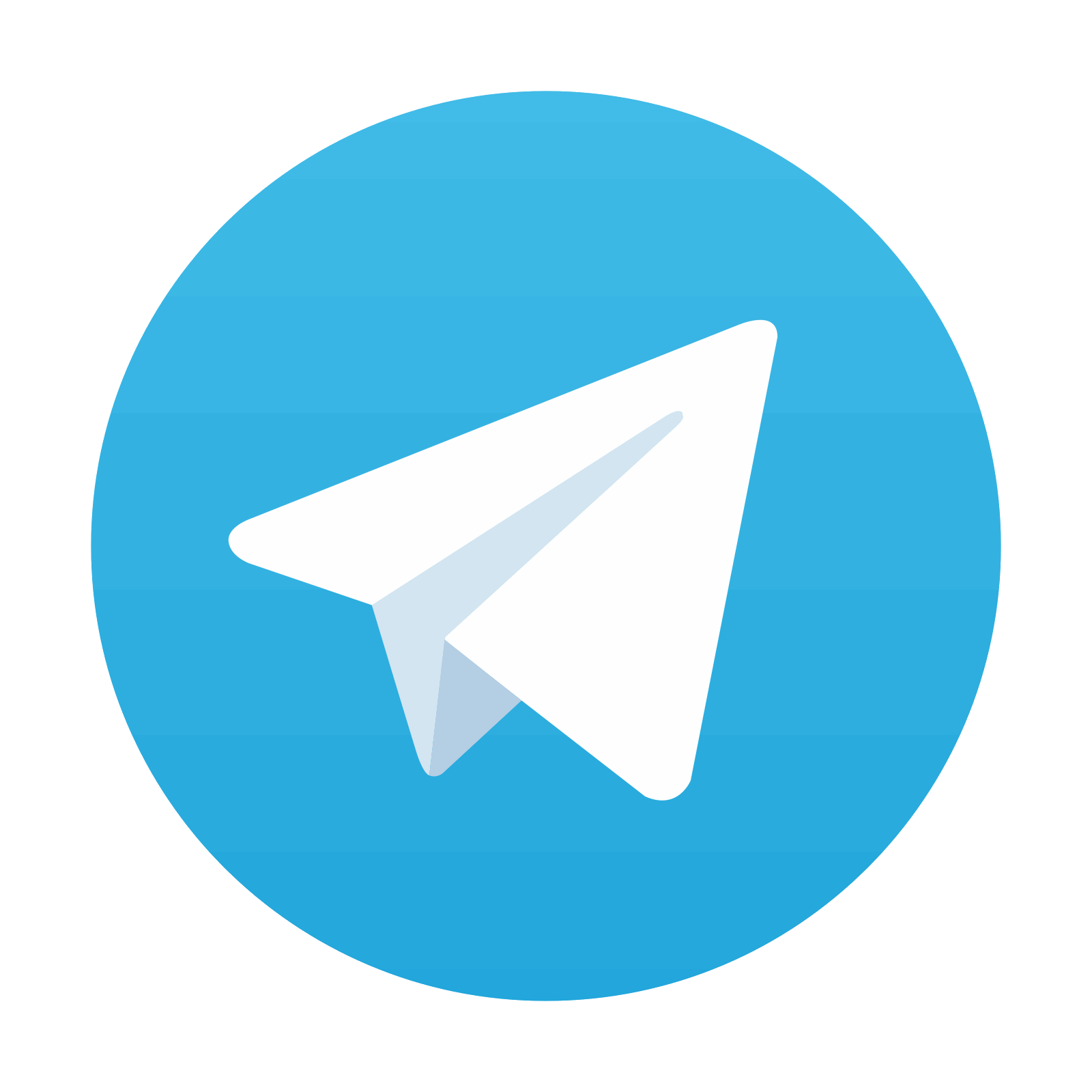
Stay updated, free articles. Join our Telegram channel

Full access? Get Clinical Tree
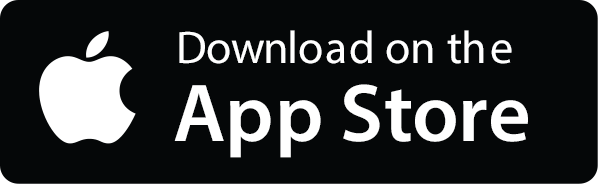
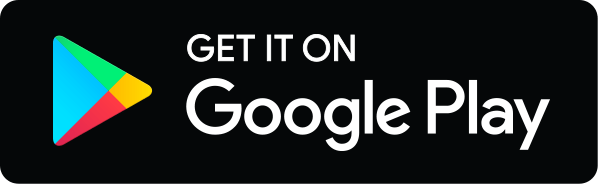
