Puberty is the process through which children attain adult secondary sexual characteristics and reproductive capability. In humans, two distinct processes of sexual maturation are recognized: gonadarche and adrenarche. Gonadarche is defined as the growth and maturation of the gonads, resulting in increased sex steroid secretion (most notably testosterone in boys) and spermatogenesis. Gonadarche requires an intact hypothalamic-pituitary-gonadal (HPG) axis, and any disruption of this axis can result in temporary or permanent disorders of reproductive endocrine function. Adrenarche is defined as maturation of the adrenal cortex, leading to increased secretion of adrenal androgens and development of pubic and axillary hair, apocrine body odor, and acne. The physiologic foundations for puberty begin in utero with the development of the neurobiologic structures that comprise the hypothalamic-pituitary component of the HPG axis and with the differentiation and development of the gonads. The entire process, extending from fetal life through achievement of reproductive competence, results from the dynamic and coordinated interactions of an expanding list of genes, proteins, signaling molecules, endocrine and paracrine factors, and epigenetic events.
Development of the reproductive endocrine system
Development of the Gonadotropin-Releasing Hormone Neuronal Network
In postnatal life, the gonadotropin-releasing hormone (GnRH) neurons are located in the hypothalamus. These neurons produce intermittent discharges of GnRH into the hypophysial-portal circulation to stimulate the pituitary gonadotropes to synthesize and secrete the gonadotropins follicle-stimulating hormone (FSH) and luteinizing hormone (LH).
The development of the HPG axis is exceptional in that GnRH neurons develop in metazoan embryos outside the central nervous system (CNS). Immature GnRH neuronal precursors become detectable in the olfactory placode and in the anlage of the nose (vomeronasal organ) from an early embryologic stage (around the sixth week of gestation in human and embryonic days E10.5–E11 in mice) and then begin their complex journey toward the hypothalamus. The embryonic migration of GnRH neurons to the hypothalamus is key for the development of the neuroendocrine network that allows normal pubertal development.
The pathway along which the GnRH neurons travel includes scaffolds formed by olfactory, vomeronasal, and terminal nerves 3 and 4. Migratory GnRH neurons are known to have receptors for at least 20 signaling molecules. They receive a plethora of guidance and movement-inducing messages during their journey, which appear to be distinct depending on the stage of their migration. These signals may act directly on GnRH neurons or indirectly on olfactory axons, as disruption of the olfactory tract “scaffolds” themselves can disrupt GnRH migration. The molecular signals involved include adhesion molecules (e.g., neuronal cell adhesion molecule [N-CAM]), extracellular matrix molecules (e.g., heparin sulphotransferases), cytokines (e.g., leukemia inhibitory factor, hepatocyte growth factor), those controlling cell-to-cell interactions (membrane receptors [e.g., neuropilin-2]), and transcription factors (e.g., Ebf2,) as well as both chemoattractants and chemorepellents (e.g., Reelin). Gradients of chemokines (e.g., CXCL12) may be particularly important for promoting and guiding the movement of GnRH neurons. This combination of factors has a high degree of redundancy, such that loss of any one factor may not fully disrupt GnRH neuronal migration.
The neurons begin their migration in the nasal compartment in or around the vomeronasal organ, pass the cribriform plate and penetrate the CNS in close proximity to the olfactory bulbs, and finally migrate apposed to a subset of vomeronasal nerves that diverge caudally into the basal areas of the forebrain. At the end of their journey, GnRH neurons diverge from guiding axons to disperse into their final positions in the septohypothalamic region, including the diagonal of Broca, the medial septum, and the preoptic areas of the hypothalamus. Eventually, these neurons reach the hypothalamus where they extend projections to the median eminence to form a network, which completes this aspect of GnRH neuronal development.
The whole process of migration involves no more than a few hundred neurons per hemisphere in mice and several thousand in primates or humans. The absolute number of GnRH neurons required for pubertal development is not known, but there appears to be a degree of redundancy in the system. Rodent studies suggest that around 12% of the GnRH neuron population is sufficient for pulsatile gonadotropin secretion and puberty onset, whereas between 12% and 34% are required for estrous cycling and ovulation in adult female mice. In addition, adult Reeler mice (which were initially identified based on an ataxia phenotype) have significantly fewer GnRH neurons in the hypothalamus and display a phenotype of delayed pubertal maturation and low fertility.
GnRH neurons extend their neurites to the median eminence under the control of factors that remain mostly unknown. Fibroblast growth factor receptor 1 (FGFR1) signaling is known to be important for this process of axon extension, as evidenced by reduced projections to the median eminence in transgenic mice expressing a dominant negative FGFR1 (dnFGFR1) in GnRH neurons.
The final stage of GnRH neuronal development comprises functional activity. By the 15th week of human gestation, the GnRH pulse generator is modulating the function of the fetal gonadotropes. The entire HPG axis is functionally active for the first time during fetal development, and after a brief perinatal pause, it continues to function in infancy (during the so-called minipuberty ), until it enters a relative quiescent state, often referred to as the juvenile pause or prepuberty .
As discussed subsequently, pathological abnormalities of pubertal development have been identified with each aspect of GnRH neuronal development: (1) defects in the synthesis of GnRH, which result mainly from an abnormal migration of GnRH neurons from the olfactory placode toward the hypothalamus during the first trimester of fetal life; (2) defect in the maturation and function of the GnRH neuronal network; (3) loss of function of GnRH itself or its receptor also known as a defect of the bioactivity of GnRH 4 ( Fig. 18.1 ).
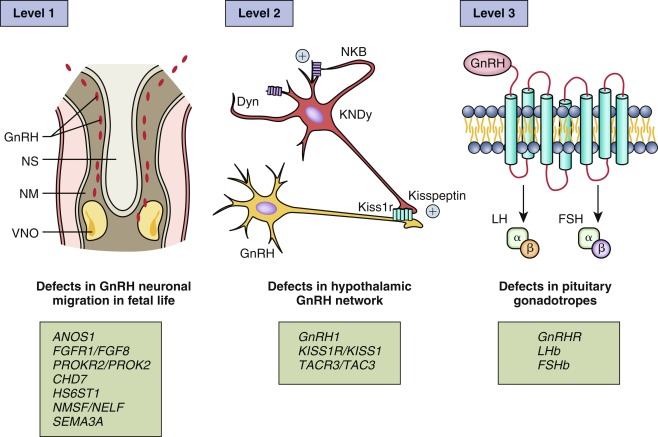
As a prismatic example , ANOS1 , previously known as KAL1 , encodes anosmin-1, an extracellular matrix protein that regulates axonal pathfinding and cellular adhesion. Anosmin-1 promotes branching of olfactory bulb neurons. Subjects with ANOS1 loss-of-function mutations have arrest of both olfactory bulb neurons and GnRH neurons at the level of the cribriform plate, resulting in abnormal sense of smell and hypogonadotropic hypogonadism (HH). Additional genetic factors involved in GnRH neuronal development and function are described in detail in the “Delayed Puberty, Hypogonadotropic Hypogonadism” section later in this chapter.
Pituitary Development
The pituitary consists of the anterior lobe (or adenohypophysis) and the posterior lobe (or neurohypophysis), as well as the pars intermedia and the infundibulum (or pituitary stalk). The anterior lobe derives from epithelial precursors/oral ectoderm and the posterior lobe derives from neural ectoderm, both under the control of a cascade of transcription factors. The spatial and temporal expression of these factors is critical, and mutations in the genes encoding these factors can lead to HH, as well as combined/multiple pituitary hormone deficiencies (see “Delayed Puberty” section later; reviewed in ).
Organogenesis of the pituitary gland begins during the fourth week of gestation with an upward extension of the oral ectoderm (to form Rathke’s pouch) toward the neural ectoderm. Simultaneously, the neural ectoderm of the ventral diencephalon extends downward, allowing for the connection of these two elements and leading to the eventual formation of the composite, two-lobe structure of the adult pituitary.
Initiation of organogenesis and formation of Rathke’s pouch involves signaling molecules, such as Hesx1, Otx2, Pitx1/2/3, Sox2, FGF8, and Lhx3. Subsequent cell lineage determination depends on molecules, such as Prop1, POU1F1 (Pit1), and Nr5a1(SF1) among others. Because of the complex pattern transcription factor control, different molecules are key to the development of different cell lineages at different times. For example, Lhx3 and Hesx1 affect the development of all lineages as does Pitx1/2/3; Prop1 affects differentiation of gonadotrophs, thyrotrophs, somatotrophs, lactotrophs but perhaps plays a less independent role in development of corticotrophs; POU1F1 regulates differentiation of thyrotrophs, somatotrophs, and lactotrophs; Nr5a1 largely affects gonadotrophs. As noted, in the absence of functional redundancy, mutations in the genes for these regulatory factors can lead to pituitary hormone deficiencies, with the hormones affected largely predicted by the cell types whose development is dependent on the particular transcription factor. However, some mutations, such as in HESX1 or OTX2 , can lead to combined pituitary hormone deficiencies, as expected, but also to isolated growth hormone (GH) deficiency and to septooptic dysplasia.
The pituitary develops early in gestation and, similar to the GnRH neuronal network, becomes functional in utero. The gonadotrophs are the last anterior pituitary cell types to develop, but gonadotropin release is detectable by week 14 in response to GnRH secretion. Gonadotropin secretion peaks around 20 to 22 weeks in utero and subsequently increases and decreases in accordance with the dynamic hypothalamic GnRH secretion, described earlier, and with positive and negative feedback loops, described later.
Testicular Development
A brief summary of prenatal testicular differentiation and development follows; a more detailed description of testicular differentiation and sex development can be found in Chapter 6 . Beginning at approximately 4 to 6 weeks of gestation, the primordial bipotential gonad arises from a condensation of the mesoderm of the urogenital ridge. During this time, the primordial germ cells proliferate and migrate from the hindgut to colonize the developing gonad. In the human male fetus, the testicular compartments, tubular and interstitial components, and specific cell types, Leydig, Sertoli, and germ cells can be visualized by 11 weeks of gestation. During early gestation, placental human chorionic gonadotropin (hCG) governs Leydig cell proliferation and secretion of testosterone and insulin-like factor 3 (INSL3); endogenous LH begins to regulate these activities in midgestation. Because of the role of placental hCG during early gestation, gonadotropin deficiency does not influence male sexual differentiation. However, LH secretion does influence the number of fetal Leydig cells, as demonstrated by a reduced number in anencephalic fetuses and an increased number in 46,XY fetuses who have elevated gonadotropin concentrations secondary to complete androgen insensitivity. Testosterone production secondary to fetal LH secretion is also critical for phallic growth during the second and third trimesters; males with LH deficiency typically have normally formed but small penises at birth. FSH secretion influences Sertoli cell differentiation and anti-Mullerian hormone (AMH) and inhibin B secretion.
Testicular descent occurs in two phases. The transabdominal phase begins at approximately 12 weeks of gestation and is influenced by the Leydig cell product, INSL3, and its cognate receptor, leucine-rich repeat-containing G-protein–coupled receptor 8 (LGR8). The second androgen-dependent phase, descent of the testes through the inguinal canal, is usually accomplished by the end of week 35.
Following a brief pause in activity after birth, the hypothalamic-pituitary-testicular axis is active during the first few months of life with testosterone concentrations peaking at 1 to 2 months of age. By approximately 6 months of age, testosterone concentrations decline to prepubertal levels. During the brief period of increased neonatal HPG activity, sexual hair does not develop and gametogenesis does not occur because of limited androgen receptor (AR) signaling in certain tissues (e.g., Sertoli cells). During infancy and childhood, seminiferous cords are solid and generally filled with immature Sertoli cells. The germ cells are limited to spermatogonia and Leydig cells are rarely visualized. Inhibin B and AMH continue to be secreted during childhood and serve as valuable markers of Sertoli cell function.
Physiology of puberty
Hypothalamic KNDy and Gonadotropin-Releasing Hormone Neurons
GnRH release is coordinated by inhibitory and excitatory neuronal and glial inputs ( Fig. 18.2 ). Retrograde tracing studies in mice have demonstrated that GnRH neurons are controlled by a complex neuronal network of inputs from many regions of the brain, including the brain stem, limbic system, hypothalamic nuclei, motor and sensory circuits, and basal ganglia.
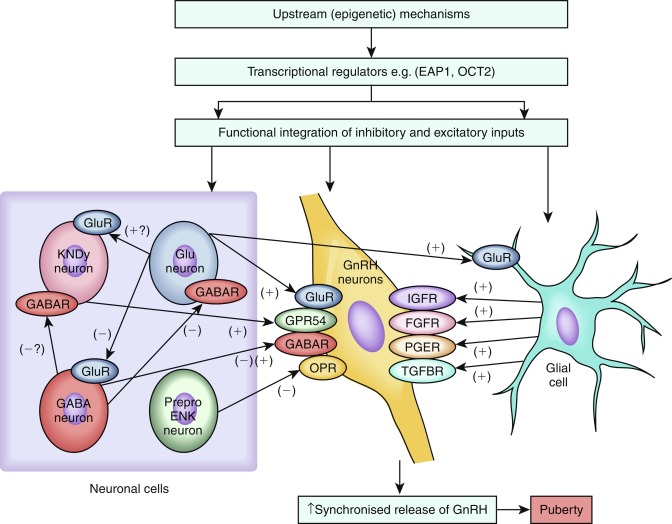
Among the various regulators of GnRH neurons, kisspeptins and neurokinin B are the most essential. The distribution of kisspeptin neurons in the hypothalamus varies with species. Kisspeptin neurons have been anatomically mapped in mice to reside within the hypothalamus caudally, in the mediobasal hypothalamus, which includes the arcuate nucleus (ARC)/infundibular region. In mice, a second population is located in the rostral part of the hypothalamus called the anteroventral periventricular ( AVPV ) region. In rats, monkeys, and humans, this regional distribution of kisspeptin neurons is less clear. The two populations of kisspeptin neurons in mice exhibit marked functional differences, with, for example, the AVPV kisspeptin neurons having a role in mediating the positive-feedback effects of estrogen, leading to the GnRH/LH surge that triggers ovulation, and the ARC kisspeptin neurons having a role in mediating negative-feedback effects of sex steroids on tonic activity of the HPG axis. AVPV kisspeptin neurons exhibit clear sexual dimorphism, with female rodents at puberty having a much greater number of kisspeptin neurons in this area.
Kisspeptin, an excitatory neuropeptide, was identified as a vital permissive factor in puberty onset by the discovery of patients with GnRH deficiency with loss-of-function mutations in the kisspeptin receptor, KISS1R , previously known as GPR54. Subsequently, an activating mutation in KISS1R was identified in a girl with central precocious puberty, and an activating mutation in KISS1 , which encodes kisspeptin itself, has been reported in a child with central precocious puberty. Moreover, mice with knockout of Kiss1r are infertile, despite normal distribution of GnRH neurons and normal hypothalamic GnRH levels, and mice with Kiss1 knockout also have a phenotype consistent with normosmic GnRH deficiency.
Kisspeptins are synthesized by hypothalamic neurons that contact GnRH neurons and their projections. Kisspeptin neurons are located outside the blood-brain barrier and therefore come directly in contact with peripheral sex hormones. Most GnRH neurons express the kisspeptin receptor and kisspeptin neurons express steroid receptors (estrogen receptor alpha, androgen receptor, and progesterone receptor) and are the main relay for the negative and positive feedback of steroid hormones on the gonadotropic axis.
Kisspeptin signals directly to GnRH neurons to control pulsatile GnRH release. Kisspeptin is upregulated in both primates and mice in the peripubertal period, and its administration to pubertal rodents advances the onset of sexual maturation. Interestingly, kisspeptin also appears to be downregulated in functional amenorrhea, suggesting its role as a mediator of environmental factors, such as emotional well-being and nutritional status. In addition, as noted, kisspeptin has been shown to be an important neuroendocrine regulator of ovulation. In mice, kisspeptin neurons located in the AVPV mediate the positive feedback of estradiol on the GnRH neuronal network and therefore the generation of the LH preovulatory pulse. In contrast, estrogens have an inhibitory effect on Kiss1 neurons in the ARC. Thus kisspeptin signaling appears to have both positive and negative feedback loops in the HPG axis. Whilst kisspeptin has been identified as a pivotal upstream regulator of GnRH neurons, whether kisspeptin is the key factor in triggering the onset of puberty remains unclear.
Another excitatory neuropeptide, neurokinin B, has been implicated in the upstream control of GnRH secretion. Identification of this pathway was based on discovery of loss-of-function mutations in TAC3 , encoding neurokinin B, and its receptor TACR3 , in patients with pubertal failure caused by normosmic HH. Kisspeptin neurons located in the ARC synthesize neurokinin B and dynorphin A and have been called KNDy ( K isspeptin, N eurokinin B, Dy norphin) neurons. Both TAC3 and KISS1 expression in the ARC is downregulated by estrogen. Therefore these neurons are considered as the relay of the negative feedback of steroid hormones on the gonadotropic axis. KNDy neurons also express the neurokinin B receptor, NK3R, suggesting that paracrine and autocrine loops control GnRH release. Dynorphin inhibits the release of GnRH, and together these peptides are currently believed to play a fundamental role in regulating the pulsatile GnRH release.
More recently, another RF-amide–related peptide (RFRP1, RFRP3), the mammalian orthologue of the avian peptide gonadotrophin-inhibiting hormone (GnIH) , was discovered as another inhibitory regulator of the gonadotropic axis by directly controlling GnRH neurons. GnIH plays an essential role in the inhibition of the HPG axis in several species. In addition to neuropeptides, several neurotransmitters participate in the control the GnRH network. Gamma aminobutyric acid (GABA) and glutamate control GnRH neuronal excitability in the ARC. In female rats, glutamine synthase is downregulated and glutamate dehydrogenase becomes more abundant in the hypothalamus at puberty, both leading to increased availability of glutamate, which acts through its n-methyl-D-aspartate (NMDA) and kainate receptors. The administration of glutamate agonists to prepubertal primates can stimulate the onset of puberty.
The neural network of GABA is quite complex because some of these neurons will have a direct effect on GnRH neurons, and others will act on interneurons. The inhibitory role of GABAergic neurotransmission in restraining the initiation of puberty has been clearly shown in primates but is more ambiguous in rodents. GABAergic signaling pathways are likely to be important in the stress-induced suppression of LH.
Some recent evidence highlights the importance in mice of micro ribonucleic acid (microRNAs) (particularly the miR-200/429 family and miR-155) in the epigenetic upregulation of GnRH transcription during the murine equivalent of the minipuberty. Moreover, miR-7a2, has been demonstrated to be essential for normal pituitary development and HPG function, with deletion in mice leading to HH and infertility.
Corticotropin-releasing hormone (CRH) signaling has long been known to play an important role in the stress-induced suppression of the GnRH pulse generator in rodents. Intracerebroventricular (ICV) administration of CRH decreases LH pulse frequency in rats, whereas stress-induced suppression of LH pulses by insulin-induced hypoglycemia is blocked by ICV administration of a CRH antagonist. Juxtaposition of CRH immunoreactive fibers to GnRH neurons has been observed in the infundibular region of the hypothalamus in the human.
Finally, the synchronous pulsatile secretion of GnRH is also controlled through neuron-glia signaling pathways. Glial inputs appear to be predominantly facilitatory and they consist of growth factors and small diffusible molecules, including transforming growth factor (TGF)β1, insulin-like growth factor 1 (IGF-1), and neuregulins, that directly or indirectly stimulate GnRH secretion. Glial cells in the median eminence regulate GnRH secretion by production of growth factors, acting via receptors with tyrosine kinase activity. FGF signaling is required for GnRH neurons to reach their final destination in the hypothalamus, as well as for GnRH neuronal differentiation and survival. In addition, GnRH neuron secretory activity is facilitated by IGF-1 and by members of the epidermal growth factor family, such as neuregulin 1β. Plastic rearrangements of glia-GnRH neuron adhesiveness, mediated by soluble molecules, such as N-CAM and synaptic cell adhesion molecule (SynCAM), coordinate the controlled delivery of GnRH to the portal vasculature, a process that is also subject to sex steroid regulation.
Pituitary Gonadotropins
GnRH stimulates the production and secretion of LH and FSH from the gonadotrophs by binding to a cell-surface receptor, which triggers increased intracellular calcium concentration and phosphorylation of protein kinase C. LH is released from readily releasable pools, which lead to a rise in serum LH within minutes after a bolus of GnRH, but also from pools which take longer to mobilize. Whereas episodic stimulation by GnRH increases gonadotropin secretion, continuous infusion of GnRH decreases LH and FSH secretion and downregulates the pituitary receptors for GnRH—a phenomenon that is used in the medical treatment of central precocious puberty. Alterations in the GnRH receptor have other important roles in regulating gonadotroph function as estrogens increase and androgens decrease GnRH receptors.
FSH and LH are glycoproteins composed of two subunits, an α subunit that is common for all the glycoprotein hormones and distinct β subunits that confer receptor specificity. The β subunits are 115 amino acids long with two carbohydrate side chains. hCG produced by the placenta is almost identical in structure to LH except for an additional 32 amino acids and additional carbohydrate groups. Rare cases of mutations in the β subunit of gonadotropin molecules that cause pathological effects have been reported: a single case of an inactivating mutation of LHB caused absence of Leydig cells and lack of puberty in a male and two cases of inactivating mutations of FSHB led to lack of follicular maturation and amenorrhea and, in two males, azoospermia. In addition, a woman with a homozygous mutation in a 5′ splice-donor site in the noncoding region of LHB displayed impaired LH secretion, normal pubertal development, secondary amenorrhea, and infertility. What is conceptually important from these observations is that normal pubertal maturation in women, including breast development and menarche, can occur in a state of LH deficiency, whereas normal LH secretion appears obligatory for ovulation. This implies that although LH is essential for the normal maturation of Leydig cells and steroidogenesis in men, its primary role in women is to induce ovulation.
The same gonadotroph cell produces both LH and FSH in the pituitary. Gonadotroph cells that are not stimulated, for example, because of disease affecting GnRH secretion, are small in diameter, while the gonadotroph cells in primary hypogonadism, stimulated by large amounts of GnRH, are large in diameter and demonstrate prominent rough endoplasmic reticulum.
Gonadal Hormone Production
Sex Steroids
The LH stimulation of Leydig cells leads to increased testicular testosterone secretion, and typical Leydig cells become apparent on histology. Testosterone acts as a paracrine factor to induce Sertoli cell maturation. Pubertal maturation of the seminiferous tubules is characterized by cytoskeletal rearrangements, including development of tight junctions, Sertoli cell polarization, Sertoli cell proliferation, migration of spermatogonia toward the basement membrane, and decreased AMH secretion.
The Leydig cells of the testes synthesize testosterone through a series of enzymatic conversions for which cholesterol is the precursor. When LH binds to Leydig cell membrane receptors, the ligand-receptor complex stimulates membrane-bound adenyl cyclase to increase cyclic adenosine monophosphate (cAMP), which then stimulates protein kinase A, which in turn causes the stimulation of the conversion of cholesterol to pregnenolone by P450scc (side-chain cleavage enzyme), the first step in the production of testosterone. With substantial exposure to LH, the number of receptors for LH and the postreceptor pathway decrease their responsiveness to LH for at least 24 h. This explains the clinical finding of insensitivity to LH after daily injections of LH or hCG compared with every-other-day injections. When assessing the response of testes to LH, hCG or LH must be administered at 2- to 3-day intervals to avoid such downregulation.
When testosterone is secreted into the circulation, the majority is bound to sex-hormone–binding globulin. The remaining free testosterone (95% of which is bound to albumin with low affinity) is conventionally considered the active moiety. At the target cell, testosterone dissociates from the binding protein, diffuses into the cell, and may be converted by 5a-reductase type 2 (a surface enzyme located on the genital skin and elsewhere and encoded by a gene on chromosome 2) to dihydrotestosterone or by aromatase (CYP19) to estrogen. Testosterone or dihydrotestosterone binds to the androgen receptor that is encoded by a gene on the X chromosome (Xq11-q12). The testosterone/dihydrotestosterone-receptor complex then attaches to the steroid-responsive region of genomic deoxyribonucleic acid (DNA) to initiate androgen-dependent transcription and translation, ultimately leading to virilization. However, androgens must be converted to estrogens to stimulate bone maturation at the epiphyseal plate.
FSH binds to specific receptors on the cell surface of Sertoli cells and causes a sequence of events that culminates in increased protein kinase A activity in a manner similar to the stimulatory effect of LH on Leydig cells. However, FSH causes an increase in the mass of seminiferous tubules, and in an undefined way supports the development of sperm. The increase in Sertoli cell number contributes to the increase in testicular volume that provides a physical marker of the onset of gonadarche in boys. As puberty progresses, the seminiferous tubules enlarge and develop a lumen. Although much variation in chronologic age and testicular volume has been described, spermarche (initial sperm production) precedes peak pubertal linear growth velocity and occurs at a median testicular volume of 10 to 12 mL, although sometimes as low as 4 mL.
Activin and Inhibin B
FSH stimulates Sertoli cells to secrete inhibin B, which from midpuberty onward serves as the major negative regulator of pituitary FSH secretion. Before puberty, inhibin B is germ cell independent. After puberty, inhibin B secretion becomes germ cell dependent and provides a marker of germ-cell integrity in adults.
Inhibin B is a heterodimeric glycoprotein member of the TGF-β family produced in males exclusively by the testis, primarily in the prepubertal testis by the Sertoli cells, and by the ovarian granulosa cells and the placenta in the female. Several studies show that serum inhibin-B levels in children change in concert with the secretion of gonadotropins. During the “minipuberty” serum inhibin-B levels increase to similar or higher levels to those observed in adolescent boys and adult men. This early inhibin-B secretion is sustained until the age of 18 to 24 months; thereafter, serum concentrations decline to lower but readily measurable levels. Early in puberty, between Tanner stages G1 and G2, serum inhibin-B concentrations again increase and reach peak levels at Tanner stage G2, but then the levels plateau. Inhibin suppresses FSH secretion from the pituitary gland and provides another explanation for different serum concentrations of LH and FSH with only one hypothalamic peptide (GnRH) stimulating them. Activins are homodimers of the β subunit of inhibin and have the opposite effect, stimulating the secretion of FSH from the pituitary gland. Absence of inhibin B because of gonadal failure causes a greater rise in serum FSH than LH in pubertal and adult subjects.
Anti-Mullerian Hormone
AMH belongs to the same TGF-β family as inhibin and is produced from the Sertoli cells of the testes from the time of testicular differentiation to puberty, and in females by the granulosa cells from birth until menopause. In normal males, AMH is high in the fetus and newborn with peak levels around 2 months of age and then decreases by the age of 1 year. Patients with dysgenetic testes have decreased serum AMH, whereas values are elevated in Sertoli cell tumors and females with granulosa-cell tumors. Undetectable AMH and inhibin B are characteristic of congenital anorchia but may also be seen in severe hypogonadotropic hypogonadism in males. In infant girls, a similar pattern in AMH levels during the first months of life has been reported, but the levels in girls are significantly lower. AMH decreases during puberty as a sign of androgen action. Beginning in early puberty, testosterone represses AMH secretion by Sertoli cells, and testosterone and AMH begin to show an inverse relationship. Intratesticular testosterone and signaling through androgen receptors in Sertoli cells are essential for the decline in AMH concentrations, meiosis, and spermatogenesis. Hence, AMH concentrations provide an indication of Sertoli cell function and androgen action in the testes.
Putting It All Together: the Pubertal Reactivation of the Gonadotropic Axis
Hormonal Changes
The gonadotropic axis undergoes complex cycles of activation and inhibition from fetal life to puberty ( Fig. 18.3 ).
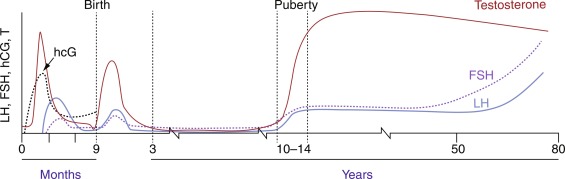
Fetal testosterone secretion early in pregnancy is caused by placental hCG stimulation. The fetal hypothalamus contains GnRH-containing neurons by 14 weeks of gestation, and the fetal pituitary gland contains LH and FSH by 20 weeks. The hypothalamopituitary portal system develops by 20 weeks of gestation, allowing hypothalamic GnRH to reach the pituitary gonadotrophs. At midgestation, there is a striking rise of circulating gonadotropin levels in both male and female fetuses, which reaches its peak at 34 to 38 weeks in the male fetus, and then falls to low levels at birth. This change in gonadotropin secretion results from the development of a negative feedback system through sex steroids, as well as from the development of inhibiting influences from the CNS on GnRH neurons.
LH and FSH secretion rise during the first month after birth, probably because the negative feedback effect of placental estrogens is withdrawn. LH is secreted in pulses during this postnatal period, often termed the minipuberty . After this postnatal activity, the HPG axis becomes relatively dormant in children between the age of 2 and 8 to 9 years.
The transition from the relative prepubertal quiescence to the adolescent pattern of GnRH secretion is a gradual rather than abrupt process. LH and FSH pulsatility has been detected in normal children as young as 4 years of age. Throughout childhood, GnRH secretion appears to undergo small but progressive increases until the onset of puberty, when GnRH secretion increases, first at night and eventually throughout the day. Because of the episodic nature of gonadotrophin secretion, a single gonadotrophin determination is not informative regarding the secretory dynamics of these hormones. However, modern third-generation assays are sufficiently sensitive to indicate the onset of puberty in single basal unstimulated samples.
The first biological change demonstrating that the HPG axis is being reactivated at puberty is the augmentation of nocturnal LH pulses in children; this reactivation is subclinical and begins before clinical development of Tanner genital stage 2. This period may thus be seen as the hormonal onset of puberty. The differences between daytime and nighttime levels of LH remain until late stages of puberty but disappear by early adulthood. During this reactivation of the axis, there is a gradual development of a dynamic interplay between the central production of GnRH and gonadotropins and gonadal sex-steroid production. A progressive maturation of negative-feedback loops occurs but so too the prepubertal suppressant drive from the CNS gradually abates, and transient intensifying positive feedback results from increasing gonadal sex-steroid production.
During the period of relative quiescence, testosterone and estrogen are also measurable in the circulation using sensitive assays, demonstrating low but definite activity of the prepubertal gonads. However, gonadal contribution to the inhibition of the hypothalamic-pituitary system occurs later, becoming operative only at midpuberty, and eventually becomes dominant over the central inhibitory feedback drive ( Fig. 18.4 ). Both mean LH and FSH levels increase through pubertal development, although LH rises to a greater extent, probably because of differences in feedback mechanisms for these two hormones. These rises are the result of both an increase in basal levels of LH and FSH, and a greater number and amplitude of LH peaks.
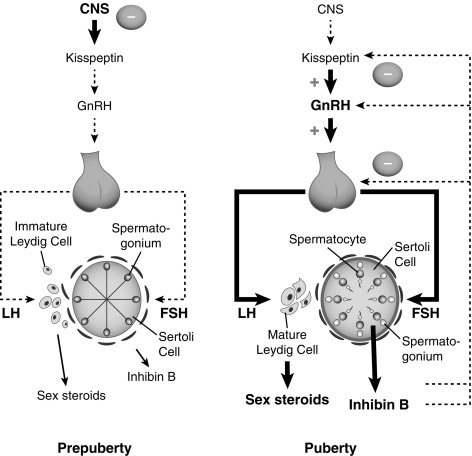
In boys during puberty, plasma testosterone levels increase dramatically. The pubertal increase in testis size results primarily from an increased number of proliferating and differentiating germ cells and, to a lesser extent, an increase in Sertoli cells. In early and midpuberty, there is a pronounced diurnal rhythm with a morning peak in measurable testosterone, but this is less pronounced in later puberty and declines gradually with age, probably because of decreased day-night ratios of gonadotropins.
The biological reactivation of the gonadotropic axis occurs earlier in girls than boys, and the dynamic of the reactivation of the gonadotropic axis is not identical in the two sexes. The secretion of testosterone increases shortly after the increase in the plasma concentration of LH and FSH. In girls, estradiol increases together with increasing LH and FSH. Then, a hormonal dialogue between gonads, the hypothalamus, and pituitary contributes to the progressive activation of the gonadotropic axis, until the occurrence of the LH ovulatory pulse in females. The basis for this sexual dimorphism is unclear and may be related to differences in gonadal hormone production and could also be a feature of the sexual dimorphism of the brain. There are also some data suggesting that although most genes regulate the timing of puberty similarly in boys and girls, there is a small subset that may exert sex-specific effects.
Physiological Mechanisms
Our understanding of the activation of the gonadotropic axis at the end of the prepuberty remains incomplete but has advanced in some areas (see discussion later). GnRH neuronal activity is under the control of several neurotransmitters and neuropeptides, as described earlier, and the onset of puberty is triggered by a decline in these inhibitory signals and amplification of the excitatory inputs, leading to increased frequency and amplitude of GnRH pulses. The neuroendocrine mechanisms that control the activation of the gonadotropic axis in the fetus, as well as during the “minipuberty” are less well understood. Likewise, the exact nature of the brake that functions during prepuberty remains unknown.
One change at puberty is a shift in the balance of GABA-glutamate signaling in the brain. Another is an increase in dendritic spine density and a simplification of the dendritic architecture of GnRH neurons. A third is an increase in kisspeptin signaling in the hypothalamus, which is caused by an increase of kisspeptin synthesis, as well as an increased responsiveness of GnRH neurons to kisspeptin stimulation. Although mainly described in mice, this paradigm, which is well conserved in evolution, is probably true in monkeys as well in humans.
The mechanisms responsible for the increased biosynthesis of kisspeptins in the hypothalamus at the end of the juvenile period remain unknown. Data pointing to hypothalamic regulation via a hierarchical network of genes (see Fig. 18.2 ) have mainly come from a systems biology approach and animal models, with little data from human subjects to date; however, integration of findings from human genome-wide association studies will likely enrich these models. Candidate transcriptional regulators that have been identified via these approaches include octamer transcription factor 2 ( Oct-2 ), thyroid transcription factor-1 ( TTF-1 ), and enhanced at puberty 1( EAP1 ). TTF-1 is a homeobox gene that enhances GnRH expression. TTF-1 expression is increased in pubertal rhesus monkeys. Oct-2 is a transcriptional regulator of the POU-domain family of homeobox-containing genes. Oct-2 messenger RNA (mRNA) is upregulated in the hypothalamus in juvenile rodents; blockage of Oct-2 synthesis delays age at first ovulation; and hypothalamic lesions, which induce precocious puberty (e.g., hypothalamic hamartomas), activate Oct-2 expression. EAP1 mRNA levels also increase in the hypothalamus of primates and rodents during puberty, EAP1 transactivates the GnRH promoter, and EAP1 knockdown with small interfering RNA (siRNA) causes delayed puberty (DP) and disrupts estrous cyclicity in rodents.
Recent data have highlighted the importance of a genetic program that controls the expression of Kiss1. The intervention of the polycomb complex proteins, EED and Cbx7, in the transcriptional repression of Kiss1 has recently been revealed. The expression of these genes in the prepubertal period progressively decreases with increasing methylation of their promoters. Thus the binding of EED on the Kiss1 promoter decreases at puberty. The inhibition of the repression of Kiss1 is also correlated with a decrease in the expression of transcription factors with zinc-finger motifs. In addition, a microRNA switch was proposed to regulate the rise of GnRH1 synthesis, which occurs during the juvenile period in GnRH neurons. The initiation of puberty from the hypothalamus therefore results from a complex network of transcription factors mainly acting as repressors of Kiss1 and GnRH1 transcription.
The concept that puberty results from the disappearance of gonadotropic axis repression is also supported by the description of loss-of-function mutations of MKRN3 and DLK1 in familial central precocious puberty (CPP, discussed in detail in “Precocious Puberty” later). Where these factors function in the hierarchical network of genes controlling kisspeptin has yet to be determined.
Somatic Changes
In boys, the first physical finding that marks the onset of puberty is the change from Tanner genital stage G1 to stage G2, including enlargement of the testes (i.e., achievement of volume ≥ 4 mL or testicular length ≥ 25 mm). Originally Marshall and Tanner reported the mean (standard deviation [SD]) onset of puberty in boys to be 11.64 (1.07) years. These pubertal stages ( Fig. 18.5 ) were based on longitudinal photographic observations of genital development of a relatively small sample of 228 boys living in a children’s home. Despite the probably poor representative nature of this sample, studies in Switzerland, the United States, and Denmark have reported roughly similar mean ages of puberty onset.

Although the mean age of onset may be fairly uniform across populations, the range of ages for onset of puberty in normal, healthy adolescents varies widely. Several pathologic states may further influence the timing of puberty either directly or indirectly and may contribute to this splay, but the great majority of the variation in pubertal timing cannot be attributed to clinical disorders. Some 95% of boys experience the onset of genital development between 9.5 and 13.5 years, and these data have led to the traditional definition of sexual precocity in boys as development of secondary sexual characteristics before age 9 years and DP as lack of testicular enlargement by age 14 years.
Development of secondary sexual characteristics results from both gonadarche and adrenarche. Adrenarche refers to the maturation of the zona reticularis of the adrenal gland, resulting in increased production of the adrenal androgens dehydroepiandrosterone (DHEA) and androstenedione, as well as the relatively inactive metabolite DHEA sulfate (DHEA-S). These adrenal androgens, along with testicular androgens, contribute to secondary sexual characteristics, such as pubic hair (pubarche), axillary hair, apocrine body odor, and acne. Like gonadarche, the onset of adrenarche appears to be a gradual, progressive maturational process that begins in early childhood and is marked by the further increases of production of adrenal androgens around the time of puberty. Although adrenarche and gonadarche typically overlap, they are separate processes that are independently regulated. The triggers for adrenarche remain unknown; however, alterations in body weight and body mass index (BMI), as well as in utero and neonatal physiology, likely modulate this developmental process, perhaps along with intraadrenal cortisol production.
Regulation of the timing of puberty
Secular Trends in the Timing of Puberty
The mean age of menarche in mid-nineteenth century Europe is reported to be between 17 and 18 years. Starting from the late nineteenth century to the mid-twentieth century, a gradual decline in age at puberty has been reported, after which this trend appears to have slowed. Much of this change in the timing in puberty has likely been the result of better hygiene and nutrition related to increased socioeconomic stability.
More recent declines in the age at puberty are more convincingly demonstrated among girls than in boys, In the mid-1990s, data from the Third National Health and Nutrition Examination Survey (NHANES III), where genital ratings were performed by visual inspection, reported earlier age at puberty in both boys and girls, than what previously had been reported from the United States. Using the traditional cutoff of 9 years, these data suggest that an increased number of boys would be classified as having precocious puberty. However, because of lack of data on pubertal onset in the previous population-based study (Third National Health Examination Survey [NHES III]), some controversy remained as how to interpret the NHANES III findings.
Furthermore, questions have been raised regarding the criteria used for genital staging in NHANES III. A subsequent secular trend analysis between NHES III (which lacked data from the early pubertal stages) and NHANES III did not find clear evidence supporting earlier age at puberty, although some indications were present in non-Hispanic white boys. These data were also reviewed by an expert panel, which concluded that the available data are insufficient in quality and quantity to confirm a change in pubertal timing in US boys. At the same time in Europe, in comparison with NHANES III studies, some data even reported suggesting older ages at pubertal onset in boys.
Secular trends in the timing have been assessed in a few European studies within specific populations. Earlier studies do not support a substantial enough change in the age at pubertal onset in boys from the mid-1960s to the late 1990s to warrant a change in the age definitions for precocious and DP. However, the most recent data from Copenhagen, comparing the timing of puberty in boys assessed between 2006 and 2008 compared with a group assessed between 1991 and 1993, does report earlier mean age of onset of puberty (11.62 vs. 11.92 years).
In summary, data are conflicting but there is likely an overall suggestion that puberty may be occurring earlier in boys than in the past. However, the change is not as dramatic as in girls, and age cutoffs used to define disorders of puberty have not been uniformly changed.
Genetic Contributions to Variation in Normal Puberty
Although the precise mechanisms that trigger puberty remain unknown, it is well established that the timing of puberty is influenced by both genetic and environmental factors. Evidence for the genetic contribution is provided by correlations in timing within families and between monozygotic twins. It is important to note that this genetic component does not preclude a significant role for environmental influences; indeed, genetics alone cannot explain secular trends in the timing of puberty. However, the strong genetic component provides an opportunity to identify factors that modulate the timing of normal puberty in the general population. Approaches used to identify specific genetic factors include candidate-gene studies and genome-wide association (GWA) studies.
Candidate Gene-Based Studies
One approach for identifying the variants that affect complex traits, such as the timing of puberty in the general population, has been candidate gene-based association studies, which seek to demonstrate a higher prevalence of genetic variants in one or more prespecified genes in cases than in controls.
In an association study that tested for associations between common variants in 10 HH-related genes ( GNRH1 , GNRHR , KISS1R/GPR54, KISS1, LEP, LEPR, FGFR1, KAL1, PROK2 , and PROKR2 ) and age at menarche, only nominally significant associations between single nucleotide polymorphisms (SNPs) in several of the genes and age at menarche were identified.
Other work similarly did not identify associations between SNPs in GNRH1 and GNRHR or LEP and LEPR and alterations in pubertal timing. However, large-scale GWA studies (see later) have demonstrated that variants in some of these genes do in fact influence pubertal timing and have revealed relatively small effect sizes of these variants, such that earlier candidate-gene studies likely had insufficient power to detect these small effect sizes.
Genome-Wide Association Studies
In large-scale GWA studies, the age of menarche has been the most commonly used marker of the timing of puberty. The first GWA studies on age of menarche involved between 17,000 and 25,000 women, all of European descent. Common variants in LIN28B were associated with age at menarche in four independent GWA studies and one metaanalysis. LIN28B is a human homologue of lin-28 , which in Caenorhabditis elegans controls the rate of progression from larval stages to adult cuticle formation, indicating conservation of specific micro-RNA regulatory mechanisms involved in developmental timing. In each case, age at menarche (AAM) was analyzed, but in one study, additional phenotypes (breast development in girls, voice breaking and pubic hair development in boys, and tempo of height growth in both boys and girls) were found to associate with variants in LIN28B , suggesting that LIN28B affects timing of puberty more generally, not just timing of menarche, and that control of pubertal timing in boys and girls shares some common elements, although subsequent studies in mice suggest that this pathway may also exert sex-specific effects. Effect sizes in the GWA studies were estimated at approximately 1.2 months earlier menarche per effect allele for LIN28B . A second menarche locus was identified in two of the four studies at 9q31.2. The biology behind the locus at 9q31.2 remains unknown, but its effect size is similar to the locus in/near LIN28B . The associated SNPs lie in an intergenic region with no obvious candidate genes nearby. The closest gene is TMEM38B , a transmembrane protein gene, which lies approximately 400 kb away from the signal at 9q31.2. Although these studies were groundbreaking, the LIN28B and 9q31.2 loci together explain only 0.6% of the variance in age at menarche.
Subsequent GWA studies have used larger and larger sample sizes, resulting in increases in statistical power and identification of many more genetic loci that influence pubertal timing.
The most recent GWA study on age at menarche studied over 350,000 women of European descent and identified 389 independent, genome-wide significant loci that influence pubertal timing. Collectively, these loci are estimated to account for 7.4% of the overall variation in timing of menarche. There was significant enrichment of expression of genes associated with these loci in the brain, consistent with the hypothesis that pubertal timing is mainly regulated by hypothalamic factors.
A GWA study has also been performed on age at voice-breaking in over 55,000 men and identified 11 genome-significant loci. Ten of these 11 loci have also been shown to be significantly associated with age at menarche, and the eleventh ( ANOS1 on the X chromosome, which is also associated with X-linked Kallmann syndrome, discussed further later) nearly reached genome-wide significance. Furthermore, analyzing all common variants across the genome, nearly all loci had similar effects on both age at voice breaking and age at menarche. Thus the mechanisms regulating pubertal timing in girls and boys appear to be largely, although not completely, overlapping.
GWA studies are designed to assess the contribution of common genetic variants to a particular phenotype. However, it is likely that other forms of inheritance also underlie constitutional delay of growth and puberty (CDGP), including rarer variants (frequency < 5% in the general population) with large or even small phenotypic effects; interactions (epistasis) between variants within a single gene or in multiple genes (oligogenicity); structural variation, such as copy number variants; and epigenetics. Indeed, some of these mechanisms have been identified as causes of hypothalamic amenorrhea.
Other Factors that Modulate the Timing of Puberty
Although these genetic advances are exciting, genetic factors alone cannot explain the reported secular changes in pubertal timing that have occurred since the late twentieth century. Clearly, changes in environmental factors must be involved as well, whether as independent regulators or through gene-environment (G X E) interactions. Variables, such as increased adiposity, insulin resistance, physical inactivity, psychological factors, changed dietary habits, and endocrine-disrupting chemicals have all been implicated as environmental factors that influence pubertal timing.
Effect of Body Mass Index on Pubertal Timing
Among white American children aged 6 to 11 years, rates of obesity increased from approximately 5% between 1963 and 1965 to 12% in 1999 to 2000. The possibility that the increasing rates of obesity contributed to the secular trend toward early puberty onset was originally highlighted in 1997. However, despite strong evidence in girls in support of a link between increased adiposity and early onset of pubertal markers, data in boys have remained somewhat ambiguous, with increased BMI being associated with earlier puberty in most studies, but with some studies showing obesity being associated with no change in pubertal timing or even with DP.
For example, in a study of 463 Danish choir boys, a significant downward trend in age at voice break was found over a 10-year period (from 14 years to 13.7 years). Age at voice break was significantly different between boys in the different prepubertal BMI quartiles, and a trend toward earlier voice break was associated with increasing BMI SDS. Boys in the heaviest quartile at 8 years of age had an increased likelihood of earlier voice break compared with those in the thinnest quartile, suggesting a relationship between prepubertal BMI and the timing of puberty in boys.
In the Copenhagen study discussed earlier, secular trends in pubertal onset over a 15-year period were assessed as were their relationship to BMI in boys. In a total of 1528 boys, onset of puberty, defined as age at attainment of a testicular volume greater than 3 mL, occurred 3 months earlier in 2006 to 2008 than in 1991 to 1993. BMI SD scores increased significantly from 1991 to 1993 to 2006 to 2008 as well. Interestingly, age of pubertal onset was no longer significantly different between study periods after adjustment for BMI indicating that the observed decline in age at onset of puberty was associated at least partly with an increase in BMI.
In a study from Jamaica, the effect body composition on the timing of onset of puberty were assessed in both boys and girls. Elevated fat mass at 8 years of age was associated with advanced puberty in both sexes. These data support the hypothesis that fast growth throughout childhood, especially fat-mass accretion, is associated with advanced pubertal development.
But not all studies agree. For example, in one study from Germany, body weight, height, peak height velocity, and pubertal stages were evaluated in 1421 peripubertal children. In contrast to the findings from studies earlier, the researchers found no significant differences in mean pubic hair stage in girls and boys with obesity when compared with either lean or normal-weight children. When analysis was restricted to children at pubic hair stage 2, age at this stage was found not to differ significantly between normal-weight and obese individuals. In boys, testicular volume at a given age was also similar across all weight groups. In the United States, a cohort of obese boys with DP has also been described.
In summary, research to date highlights inconsistencies in how obesity affects pubertal timing in boys. Recent data from a study in the United States may shed light on a reason for some of the inconsistency among the studies. Some 3872 boys were divided based on BMI into three categories (normal weight, overweight, and obese). Based on genital development, puberty among white and African American boys occurred earlier in the overweight compared with normal weight group but later in the obese compared with overweight category. This relationship was not observed among Hispanic youth. These data suggest that the relationship between earlier puberty and body weight may not be linear in boys and may differ by racial/ethnic population group.
Effect of Endocrine-Disrupting Chemicals
Some evidence supports associations between human pubertal timing and exposure to environmental modifiers, although much of the data relate to girls. For example, earlier menarche and pubarche have been associated with exposure to polybrominated biphenyls (PBBs) and dichlorodiphenyl dichloroethene (DDT), whereas delayed breast and pubic hair development, as well as delayed menarche have been associated with lead exposure. In addition, elevated serum levels of a mycotoxin (zearalenone) have been reported in girls with precocious puberty.
Some studies among boys have also been reported. Among the strongest studies is a longitudinal assessment of growth and pubertal timing among a cohort of around 500 boys in Russia. The results from this study have recently been summarized, with data indicating that prepubertal exposures to nondioxin-like polychlorinated biphenyls accelerate puberty, whereas levels of insecticides, dioxin-like compounds, organochlorine pesticides, and lead delay puberty. Other studies indicate that greater exposure to pesticides is associated with cryptorchidism or hypospadias.
The extent to which these are associations versus cause-and-effect relationships is not fully known, nor are underlying mechanisms. What effects these exposures have in the general population as opposed to isolated examples of associated abnormalities is also not clear. Certainly more research is this area is needed.
Precocious puberty
Traditionally, the onset of secondary sexual characteristics in a boy before age 9 years has been defined as precocious, whereas lack of testicular enlargement by age 14 years has been classified as DP. In clinical settings, boys present less often with precocious puberty than with DP, which is discussed more extensively later in the chapter. For clinical assessment, in addition to these dichotomous age cutoffs, a recent online tool allows for assessment of the stage of development (pubic hair, testicular volume, genital staging) relative to population norms. The progression of puberty can be monitored by using the puberty plots presented as stage line diagrams ( Fig. 18.6 ). These plots provide probability curves for an individual being at a certain developmental stage at certain age. The centile value on the y-axis is a quantitative assessment of pubertal timing for the individual being assessed on the plots. Such diagrams assist in identifying early and delayed development, as well as finding differences in the progression of puberty.
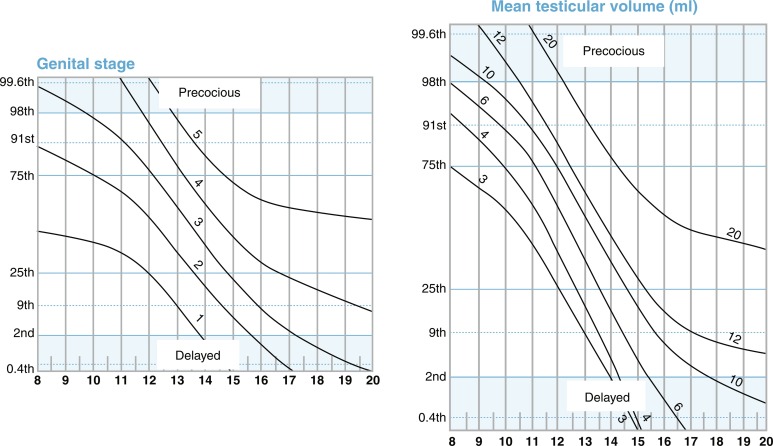
Gonadotropin-Releasing Hormone-Dependent Forms of Precocious Puberty
The most common form of precocious puberty is caused by the early activation of pulsatile GnRH secretion, termed CPP or gonadotropin-dependent precocious puberty . CPP may result from hypothalamic tumors or CNS lesions (neurogenic CPP) but in most cases remains unexplained (idiopathic CPP) ( Table 18.1 ).
Central (GnRH Dependent) | Peripheral (GnRH Independent) |
---|---|
Idiopathic | Congenital adrenal hyperplasia |
Central nervous system (CNS) masses | McCune-Albright syndrome |
| Testosterone producing tumors |
|
|
|
|
| hCG-producing tumors |
|
|
CNS infection |
|
Head trauma |
|
Iatrogenic |
|
|
|
|
|
| Exogenous exposure to androgen |
Malformations of CNS | Familial male limited precocious puberty |
| Hypothyroidism (Van Wyk–Grumbach syndrome) |
|
Hypothalamic hamartomas are an example of a cause of neurogenic CPP. The hamartomas are congenital malformations characterized by heterotropic gray matter, neurons, and glial cells, and they are generally located on the floor of the third ventricle or attached to the tuber cinereum. On magnetic resonance imaging (MRI), hamartomas exhibit an isodense fullness. Histologic examination has shown immunoreactivity for GnRH and for astroglial factors, such as TGF-α. Postulated mechanisms include GnRH secretion directly from these neurons, secretion of TGF-α that in turn stimulates GnRH secretion from GnRH neurons, and electrical activation of adjacent neural centers. Most hypothalamic hamartomas are sporadic, but they may occur in association with Pallister-Hall syndrome, which is caused by mutations in the GLI3 gene. Optic gliomas, which can be associated with neurofibromatosis 1, can also cause neurogenic GnRH-dependent precocious puberty. Other etiologies of neurogenic CPP include pineal tumors, suprasellar cysts, head trauma, CNS radiation, and static encephalopathy.
Loss-of-function mutations of the MKRN3 gene, located at chromosome 15q11-q13, have been associated with GnRH-dependent precocious puberty. MKRN3 encodes makorin RING-finger protein 3, which may function as an RNA-binding and/or a ubiquitin ligase. All clinically affected individuals inherited the mutated allele from their fathers, which can be explained because this gene is maternally imprinted and expressed only from the paternal allele. Both boys and girls are affected, although the degree of precocity appears to be greater in girls than boys. About 30% of familial cases and 3% of sporadic cases of CPP are associated with mutations in MKRN3 (OMIM ID 603856 ). The mutations in MKRN3 associated with CPP include frameshift mutations that are likely to cause loss of function; this suggests that MKRN3 normally acts to delay the onset of puberty and calls attention to the importance of inhibitory factors in the regulation of pubertal timing.
There is compelling evidence that mutations disrupting the DLK1 gene can also cause CPP. Deletions and imprinting defects in the region on chromosome 14 around DLK1 cause Temple syndrome, which is associated with precocious puberty. A smaller deletion that appears to affect DLK1 alone was identified in four individuals with CPP from the same family, suggesting that disruption of DLK1 is responsible for the precocious puberty seen in Temple syndrome. The association of DLK1 with precocious puberty was confirmed by a subsequent report of frameshift mutations in DLK1 in individuals with precocious puberty from three additional unrelated families. Like MKRN3 , DLK1 is imprinted, and CPP is observed only if the paternal allele is disrupted. DLK1 encodes a noncanonical ligand for the Notch family of receptors, and its precise function is unknown.
Gonadotropin-Releasing Hormone-Independent Forms of Precocity
Precocious development of secondary sexual characteristics may also be caused by mechanisms that do not involve activation of pulsatile GnRH secretion. These forms of precocity are called gonadotropin-independent or peripheral precocity and include gonadal and adrenal tumors, inappropriate activation of LH receptor because of gene mutations or signaling by tumors producing hCG, and exposure to exogenous sex steroids (see Table 18.1 ). Familial male-limited precocious puberty (FMPP, OMIM ID: 176410 ), formerly known as testotoxicosis , is a rare, dominant form of gonadotropin-independent precocity caused by constitutively activating mutations of the LH/hCG receptor ( LHCGR ). This disorder usually presents by age 1 to 4 years with rapid virilization, growth acceleration, skeletal (bone age) advancement, and elevated testosterone levels despite prepubertal levels of LH. McCune-Albright syndrome (MAS, OMIM ID: 174800 ) is another rare genetic cause of male sexual precocity. It is caused by postzygotic somatic activating mutations in GNAS1, the gene that encodes the α subunit of the heterotrimeric G s protein. G s transduces the signal from multiple G-protein–coupled receptors, including the LH receptor, and the constitutive activation of G S signaling leads to autonomous cell proliferation and scattered hyperfunction in endocrine organs, with a wide phenotypic spectrum that depends on which organs carry the mutation. The classical features of MAS include the clinical triad of bone fibrous dysplasia (BFD), café-au-lait skin spots, and precocious development of secondary sexual characteristics. In addition, hyperthyroidism caused by activation of the thyroid-stimulating hormone (TSH) receptor, hypercortisolism caused by constitutive activation of the CRH and/or adrenocorticotropin hormone (ACTH) receptor, kidney phosphate wasting, cholestasis, and hypertrophic heart disease can be present. For reasons that are not clear, MAS leads to sexual precocity more often in girls than in boys. Many boys may have unrecognized testicular pathology, but the predominant finding is Leydig cell hyperplasia, which may not warrant active intervention.
In 1960 Van Wyk and Grumbach first described a syndrome characterized in girls by breast development, uterine bleeding, and multicystic ovaries in the presence of long-standing primary hypothyroidism. A unique diagnostic feature of the Van Wyk–Grumbach syndrome is the combination of delayed bone age with apparent sexual precocity. Boys with this syndrome have macroorchidism without significant virilization. Testicular histology shows enlargement of the seminiferous tubules without an increase in Leydig cell number. If performed, GnRH stimulation shows a prepubertal response with suppressed LH, confirming GnRH-independent precocity. Most cases appear to originate from autoimmune thyroid disease, but there are some case reports where the syndrome is secondary to unrecognized congenital hypothyroidism. The pathophysiology of Van Wyk–Grumbach syndrome involves a complex mechanism, which is mediated at least in part by the direct action of TSH on FSH receptors. Recombinant human TSH (Rec-hTSH) elicits a dose-dependent cAMP response in cells expressing the human FSH receptor in vitro; however, the concentration of rec-hTSH required for half-maximal stimulation is several logs greater than that of hFSH. Early recognition and initiation of thyroid hormone replacement can lead not only to resolution of symptoms and improvement in adult height but also to avoidance of further diagnostic procedures.
GnRH-independent precocity can also be caused by the virilizing forms of congenital adrenal hyperplasia (CAH). These are autosomal recessive disorders of cortisol synthesis that present with premature pubic hair development, axillary hair development, accelerated linear growth, and phallic enlargement in the absence of testicular enlargement. The most common form is 21-hydroxylase deficiency because of loss-of-function mutations in CYP21A2 . Mutations in 11β-hydroxylase ( CYP11B1 ) and 3β-hydroxysteroid dehydrogenase type 2 ( HSD3B2 ) account for 5% to 10% of cases. Androgen-secreting tumors are another rare cause of GnRH-independent precocity in boys. Leydig cell tumors secrete testosterone. Because these tumors are usually unilateral, testicular volume may be asymmetric. The majority of Leydig cell tumors are benign. Ultrasound may be useful because the tumor may be too small to palpate. Mutations in the LHCGR gene have been identified in some testicular adenomas. Tumors secreting hCG also stimulate testosterone secretion in boys and can resemble familial male limited precocious puberty (testotoxicosis). These tumors are generally hepatic in origin (of note, activating mutations of the LH receptor and hCG-secreting tumors do not cause precocious puberty in girls, as estradiol production requires activation of both the FSH and LH receptors). Adrenal tumors in children may secrete androgens, and virilization is a common presentation.
Whether precocious puberty is gonadotropin dependent or gonadotropin independent not always clear. Both forms can sometimes be present because peripheral forms of sexual precocity, such as CAH or testicular or adrenal tumors, can eventually lead to superimposed central precocious puberty through mechanisms that are unknown. In contrast, in some cases of precocious pubertal development among girls, pubertal manifestations will stop progressing or regress, making treatment unnecessary; such cases are not as commonly seen in boys. The mechanisms responsible for these nonprogressive forms of precocious puberty are not known, but there is evidence that in some cases the HPG axis is intermittently but not fully activated.
Premature Adrenarche
Another form of early development of secondary sexual characteristics occurs when the zona reticularis of the adrenal cortex matures early, causing premature adrenarche. Premature adrenarche, more common among girls, is not associated with progressive pubertal development and is manifested by pubic and axillary hair with modest elevation of DHEA-S. Usually, there is no substantial advancement in bone age; however, even in the subset with more advanced bone ages there appears to be little to no compromise of adult height, although admittedly, most of these data derive from studies among girls. As a result premature adrenarche does not require treatment.
Consequences of Precocious Puberty
In cases of progressive central precocious puberty or peripheral precocity, the concerns include adverse psychosocial outcomes and short adult stature because of early epiphyseal fusion. Several studies have assessed adult height in subjects with a history of precocious puberty, demonstrating that loss in adult height can be substantial. Height loss is inversely correlated with the age at the onset of puberty. It is especially important to assess carefully the growth rate and skeletal maturation of individuals with central precocious puberty because of CNS lesions (e.g., tumors, cerebral malformations or injury from trauma or radiation) because these lesions may be associated with concomitant GH deficiency, which can be masked by sex steroid–driven growth that may deceptively appear adequate. In such cases, the combination of undiagnosed and untreated GH deficiency and rapid skeletal maturation driven by sex steroids may result in severely compromised adult height.
Although available data derive mostly from study of girls with pubertal onset at the earlier end of the normal range, it has been suggested that a higher proportion of early-maturing adolescents within the general population engage in exploratory risky behaviors (sexual intercourse and substance use) at an earlier age than adolescents with average or late timing of maturation. However, available data regarding potential adverse psychosocial outcomes specific to patients with precocious puberty are limited, and it is not clear that data obtained from individuals with maturation at the early end of the normal spectrum are fully applicable to precocious puberty.
Epidemiological studies have associated earlier pubertal timing with increased risk for a number of negative adult health outcomes, including obesity; reproductive cancers, such as breast cancer in women and prostate cancer in men; type 2 diabetes; and cardiovascular disease. Most of these studies were conducted in women because recalled age at menarche is a fairly reliable indicator of pubertal timing, and a comparable marker is lacking in men, although recalled age at voice breaking has been used in some studies. Also most of these studies examined pubertal timing more generally rather than precocious puberty specifically, but many of the observed associations extended into the precocious range.
Early association studies were unable to establish causality, that is, whether DP causes the increased risk directly or whether some common upstream factor affects pubertal timing and adult-disease risk independently. More recently, human genetic approaches have been used to address this issue. One approach is to compare the effects of common genetic variants on two traits to determine whether the traits share a common genetic basis; this approach has been used to demonstrate that pubertal timing and BMI have shared genetic determinants. Another technique called Mendelian randomization assesses whether (after adjustment for pleiotropy) the genetic determinants of one trait also influence a second trait, with the logical inference that any such influence is mediated by the first trait influencing the second trait. This technique has been used to suggest that it is pubertal timing itself that influences risk of reproductive cancers, including breast, ovarian, and endometrial cancers in women and prostate cancer in men. Mendelian randomization also indicates that earlier pubertal timing leads to higher blood pressure in adulthood. With regard to BMI, pubertal timing has been shown to influence adult BMI, and childhood obesity has been shown to affect pubertal timing ; thus more than one mechanism underlies the relationship between pubertal timing and BMI.
Pathologic entities leading to sexual precocity warrant treatment, but whether and at what ages one should initiate treatment for idiopathic central precocious puberty is less clear, particularly if adult height does not appear to be compromised. Currently, robust data regarding the short-term and long-term psychosocial sequelae of CPP and data regarding whether treating CPP with GnRH analogues (GnRHa) alter these outcomes are lacking. Thus one needs to be cautious when using psychosocial outcomes as a rationale for pharmacologic intervention to halt pubertal progression, especially among boys with the onset of puberty close to the normal range.
Evaluation of the Boy With Precocious Development of Secondary Sexual Characteristics
Boys with sexual precocity require careful evaluation because many have underlying disorders. Those with premature pubarche (early development of pubic hair or body odor) need to be assessed for peripheral causes of precocity before determining that the premature pubarche stems simply from premature adrenarche. Premature adrenarche may be distinguished from true precocity over time by lack of rapid progression. Conversely, progressive precocity is marked by significant bone age advancement (> 2 SD for age), growth acceleration, and rapid progression of secondary sexual characteristics on physical examination.
The evaluation of a boy with sexual precocity is outlined in Box 18.1 . Considerations include first verifying that the pubertal development is occurring outside the range of normal development before initiating an evaluation. It is also important to note that not all tests are appropriate in each case and that the diagnostic yields of various tests are not known. Hence it is important to allow history and physical examination to guide the evaluation. The child with both testicles enlarged to 8 mL or more, for example, is most likely to have central precocious puberty, with FSH having led to the expansion of seminiferous tubule volume (whereas cases of testotoxicosis, and hCG-secreting tumors can also present with modest bilateral testicular enlargement, volumes in these conditions are usually no more than 6 mL). Conversely, the child with bilateral prepubertal-sized testicles is more likely to have peripheral precocity; the child with unilateral testicular enlargement may have a testicular tumor. Testing should be directed accordingly.
Common initial screening tests
- •
Careful history, physical examination, and assessment of growth velocity
- •
Bone age
- •
LH, FSH
- •
Testosterone (and in some cases estradiol)
- •
DHEA-S
- •
17-hydroxyprogesterone (to screen for congenital adrenal hyperplasia)
- •
TSH, T 4
Secondary tests to consider
- •
MRI of pituitary and brain (to screen for a central nervous system lesion)
- •
GnRH agonist stimulation test (can distinguish central precocious puberty from peripheral precocity)
- •
ACTH stimulation test (to diagnose congenital adrenal hyperplasia)
ACTH , Adrenocorticotropin hormone; DHEA-S , dehydroepiandrosterone sulfate; FSH , follicle-stimulating hormone; GnRH , gonadotropin-releasing hormone; LH , luteinizing hormone; MRI , magnetic resonance imaging; T 4 , thyroxine; TSH , thyroid-stimulating hormone.
Peripheral causes of sexual precocity are characterized by suppressed LH and FSH values in the setting of elevated sex steroid levels. Testosterone levels will be elevated in instances of isosexual precocity (secondary sexual characteristics consistent with male sex), whereas estradiol levels may be elevated in the rare instances of contrasexual precocity (secondary sexual characteristics inconsistent with sex, such as marked breast development as the presenting sign in a boy). Determining the underlying mechanism for progressive peripheral precocity is important because all instances will result from pathologic conditions or exogenous exposures. DHEA-S is often used as a screen for adrenal tumors or adrenal pathology. Determination of the 17-hydroxyprogesterone concentration is used to screen for congenital adrenal hyperplasia because of 21-hydroxylase deficiency. Congenital adrenal hyperplasia and hormone-secreting tumors of the adrenal gland are discussed in detail in Chapter 14 . Evaluation of skeletal maturation with a bone age is an important adjunct, as it allows for prediction of adult height.
Historically, the gold standard for the diagnosis of central precocious puberty was GnRH stimulation testing and the demonstration of pubertal gonadotropin responses that derive from the gonadotropes having been primed by endogenous GnRH secretion. However, with the advent of ultrasensitive gonadotropin assays, the recognition that these assays can identify individuals with CPP using unstimulated random samples, and the unavailability of GnRH for testing has led many to forego stimulation testing. GnRH agonists can be used as an alternative to GnRH for stimulation testing, but again the diagnosis can often be made through the combination of clinical features and a baseline LH value in the pubertal range. Although determining the diagnostic cutoff for the basal LH level is difficult because of a lack of normative data and variability among assays, a value of 0.3 IU/L or more, using an ultrasensitive assay with a detection limit near 0.1 IU/L, is commonly cited. If stimulation testing is done, a diagnostic cutoff of 5 IU/L for peak value has been suggested. LH values are more useful than FSH values in the diagnostic evaluation of precocious puberty, but stimulated LH/FSH values can help to identify patients with slowly progressive precocious puberty because these children tend to have FSH-predominant responses. Idiopathic central precocious puberty is a more common cause of precocious puberty among girls than among boys in endocrinology clinics. Hence all boys with CPP should have brain MRI to exclude underlying pathology.
In summary, it is important for physicians evaluating patients with suspected precocious puberty to address these questions: Is pubertal development occurring outside the normal range of development? What is the underlying mechanism, and is that mechanism associated with a risk of a serious condition, such as an intracranial lesion? Is the pubertal development likely to progress, and could this impair the child’s normal physical and psychosocial development?
Treatment of the Child With Precocious Puberty
Unless there is underlying pathology that requires intervention, whether the precocious pubertal development is likely to impair the boy’s normal physical or psychosocial development is often the feature that determines if treatment is needed.
Central Precocious Puberty
Because nonprogressive forms of precocious puberty do not require intervention, a prerequisite for the consideration of therapy for CPP is the presence of documented, progressive pubertal development over a 3- to 6-month period, although this period of observation may not be necessary if the child is at or beyond Tanner stage 3 genital development. Epiphyseal fusion is an estrogen-dependent process, and early and progressive production of sex steroids can cause rapid advancement of skeletal maturation and result in compromised adult height. Thus preservation of adult stature is one of the main reasons to consider treatment of CPP with GnRH agonists, which downregulate the pituitary-gonadal axis and limit pubertal progression. Although the data derive primarily from studies among girls, it appears that the risk of short stature is most pronounced in those children with earlier onset of symptoms. An advanced bone age also contributes to poorer height outcomes. Available data suggest that the greatest height gain (preservation) occurs with treatment of girls with onset of puberty before 6 years of age, with more modest benefit being seen with onset between 6 and 8 years. However, insufficient data exist to draw similar conclusions regarding age of onset and height outcomes among boys ; consequently, a consensus conference recommended considering initiation of GnRHa therapy for all boys with onset of CPP before age 9 years who have compromised height potential.
The second main reason to consider therapy in CPP is to mitigate potential psychosocial effects of precocious puberty. However, as noted earlier, additional studies are needed to determine the effects of precocious puberty on quality of life and psychosocial functioning and to evaluate whether treatment with GnRHa therapies affects these outcomes. Thus the consensus conference concluded that the use of GnRHa therapies solely to influence psychosocial consequences of precocious puberty needs to be considered carefully, given the absence of convincing data. These cautions are even more pertinent for boys because data regarding males are even more scarce.
Finally, although the associations with pubertal timing and later life health outcomes mentioned earlier are intriguing, there are no data regarding whether treatment of precocious (or delayed) puberty affects the risk of such outcomes. This question represents an important area of further study because the ability to mitigate risks for cancer or cardiovascular disease would be an important consideration when deciding for or against treatment of precocious puberty.
Various formulations (intramuscular, subcutaneous, and intranasal) of short- (daily) and long-acting GnRH agonists are available to treat CPP. The depot formulations are preferred, as they are easier for patients and families to administer regularly over a long treatment period. The initial depot medications were 1-month formulations, which have been shown to be well tolerated and effective. Subsequently, 3-month and 6-month depot formulations were developed, and a 1-year implant (histrelin-acetate) has also become available. Which GnRHa to use depends on patient and provider preference, local regulatory approvals, and reimbursement systems.
GnRH agonists are generally well tolerated. Occasionally, an initial, temporary flare of GnRH activity can occur and result in transient advancement of secondary sexual characteristics. Local reactions (sterile abscesses) can occur in 10% to 15% of patients using injected depot formulations of GnRH analogs. Other considerations include long-term effects of GnRH agonists on bone mineral density (BMD) and reproductive function. These outcomes have largely been studied among girls, and the available data are reassuring. Whether GnRHa use is associated with increased obesity is less clear, and data among boys with CPP are sparse. However, most studies in girls are reassuring and indicate that this concern is not great enough to argue against use of GnRHa in cases of CPP, especially because risk factors for obesity may be associated with CPP itself and not necessarily the use of GnRHa.
Monitoring of GnRH agonist therapy should consist of assessment of Tanner stage and growth velocity every 3 to 6 months, with assessment of skeletal maturation every 6 to 12 months. Progression of pubertal development or of rapid skeletal maturation indicates a lack of efficacy, poor adherence, or a misdiagnosis. Such patients warrant reevaluation, including potential measurement of baseline or stimulated LH levels Whether it is helpful to routinely measure gonadotropins to monitor suppression during GnRHa treatment is controversial. When to discontinue GnRH agonist therapy must be evaluated for each individual patient. Data among boys are limited, but examination of clinical characteristics at the discontinuation of therapy—such as treatment duration, height, growth velocity, bone age, and chronologic age—has failed to identify clear predictors of adult height that might guide decisions around when to discontinue GnRH agonist therapy. In the absence of this data, it seems reasonable to time discontinuation based on patient and family preference, typically with the intent of having pubertal development resume at an age that allows for development to occur in parallel with the patient’s peers.
Peripheral Precocity
In the past, treatment of GnRH-independent causes of sexual precocity, such as MAS or familial male-limited precocious puberty, included using inhibitors of steroidogenesis (ketoconazole), weak antiandrogen agents (spironolactone), and first-generation aromatase inhibitors (AIs) (testolactone). Although these therapies were partially effective in slowing growth velocity and reducing virilization, the risk of hepatotoxicity and adrenal insufficiency with ketoconazole, as well as the requirement of multiple daily dosing, were obstacles to achieving a favorable therapeutic outcome. More recently, short-term combination therapy with a potent antiandrogen agent, bicalutamide, and third-generation AIs, anastrozole and letrozole, appears to be effective in reducing growth rate and virilization and improving predicted adult height. This combination therapy provides a more convenient once-daily dosing regimen, although the therapy is more expensive than previous treatments and can be associated with gynecomastia. Full endorsement of these regimens awaits further controlled clinical trials, including the evaluation of the long-term effects of such therapy on adult height, fertility, metabolic parameters, cognitive functions, and bone health. If superimposed CPP develops in a patient with peripheral precocity, treatment with a GnRH agonist may be warranted.
Delayed puberty
The etiologies, evaluation, and treatment of DP have previously been reviewed by the authors. DP is defined as the absence of testicular enlargement 2 to 2.5 SDs later than the population mean (traditionally age 14 years in boys). As noted previously, because of the downward trend in pubertal timing in some but not all reports from the United States, and other countries, some advocate for younger age cutoffs for the general population or perhaps for particular countries or ethnic groups. However, the secular change in the onset of puberty has not been as notable in late developing boys and hence the need to readjust age definitions for DP in males may not be necessary. Of note, because pubarche may result from maturation of the adrenal glands (adrenarche) independent of HPG axis activation, the presence of adrenarchal signs (such as pubic hair) does not exclude a diagnosis of DP.
DP is often quite concerning to patients and families. It can affect psychosocial well-being, peer relationships, and sports participation, and these issues are common reasons for initiating therapy. However, as with precocious puberty, further studies are needed to assess fully the psychosocial distress experienced by individuals with DP, whether this distress has long-term sequelae, and what impact sex-steroid supplementation has on these outcomes. Patients, families, and practitioners are also often worried that DP may affect adult stature, and many patients present with relative familial short stature, along with DP and lack of a pubertal growth spurt, which accentuates concerns about both current and adult stature. Adult height can indeed be affected by DP, but on average it is only slightly below the genetic target. It remains unclear whether adult bone mass is adversely affected by pubertal delay and whether concerns related to bone health represent a medical reason to initiate therapy. Finally, as with early puberty, older age at onset of puberty has been associated with later life outcomes, but it is not known if treatment of DP affects these associations.
Etiologies of Delayed Puberty
The most common cause of DP in boys is self-limited DP or CDGP, which refers to an extreme of the normal spectrum of pubertal timing. In two large series, approximately between 65% to 80% of boys and 30% to 50% of girls with DP had self-limited DP ( Fig. 18.7 ). However, because the data derive from referral centers, these percentages may underestimate the frequency of self-limited DP encountered by primary care providers.
