Primary Intracranial Neoplasms
FIGURE 35.1. A: Coronal section through the telencephalon at the plane of the frontal horn of the lateral ventricle. B: Transverse section at the level of the floor of the central part of the lateral ventricle. View of the superior surface of the plane of sectioning. C: Section through telencephalon and brainstem parallel with the cerebral peduncles. View of the posterior surface of the plane of sectioning. On the right side of the figure, the section reaches back to approximately the middle of the cerebral peduncle (oblique section). I to III indicate thalamic nuclei: I, medial nucleus; II, anterior nucleus; III, lateral nucleus. (A to C, from Sobotta J, Figge FHJ. Atlas of human anatomy, Vol. 2, 9th ed. Munich, Germany: Urban & Schwarzenberg, 1977. © Urban & Schwarzenberg, with permission.)
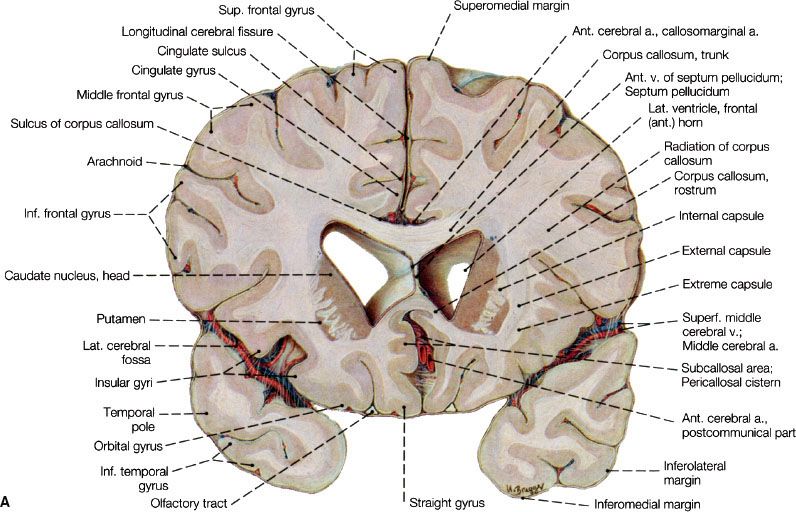
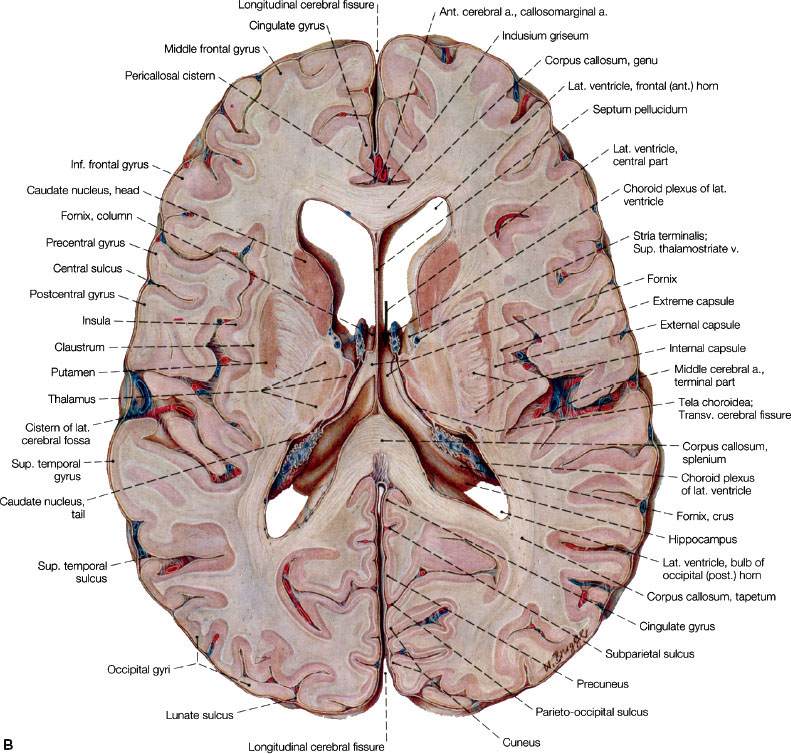
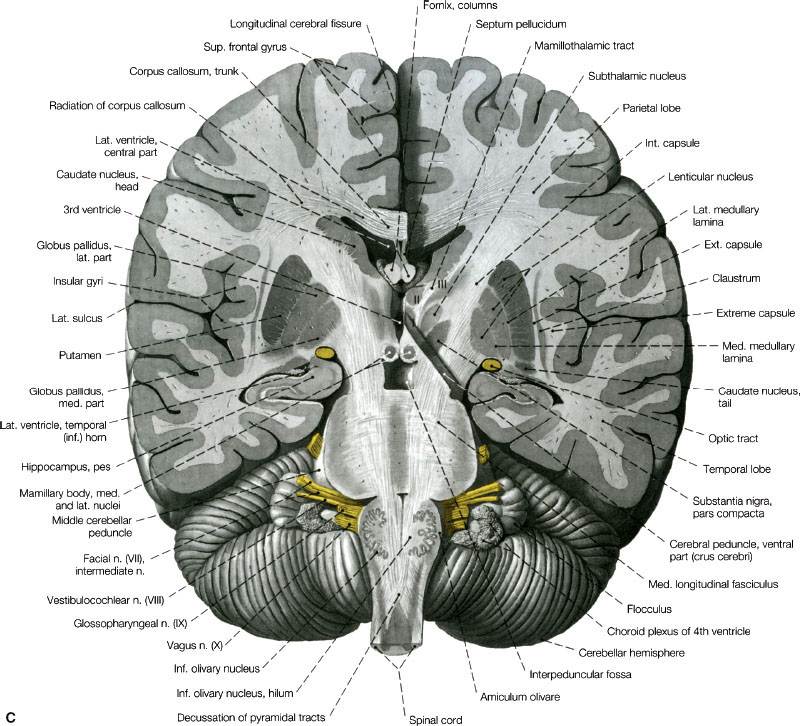
ANATOMY
The central nervous system (CNS) is enveloped by three meningeal layers: the dura mater (also known as the pachymeninges), the arachnoid mater, and the pia mater. The pia and arachnoid layers are also referred to as the leptomeninges, and within them is the subarachnoid space, which is filled with cerebrospinal fluid (CSF). Dural folds separate the two hemispheres of the cerebrum (falx cerebri) and the cerebrum from the cerebellum and brainstem (tentorium or falx cerebelli). The frontal and parietal lobes are separated by a well-defined sulcus (“central sulcus”). The frontal and temporal lobes are separated by the Sylvian fissure, and the parietal and occipital lobes are separated by the calcarine sulcus (Fig. 35.1).
The diencephalon consists of the thalamus and the pineal region and is situated between the cerebrum and the mesencephalon, adjacent to the third ventricle. Lateral to the thalamus is the internal capsule, which carries the motor fibers (upper motor neurons) from the cortex en route to the brainstem and spinal cord.
At the tentorial notch, the mesencephalon rides on the upper part of the clivus. Its interior, the tectum, is partially occupied by cranial nerve nuclei (for the oculomotor, trochlear, and proprioceptive portions of the trigeminal nerves). The dorsal plate houses the superior and inferior colliculi, which regulate eye movements and hearing impulses, respectively. The trochlear nerve is the only cranial nerve that exits from this dorsal location.
The pons relays information between the two cerebellar hemispheres and from the spinal cord to the cerebellum, carries the major ascending and descending pathways between the mesencephalon and the medulla oblongata, and contains the major motor and tactile sensory nuclei for the trigeminal nerve, which emerges from its lateral surface. The border between the pons and the medulla oblongata is noteworthy for the emergence of the abducens, facial, and vestibulocochlear (acoustic) cranial nerves.
The cerebellum develops laterally and posteriorly from the pons and differentiates into the median vermis cerebelli and the bilateral hemispheres, which are flattened by the sloping tentorium on both sides. Anteriorly, the cerebellum faces the dorsal aspects of the pons and the medulla oblongata (the floor of the fourth ventricle).
The medulla oblongata forms the link between the pons, the spinal cord, and the cerebellum. It houses the majority of the cranial nerve nuclei (abducens, facial, vestibulocochlear, glossopharyngeal, vagal, accessory, and hypoglossal).
CSF is produced by the choroid plexus, which lies in the roofs of the fourth and third ventricles, as well as in the medial walls of the central body and inferior horns of the lateral ventricles. The foramina of Munro transmit CSF between the third and lateral ventricles at the superolateral corners of the third ventricle. The aqueduct of Sylvius in the midbrain transmits CSF from the third to the fourth ventricles. It is the narrowest canal of the intracranial nervous system and is therefore the most common location of obstruction of flow by compression or tumor deposits, resulting in noncommunicating (obstructive) hydrocephalus. CSF in the fourth ventricle flows out of the ventricular system through the midline foramen of Magendie and the two lateral foramina of Luschka to the subarachnoid space.
CSF resorption back into the venous system occurs at arachnoid (or Pacconian) granulations—special outpouching structures from the arachnoid membrane that enhance fluid movement from the CSF space into the venous sinus system. Scarring from infection or inflammation or clogging of the arachnoid granulations causes increased pressure in the CSF space and communication hydrocephalus.
EPIDEMIOLOGY
In 2010, there were an estimated 22,020 new cases of primary CNS tumors in the United States and 13,140 deaths,1 for an incidence of approximately 6.5 per 100,000 persons. The incidence of brain tumors increases with age to reach 50 per 100,000 at age >75 years.1
Occupational and environmental exposures have been associated with the development of CNS tumors. Farmers and petrochemical workers have been shown to have a higher incidence of primary brain tumors. A variety of chemical exposures have been linked, as reviewed by Ohgaki and Kleihues.2 The use of cellular phones has been questioned as a contributing factor to the development of brain tumors. The World Health Organization (WHO) recently classified radiofrequency electromagnetic fields, such as those emitted by wireless phones, as “possibly carcinogenic to humans” (group 2B) based on limited clinical evidence.3 Although prior cohort, case–control, and time–trend analyses showed no association between cell phone use and brain tumor risk,4–6 more recent case–control studies suggested a potential increased risk of glioma among individuals with the greatest cumulative lifetime cell phone use (≥ more hours in one study, >2,000 hours in another).7,8 However, significant concerns with respect to recall and selection biases in both studies prevent a causal association from being concluded.
Prior exposure to ionizing radiation is a known risk factor for development of primary CNS tumors, particularly meningiomas, but also gliomas, sarcomas, and other tumor types.9 There is a 2.3% incidence of primary brain tumors in children treated with prophylactic cranial irradiation for acute leukemia, a 22-fold increase over expected.10,11
Development of intracranial malignancy is also associated with several hereditary diseases, such as neurofibromatosis types 1 and 2, von Hippel-Lindau disease, and tuberous sclerosis. Other hereditary associations are with retinoblastoma and Li-Fraumeni syndrome.
NATURAL HISTORY
The natural history of a primary brain neoplasm is determined by its histology, grade, and location. The majority of adult gliomas spread invasively without forming a natural capsule. They frequently cause edema in surrounding tissue. This edema is usually vasogenic but may be ischemic or cytotoxic. It is seen best on T2-weighted magnetic resonance imaging (MRI) and is responsible for at least some of the clinical symptoms and signs. The edema is a consequence of altered blood–brain barrier (BBB) permeability. Different tumors cause varying amounts of edema (in descending order: metastases, astrocytomas, meningiomas, and oligodendrogliomas).
Some high-grade neoplasms metastasize by “seeding” the subarachnoid and ventricular spaces. Because of gravity or flow, these metastatic deposits are often present in the caudal portion of the spinal canal. Tumors that have a propensity for CSF spread include medulloblastomas, primitive neuroectodermal tumors (PNETs), and CNS lymphoma. The exact frequency of CSF spread among other histologies (e.g., germ cell tumors, ependymomas) is debated in the literature. Extracranial metastases from primary brain tumors are rare but can occur with medulloblastomas, germinomas, and high-grade astrocytomas.
TABLE 35.1 SYMPTOMS, SIGNS, AND DIAGNOSTIC CHARACTERISTICS OF VARIOUS INTRACRANIAL TUMORS
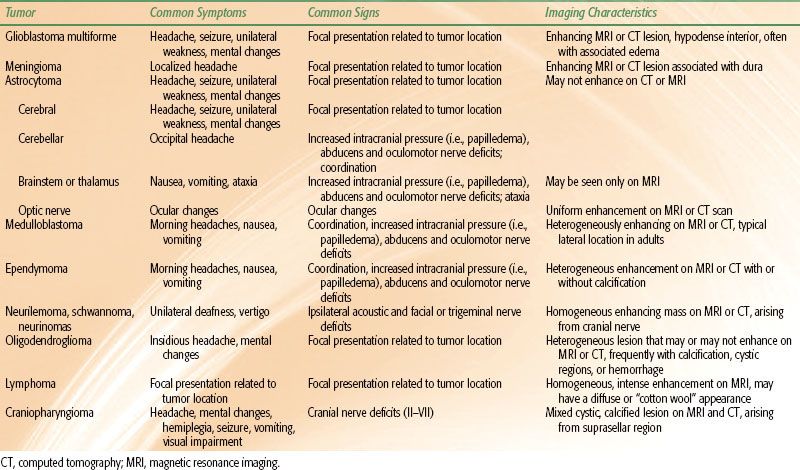
CLINICAL PRESENTATION
The presenting symptoms of a primary brain tumor are classified as generalized or focal. Headache is more prevalent in patients with fast-growing, high-grade tumors. Seizures are a more common presenting feature in low-grade tumors. Focal neurologic deficits such as weakness, language dysfunction, or sensory loss are more frequent presentations of high-grade tumors. Acute events such as hemorrhage markedly alter the tempo of symptom onset regardless of tumor grade. Table 35.1 summarizes common clinical presentations of the more common CNS tumors.
Because brain parenchyma is anesthetic, headaches associated with brain tumors are due to increased intracranial pressure or to local pressure on sensitive intracranial structures (mainly dura and vessels). Headaches associated with increased intracranial pressure classically occur in the morning. Associated findings may include focal neurologic deficits, behavioral changes, and papilledema. Cushing’s triad is classically associated with increased intracranial pressure, but the full triad (hypertension, bradycardia, respiratory irregularity) is seen in only one-third of the cases of increased intracranial pressure. Long-standing increases in intracranial pressure may lead to optic atrophy and blindness because of transmission of the pressure to the optic nerves.
DIAGNOSTIC WORKUP
The initial workup of patients with brain tumors must include a complete history and physical examination. Information obtained from relatives and friends is helpful because many tumors cause changes in mentation not appreciated by the patient. In patients with symptoms, signs, or imaging suggestive of systemic dissemination, biopsy confirmation of the primary tumor or at least one of the extracranial metastatic sites is recommended. Solitary brain lesions in adult patients with certain types of known systemic cancer (e.g., lung, breast, or colon cancers or melanoma) are far more likely to be a cerebral metastasis than a primary CNS tumor, although this is not always the case.
Imaging Studies
MRI with a gadolinium-containing contrast agent is the imaging modality of choice for most CNS tumors. Computed tomography (CT) is generally reserved for those situations in which MRI is contraindicated, such as implanted pacemaker, metal fragment, or paramagnetic surgical clips, or where there is a need to image the extent of calcification or hemorrhage.
FIGURE 35.2. Magnetic resonance image of brain showing (A) glioblastoma, demonstrating a contrast-enhancing lesion with central necrosis and vasogenic edema, and (B) low-grade glioma, illustrating a nonenhancing lesion difficult to delineate from normal parenchyma.
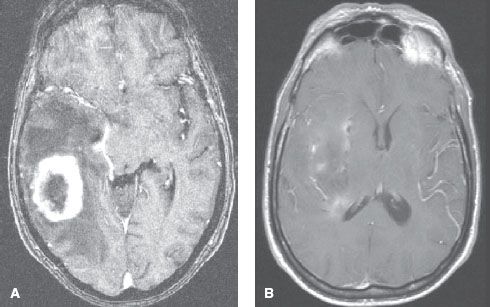
FIGURE 35.3. Oligodendroglioma imaged using an anatomic imaging technique, contrast-enhanced three-dimensional, spoiled gradient echo, T1-weighted magnetic resonance imaging (top) and a functional imaging technique, fluorodeoxyglucose positron emission tomography (bottom). The red contour delineates the extent of increased metabolic activity seen using functional imaging. Looking at the top panel, one can appreciate the fact that if the anatomic image set alone were to be used for target definition, it would yield a gross underestimation of the target volume as compared with the functional imaging technique.
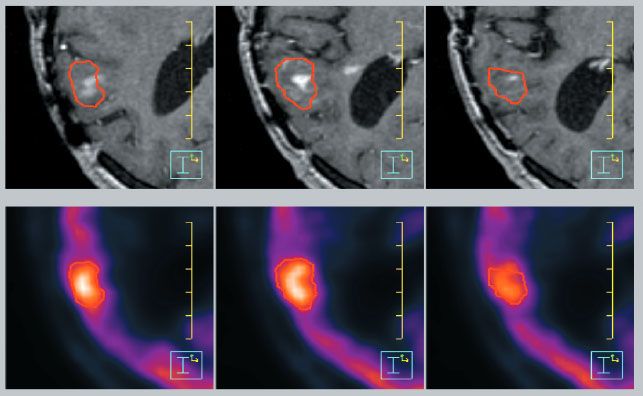
Magnetic Resonance Imaging
The most useful imaging studies are T1-weighted sagittal images, gadolinium (Gd)-enhanced (usually obtained in high-resolution modes such as spoiled gradient echo or magnetization-prepared rapid gradient echo) and unenhanced T1 axial images, T2-weighted axial images, and fluid-attenuated inversion recovery (FLAIR) sequences. As is the case with CT contrast agents, gadolinium-based contrast leaks into parenchyma in areas with BBB breakdown, and the paramagnetic properties of gadolinium generate hyperintense signal on T1 scans. T1 images usually are better at demonstrating anatomy and areas of contrast enhancement. T2 and FLAIR images are more sensitive for detecting edema and infiltrative tumor. Tumor appearance on T1-weighted MRI is similar to that on CT, although tumor volumes are better delineated on MRI, particularly with low-grade neoplasms that do not demonstrate contrast enhancement (Fig. 35.2). With the increasing incidence of posttreatment “pseudoprogression,” additional specialized diffusion, perfusion, and spectroscopic sequences are being increasingly used to distinguish tumor from necrosis or pseudoprogression, and positron emission tomography (PET) imaging may also have some role in this; in the United States, only fluorodeoxyglucose-PET is approved, but amino acid, fluorothymidine, and F-DOPA PET imaging is being evaluated (Fig. 35.3). Diffusion-weighted and functional MR also has utility in guiding resection, and in this context, magnetoencephalography is also being studied.
Neuraxis Imaging
For neoplasms with high risk of CSF spread, staging of the neuraxis is essential. Gd-enhanced MRI of the spine is the imaging modality of choice. Ideally, neuraxis imaging should be performed before surgery. In the immediate postoperative period, spinal MRI scans may be difficult to interpret because arachnoiditis and blood products in the CSF can mimic leptomeningeal metastasis. Delayed spinal MRI (>3 weeks after surgery), combined with an increased dose of intravenous gadolinium, is a sensitive imaging study to detect leptomeningeal disease.
Histologic Confirmation of Diagnosis
The morbidity of biopsy has decreased significantly with improvements in operative technique and anesthesia, as well as with the availability of stereotactic biopsy techniques. Exception might be made in selected patients, such as those patients with known active systemic cancer and multiple lesions that are radiographically consistent with brain metastases, patients with typical clinical and MRI findings of a brainstem glioma or optic nerve meningioma, HIV-positive patients with CT or MRI findings consistent with primary CNS lymphoma and positive Epstein-Barr virus polymerase chain reaction in the CSF, or patients with secretory germ-cell tumors.
Cerebrospinal Fluid Cytology
CSF cytology is essential for staging tumors with a propensity for CSF spread (e.g., medulloblastoma, PNET, germ cell tumors, CNS lymphoma). Sampling of the CSF in the immediate postoperative period may lead to false-positive results, however, and is best done before surgery or more than 3 weeks after surgery, as long as intracranial pressure is not elevated. CSF spread of tumor may be associated with several abnormal CSF findings. These include CSF pressure >150 mm H2O at the lumbar level in a laterally positioned patient, elevated protein level (>40 mg/dL in the lumbar cistern), a reduced glucose level (<50 mg/mL), and the finding of tumor cells by cytologic examination. Tumor markers in the CSF may help in making the diagnosis.
Differential Diagnosis
Most adults with new or persistent neurologic findings (focal deficit, increased intracranial pressure, seizures, altered mentation) are investigated using CT or MRI. There are a number of classical imaging features that help to refine the differential diagnosis (Table 35.2).
TABLE 35.2 DIFFERENTIAL DIAGNOSIS OF SPACE-OCCUPYING LESIONS ON COMPUTED TOMOGRAPHY OR MAGNETIC RESONANCE IMAGING
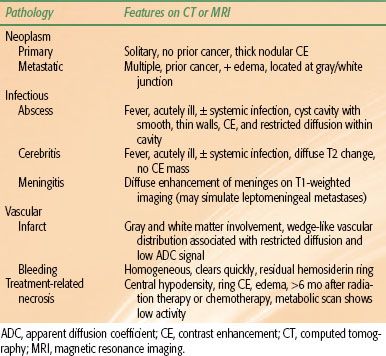
TABLE 35.3 HISTOLOGIC CLASSIFICATION OF TUMORS OF THE CENTRAL NERVOUS SYSTEM
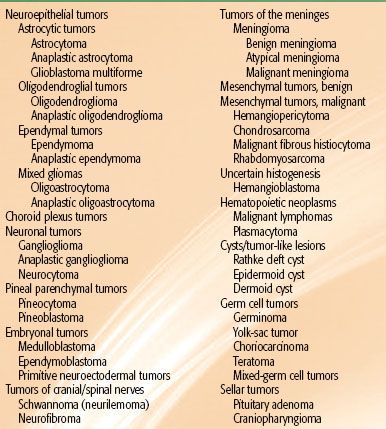
PATHOLOGY
Primary intracranial tumors are of ectodermal and mesodermal origin and arise from the brain, cranial nerves, meninges, pituitary, pineal, and vascular elements. The WHO classification system is the mostly widely used and lists approximately 100 distinct pathologic subtypes of CNS malignancies in broad categories (Table 35.3).12 Guidelines for assigning grade of malignancy are provided, where applicable.
GENERAL MANAGEMENT
The medical management of patients with brain tumors includes management of increased intracranial pressure, seizures, and venous thromboembolic disease.
Cerebral Edema
Glucocorticoids are used to control neurologic signs and symptoms caused by cerebral edema. Lower doses of steroids (e.g., 2 to 4 mg dexamethasone) twice daily have been shown to be as effective as higher doses. Prolonged steroid use is associated with multiple medical problems, and therefore steroids should be discontinued or tapered to the lowest dose necessary, as soon as possible. Dexamethasone is the most common corticosteroid used for historical reasons and because of minimal mineral-corticoid effects. As with all corticosteroids, a slow taper is necessary to prevent a rebound in cerebral edema and also to allow the pituitary–adrenal axis to recover.
Seizures
Patients with seizures require anticonvulsants. Because anticonvulsants such as carbamazepine, phenobarbital, and phenytoin induce hepatic cytochrome P450 isozymes, which increase the metabolism and clearance of several cancer chemotherapy agents such as paclitaxel and irinotecan,13,14 non–enzyme-inducing anticonvulsants, such as levetiracetam, lacosamide, lamotrigine, and pregabalin are preferred.
Prophylactic anticonvulsant use (in patients who have never experienced a seizure) remains controversial, although practice guidelines from the American Academy of Neurology recommended against their use because of lack of data.15
SURGERY
Surgical procedures can be summarized as biopsy for diagnosis only, resection for cure, surgical debulking for management of mass effect–related symptoms, CSF diversion procedures to relieve acute symptoms caused by increased intracranial pressure or hydrocephalus, and, increasingly, re-resection to distinguish and manage the effects of progressive tumor from symptomatic necrosis or pseudoprogression. Other roles of surgery include the placement of chemotherapy wafers, brachytherapy devices, and catheters for interstitial drug delivery and for monitoring tumor drug concentrations. Complete resection of tumor is associated with a survival advantage for some tumor types.16,17 However, for some radiosensitive and/or chemosensitive malignancies such as primary CNS lymphoma, aggressive resection is unnecessary, and the surgeon’s role is limited to providing diagnostic material.
Operative Technique
Ultrasound-, CT-, and MRI-guidance systems provide surgeons with intraoperative navigation based upon preoperative and/or intraoperative data. In general, these consist of a workstation into which the relevant imaging studies have been loaded, together with infrared or ultrasound detectors that recognize the three-dimensional (3D) orientation and position in space of various tools. Once the patient is registered, the tumor’s margins are “visualized” below the scalp so that the surgeon can plan the smallest and safest approach. Resection is assisted by use of the intraoperative microscope and guided by the appearance and consistency of tumor tissue compared with surrounding normal brain. Intraoperative CT, MRI, or ultrasonography can be used to evaluate the completeness of tumor resection. In the case of lesions that are in or near suspected functional cortex, cortical mapping can be performed to localize areas that are critical for motor or speech function. Endoscopy can be used to minimize access for resection of intraventricular lesions or pituitary tumors, as well as for re-establishing pathways of CSF flow, for example, in cases of tumors that have obstructed the cerebral aqueduct, thereby avoiding the need for a CSF shunt. In addition to intraoperative image guidance, the use of ultraviolet-fluorescent tumor-localizing dyes such as 5-aminolevulinec acid has gained acceptance in Europe to enhance the completeness of resection and is being investigated in the United States.
Stereotactic biopsy is performed with either a stereotactic frame or scalp fiducials; a CT or MRI is performed and the data loaded into an image guidance system. Target and entry points are selected, and the trajectory is visualized on a workstation. The entry point is located on the patient’s scalp, and a small burr hole or twist drill hole is made. The biopsy needle is oriented using the image guidance system and passed to the appropriate depth, and tissue samples are obtained. Multiple tissue samples may be obtained along the needle tract, until the pathologist can provide an intraoperative diagnosis. The volume of tissue removed is insufficient to relieve mass effect, and patients who are symptomatic are better treated by open resection.
RADIOTHERAPY
Radiobiologic Considerations Underlying Tissue Injury
The process of radiation injury in the brain is highly complex and dependent on a variety of technical factors, including dose, volume, fraction size, and the specific target cell population, as well as secondary mechanisms of expression of injury such as vascular leak causing edema, vascular endothelial loss resulting in hypoxic injury, reactive gliosis, and to-date inadequately studied host factors. Some structures (e.g., optic chiasm, hypothalamus, lacrimal gland, lenses, etc.) appear to be substantially more sensitive to radiation than others. Even focal lesions may result in widespread radiographic and/or functional perturbations. The time course for the manifestation of injury can be highly variable and the clinical picture easily confounded with tumor progression. The effect on endothelial cells often becomes manifest as an early T2 signal abnormality on MRI, possibly due to disruption of the BBB and edema formation. Metabolic perturbations observed with PET may reflect oligodendroglial demyelination. Further vascular perturbation and regeneration in response to injury results in an enhancing lesion on imaging. Delayed effects include white matter necrosis and vascular obliteration. The time course can be shortened from several months to a few weeks by increasing the volume of brain irradiated or increasing the fraction size or total dose.
Historically, late injury from radiotherapy has been reported as the “tolerance” dose at either the 5% or 50% risk level at 5 years (TD 5/5 or TD 50/5, respectively). The values for whole-brain fractionated radiotherapy at 2 Gy per fraction are 60 and 70 Gy, respectively. With partial-brain irradiation, the corresponding values are 70 and 80 Gy, respectively. In the setting of fractionated radiotherapy with a fraction size <2.5 Gy, recent Quantitative Analysis of Normal Tissue Effects in the Clinic estimates are 5% and 10% rates of symptomatic necrosis at 72- and 90-Gy maximum equivalent doses in 2-Gy fractions, respectively.18 For larger fraction sizes (≥2.5 Gy), incidence and severity of toxicity are unpredictable. In children, cognitive dysfunction has largely been seen after whole-brain doses.
General Concepts
Pertinent Anatomic Landmarks
With conventional simulation, radiographic and surface topographic reference points for appreciation of beam-to-head projection geometry are necessary. The external auditory meatii define anatomic reference planes such as Reid’s baseline and the Frankfort horizontal plane, connecting points in the two external auditory meatii and one anterior infraorbital edge. Unless marked at simulation, the external auditory meatii may be difficult to see on lateral projections because of the overlying temporal bone. The two lateral parts of the anterior cranial fossa, the two anterior parts of the middle cranial fossa floors, and the two mandibular angle points, with their lateral locations, represent appropriate reference points. With CT-based planning, the need for identifying these is obviated.
In a lateral radiograph, the sella turcica is centrally located and marks the lower border of the medial telencephalon and diencephalon. The hypothalamic structures are located an additional 1 cm superior to the sellar floor, and the optic canal runs at most 1 cm superior and 1 cm anterior to that point. The pineal body (or the tentorial notch) usually sits approximately 1 cm posterior and 3 cm superior to the external auditory meatus. The cribriform plate is the most inferior part of the anterior cranial fossa; it is an important reference point for the inferior border of whole-brain irradiation fields. In most patients, little distance is found between the lateral projections of the lens and the most inferior part of the cribriform plate. The temporal lobes are situated in the middle cranial fossae, the floor of which is easily identified on lateral radiographs. Individualized blocks should always be used to delineate the field inferior border for whole-brain radiotherapy.
On an anteroposterior radiograph with a Frankfort horizontal plane (ear markers and one inferior orbital edge in a horizontal plane), the temporal bones (pyramids) project in the orbits. This implies that the ethmoid sinuses and the sphenoid sinus will project between the orbits, the sella just above these air cavities, and the foramen magnum just below the connection line between the inferior orbital edges. The frontal and occipital lobes therefore project above the orbits, and the temporal lobes and cerebellum in and somewhat below the orbits.
Treatment Setup
The head should be positioned so that its major axes are parallel with and perpendicular to the central axis incident beam and the treatment table. It may be preferable to fully flex or extend the neck in some patients, depending on tumor location and choice of beams, although the use of noncoplanar fields and intensity-modulated radiotherapy (IMRT) techniques makes this less necessary.
Reproducibility of head positioning is achieved by using a fixation device. Many devices are available for this, with reproducibility precision ranging from 1 to 5 mm. With the advent of image-guided radiation therapy and intrafraction motion detection, unprecedented accuracy can be achieved in delivering radiotherapy. This permits substantial reduction in margins for setup variability.19
Target Volume Definition
Two major factors drive margin selection: the inaccuracy of estimating the clinical target volume (CTV) and the specific dosimetric and setup variability components that are institution specific and determine the planning target volume (PTV).
Common sense and practice dictate that these CTV expansion margins should not traverse anatomically discontiguous structures or include areas unlikely to be infiltrated by tumor. Inclusion of the bony skull is unnecessary unless direct tumor extension is suspected. With some exceptions, “compartmental crossing” to the contralateral hemisphere or, for example, into the posterior fossa or the brainstem for a supratentorial cortical tumor is not necessary, but because many infiltrating gliomas “cross” through the anterior and/or posterior corpus callosal tracts, these should be adequately included in CTV margin selection.
Radiotherapy Techniques
The most commonly employed radiotherapy techniques in the management of CNS tumors are partial-brain irradiation, whole-brain radiotherapy (WBRT), craniospinal irradiation (CSI), stereotactic radiosurgery (SRS), fractionated stereotactic radiotherapy (FSRT), and, less commonly, brachytherapy. The indications for each of these techniques are discussed under the sections on the individual tumor types. CSI is more frequently used in management of pediatric CNS tumors and is discussed in detail in Chapter 82.
Whole-Brain Radiotherapy
WBRT is used most often for patients with brain metastases but also for patients with primary CNS lymphomas and glioblastomatosis cerebrii and as a component of CSI.
Whole-brain irradiation is administered through parallel-opposed lateral portals. The inferior field border should be inferior to the cribriform plate, the middle cranial fossa, and the foramen magnum, all of which should be distinguishable on simulation or portal localization radiographs (Fig. 35.4). The safety margin depends on penumbra width, head fixation, and anatomic factors but should be at least 1 cm, even under optimal conditions. A special problem arises anteriorly because sparing of the ocular lenses and lacrimal glands may require blocking with margins <5 mm at the cribriform plate.
The anterior border of the field should be approximately 3 cm posterior to the ipsilateral eyelid for the diverging beam to exclude the contralateral lens. However, this results in only approximately 40% of the prescribed dose to the posterior eye. A better alternative is to angle the beam approximately 3 degrees or more (100- or 80-cm source-to-axis distance midline, but also field size dependent) against the frontal plane so that the anterior beam border traverses posterior to the lenses (approximately 2 cm posterior to eyelid markers). Placing a radiopaque marker on both lateral canthi and aligning the markers permits individualization in terms of the couch angle. This arrangement provides full dose to the posterior eyes. However, the eyelid-to-lens and eyelid-to-retina topography is individually more constant than the canthus, and lateral beam eye shielding is better individualized with the aid of CT or MRI scans.20 When in doubt about tumor coverage or lens sparing for tumors in a subfrontal or middle cranial fossa location, one should consider CT-based contouring and planning.
FIGURE 35.4. Lateral portal localization film of whole brain, illustrating adequate inclusion of the cribriform plate and the anterior and middle cranial fossae.
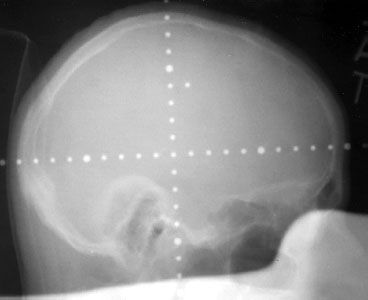
Craniospinal Irradiation
Traditional CSI techniques use opposed lateral cranial fields and one or more posterior spinal fields, depending on patient size. The junctioning of noncoplanar fields in the cervical region is potentially hazardous because of the risk of overlap resulting in radiation myelitis. Consequently, great attention needs to be paid to precise immobilization, and a variety of immobilization devices are available for this purpose. Image guidance during radiotherapy can help in ensuring day-to-day reproducibility. The prone position permits direct visualization of the light field from the linear accelerator on the patient, thereby allowing daily adjustments of the junctions. However, if anesthesia or sedation is required, as may be the case with young children, a supine setup may be considered safer.21 To avoid the risk of dose overlap, at least two techniques (with numerous variations) are commonly used, and several variations of these have also been reported. In the first, a gap is used between abutting fields such that the beam edges intersect deep to the spinal cord. This gap could result in a cold spot in a small segment of the spinal cord. If the beam intersection point were raised dorsally, a hot spot would result.
The second technique attempts to avoid this problem by using a half-beam technique in which the caudal edge of the brain is matched precisely with the cephalad edge of the abutting spine field without cold or hot spots. This requires collimator angulation and sometimes a couch rotation as well. For both techniques, the use of moving junctions (known as “feathering”) smoothes out any dose inhomogeneity.22 Several studies have demonstrated that adequate coverage of the subfrontal region, posterior fossa, and depth assessment of the cord requires CT-based planning. Given the complexity of CSI, we recommend that it be delivered at centers with adequate staff, experience, and expertise.
With sophisticated techniques such as helical intensity-modulated, image-guided tomotherapy and volumetric arc-modulated radiotherapy, it is possible to treat the entire neuraxis in a single setup.23 In recent years, protons, as well as other techniques, have been used for CSI.
Stereotactic Radiosurgery
Stereotactic radiosurgery requires a team comprised at a minimum of a neurosurgeon, radiation oncologist, and radiation oncology physicist, in addition to appropriate support staff. SRS can be delivered using a conventional or modified linear accelerator (LINAC) system, a Gamma Knife (Elekta Corp., Stockholm), or a robotically controlled miniaturized linear accelerator (CyberKnife; Accuray, Sunnyvale, CA). In LINAC radiosurgery circular or oval collimators ranging from 4 to 40 mm are used to collimate the treatment beam into a circular pencil beam, and treatment is delivered using multiple noncoplanar arcs that intersect at a single point to treat an approximately spherical target of <4 cm in diameter. Newer miniaturized multileaf collimators allow beam shaping. The Gamma Knife is a fixed-beam, multisource radiation unit containing 201 cobalt-60 (60Co) sources that are collimated using a helmet with circular apertures ranging from 4 to 18 mm that are focused onto a single target point. The newer Gamma Knife technology obviates the need for “helmet changes” and can effectively allow IMRT-like dose distributions to be created. For irregularly shaped lesions, treatments delivered using either noncoplanar arcs delivered through a single circular collimator or a single collimator helmet lead to the inclusion of a large amount of normal brain and yield inferior conformality. In these cases it is advantageous to use multiple circular collimators or collimator helmets placed on different target points (and in the newest version of the Gamma Knife [Perfexion], different segments can be treated with different collimator diameters, and differential weighting is also possible), or to consider the use of multileaf collimated beams.
Radiation Therapy Oncology Group (RTOG) study 90-05 established the maximum tolerated dose of single faction SRS to be 24, 18, and 15 Gy for tumors ≤20 mm, 21 to 30 mm, and 31 to 40 mm in maximum diameter, respectively, and these and other parameters are frequently used to guide prescription doses.24
Fractionated Stereotactic Radiotherapy
For lesions larger than 4 cm and/or located in critical regions, the delivery of a single large-fraction treatment as in SRS is not desirable because of a high risk of CNS toxicity. Fractionated stereotactic radiotherapy (FRST) is a hybrid between conventionally fractionated radiotherapy and SRS that combines fractionation with stereotactic localization and targeting techniques. Various systems for FSRT have been developed, with a reported accuracy of 1 to 3 mm.25–27 As for SRS, the use of multiple arcs and circular collimators for irregularly shaped lesions leads to the inclusion of a large amount of normal tissue. The use of multiple noncoplanar fixed fields each having a unique entrance and exit pathway is preferable because of better conformality because multileaf collimators are almost always used.19,28 With the Perfexion device, the use of the “Extend” frame permits FSRT, as well as targeting of lower cranial lesions.
Heavy Charged Particles
Heavy-charged-particle beams deposit their dose at a depth that depends on their energy over a distance of few millimeters when the heavy charged particles come to rest, the so-called Bragg peak. To cover a larger volume, the particle beam can be modulated, in effect adding up multiple Bragg peaks. The very sharp dose gradient at the distal edge permits the use of high-dose radiotherapy for tumors in critical locations, such as at the clivus and base of skull, and provides better normal-tissue sparing in other situations, especially, for example, in craniospinal irradiation.29 A small number of European and Japanese centers are treating CNS tumors with carbon ions, which putatively have higher linear energy of transfer and presumed superior biologic effect, but no substantial clinical data are available to back these claims. There are no U.S. centers using these technologies, although neutron-beam irradiation, with or without coadministration of boronated agents to increase the cross-sectional area of interaction, continues to be used in some U.S. centers.
Brachytherapy and Radiocolloid Solutions
Selection criteria for brachytherapy include tumor confined to one hemisphere, no transcallosal or subependymal spread, small size (<5 to 6 cm), well circumscribed on CT or MRI, and accessible location for the implant. A balloon-based system, GliaSite (IsoRay Medical, Richland, WA), placed into the cavity at the time of surgery has been employed in the treatment of recurrent malignant gliomas whose largest spatial dimension is <4 cm and are roughly spherical.30 After treatment planning the balloon is filled with a liquid that contains organically bound iodine-125 (125I), and treatment is completed within 3 to 7 days. Direct infusion of radioimmunoglobulins has been used in primary and recurrent brain gliomas.31
CHEMOTHERAPY AND TARGETED AGENTS
Conventional Chemotherapy
Many conventional chemotherapy agents do not adequately penetrate normal or nonenhancing tumor-infiltrated brain, whereas some drugs, despite having a molecular weight and chemical structure that make them appear capable of crossing the BBB, are p-glycoprotein and other active transporter substrates that are actively effluxed out of the brain parenchyma. Even when drug delivery is adequate, most CNS tumors are resistant to most chemotherapeutic agents. Alkylating agents such as carmustine (BCNU) and lomustine (CCNU) have been the most widely studied drugs in CNS tumors. These agents cross the BBB, but prolonged use is difficult because of cumulative myelotoxicity and the dose-related risk of pulmonary fibrosis. Despite radiographic responses in 15% to 40% of patients, the impact on survival has been modest at best. Procarbazine has similar efficacy but is better tolerated. Cisplatin and carboplatin have been used as either single agents or in combination regimens. Response rates have been modest and their impact on survival is unclear. Topoisomerase I (CPT-11, irinotecan) and topoisomerase II inhibitors (etoposide) have shown only modest activity. Taxanes, such as paclitaxel, have not demonstrated activity as single agents. Temozolomide, an oral agent with excellent bioavailability, has a good toxicity profile and is the only agent to demonstrate a survival benefit for glioblastoma patients in randomized clinical trials.
Combination regimens, such as BCNU and temozolomide, are not more efficacious,32 because of the need to reduce the dose of each agent due to overlapping myelotoxicity.
Direct Delivery of Therapeutic Agents
Methods for circumventing the BBB include implantation of slow-release chemotherapy wafers into a tumor resection cavity,33,34 pharmacologic or osmotic BBB disruption,35,36 and convection enhanced drug delivery (CED). CED involves the use of intracerebrally implanted catheters to deliver a drug into the brain parenchyma or tumor at a slow but continuous rate of flow. Unlike diffusion, in which a drug distributes along an exponentially decaying concentration gradient depending on the size of the molecule, drug distribution by CED is less size dependent, occurs over a larger volume of brain tissue, and results in a more uniform drug concentration within the volume of distribution.37 Large and/or hydrophilic agents that do not cross the BBB are ideal candidates for delivery via CED. Examples of agents used in CED studies include viruses,38 paclitaxel,39 topotecan,40 and a variety of engineered, targeted protein toxins. These toxins are engineered to include a targeting ligand (e.g., interleukin-4, interleukin-13, tumor growth factor-α, and transferrin) and a genetically altered bacterial toxin that is effective only when internalized by a cell that expresses the target of the ligand.41–44 Two phase III clinical trials have evaluated the use of CED. The PRECISE trial45 studied CED of interleukin-13 linked to Pseudomonas exotoxin (cintredekin besudotox) as compared to Gliadel (Eisai, Woodcliff Lake, NJ) wafers in glioblastoma patients at first recurrence. No difference in overall survival was observed, but cintredekin besudotox was associated a higher pulmonary embolism rate. The TransMID trial evaluated the efficacy of transferrin-CRM107 delivered by CED to patients with inoperable recurrent or progressive glioblastoma. The trial was terminated early in 2007 because it was deemed unlikely to meet the prespecified criteria for efficacy.
BCNU impregnated in a polymer and made into a wafer has been used for local delivery, placed on the walls of the resection cavity at the time of surgery. The wafer slowly undergoes biodegradation, releasing the active drug. This local delivery system has the advantages of minimal systemic toxicity, no limitation posed by the BBB, and delivery of very high local concentrations of chemotherapy. Studies in glioblastoma multiforme (GBM) have shown only marginal benefit.34,46
Targeted Agents
The molecular changes in gliomas offer opportunities for targeted therapies. Signal transduction pathways, for example, are often markedly enhanced and may contribute to the cancer phenotypic and biologic changes. Molecules that block signal transduction pathways are undergoing extensive investigation.47 These include agents that block angiogenesis (vascular endothelial growth factor receptor); proliferation, tumor cell invasion, and survival (EGFR); and cell survival (platelet-derived growth factor receptor); as well as inhibitors of downstream signaling molecules such as Akt, Ras, Raf kinase, and mTOR. Because single-agent strategies have shown minimal efficacy, clinical trials are now focusing on combination regimens.47
FOLLOW-UP
The follow-up schedule for a brain tumor patient must be frequent enough to check on side effects and to taper steroids shortly after completion of treatment. Periodic MRIs are used to detect tumor recurrence at a stage when further therapy may be contemplated. Assessment of intellectual functioning and quality of life is important, and patients must be monitored for neuroendocrine and ophthalmologic side effects.
SEQUELAE OF TREATMENT
Surgery
With appropriate patient selection, diligent surgical technique, and use of surgical adjuncts such as speech and/or motor mapping, the rate of complications can be minimized. Even in the best of hands new temporary neurologic deficits can be seen in 15% or more of patients, although the rate of permanent new deficits is typically now <5%.48 The incidence and types of deficits seen following surgery depend upon the location of the tumor and the deficits present preoperatively. The most common complications associated with surgery are bleeding and infection, particular in the case of reoperation in a patient who has received prior radiotherapy and/or chemotherapy or when chemotherapy wafers are placed into a resection cavity.49 It has been suggested that the use of linear incisions (as opposed to U-shaped flaps) can help reduce the incidence of incision-related complications, such as infection.50 Posterior fossa resections, particularly in children with medulloblastoma, may be associated with posterior fossa syndrome (mutism plus bulbar symptoms). Transient perioperative edema, within about 48 hours of surgery, may be responsible for early postoperative neurologic worsening and can often be mitigated with the use of a short course of high-dose steroid therapy.
Patients with postoperative neurologic deterioration require careful clinical assessment, and in most cases a CT or MRI is required to determine the cause of the deterioration. MRI diffusion-weighted sequences can be used to detect the presence of a new infarct. A high index of suspicion should be maintained for postoperative infection because symptoms may be masked by perioperative steroid use, and the headache and fever associated with craniotomy may obscure the classic signs of meningitis.
Radiotherapy
The response of intracranial tissues to radiation has been classically divided into three phases based on the timing of onset of symptoms: acute, subacute, and late.
Acute Toxicity
Transient worsening of pretreatment deficits may develop during the course of treatment, and further acute toxicities may manifest up to 6 weeks following completion of irradiation. These symptoms are believed to be the consequence of a transient peritumoral edema and usually respond to a short-term increase or the institution of corticosteroids. Persistent or refractory symptoms may be caused by tumor progression, and repeat imaging while under treatment may be indicated if the clinical condition worsens despite steroids.
General symptoms such as fatigue, headache, and drowsiness may be seen, especially in individuals treated with large brain fields or with CSI. A mild dermatitis that develops in irradiated areas may be treated with topical agents if necessary. Alopecia within the irradiated areas is common and may be permanent with higher total doses. Nausea and vomiting independent of changes in intracranial pressure may occur, particularly with posterior fossa or brainstem irradiation. Otitis externa can be seen if the ear is included in the irradiation fields, and serous otitis media also may occur. Patients treated with CSI with photons are at risk for mucositis and esophagitis because of the exit dose from the spinal fields through the oropharynx and mediastinum. Hematologic toxicity may also be seen in these patients due to irradiation of the vertebral bodies, a major depot of bone marrow in adults.
Subacute Toxicity
Subacute or “early-delayed” toxicity that develops during the 6-week to 6-month period following irradiation is attributed to changes in capillary permeability, as well as to transient demyelination due to damage to oligodendroglial cells. Symptoms, which include headache, somnolence, fatigability, and deterioration of pre-existing deficits, usually respond to steroids. The main challenge is to distinguish the clinical and imaging findings from tumor recurrence. The phenomenon of pseudoprogression temporally fits within the subacute toxicity time frame.
Late Sequelae
Late sequelae of radiotherapy appear from 6 months to many years following treatment and are usually irreversible and progressive. They are believed to be due to white matter damage from vascular injury, demyelination, and necrosis. The pathophysiology of radiation-induced neurocognitive damage is complex and involves intercellular and intracellular interactions between vasculature and parenchymal cells, particularly oligodendrocytes, which are important for myelination. Oligodendrocyte death occurs either due to direct p53-dependent radiation apoptosis or due to exposure to radiation-induced tumor necrosis factor-α.51,52 Postradiation injury to the vasculature involves damage to the endothelium leading to platelet aggregation and thrombus formation, followed by abnormal endothelial proliferation and intraluminal collagen deposition.53
The most serious late reaction to radiotherapy is radiation necrosis, which has a peak incidence at 3 years. Radiation necrosis can mimic recurrent tumor clinically by the reappearance and worsening of initial symptoms and neurologic deficits and radiographically with the development of a progressive, irreversible, enhancing mass with associated edema on imaging. PET, MR spectroscopy, and nuclear and dynamic CT scanning procedures may aid in the differentiation of radiation necrosis from recurrent tumor. The best treatment for symptomatic necrosis is control of symptoms with steroids, followed by surgical debulking, although even after resection necrosis may progress. Given the central role of capillary leakage to radiation necrosis, bevacizumab, an antibody against vascular endothelial growth factor (VEGF), has been tested clinically as treatment for radiation necrosis and has shown encouraging results.54 Other measures include use of corticosteroids with anticoagulation or hyperbaric oxygen, although randomized trials have not shown these to be useful. Although focal necrosis is usually due to radiotherapy alone, diffuse leukoencephalopathy is more commonly associated with the combination of radiotherapy and chemotherapy, particularly methotrexate.
Inclusion of the middle ear may result in high-tone hearing loss and vestibular damage, especially in patients who receive cisplatin. Retinopathy or cataract formation may be seen if the eye is in the radiation field. Optic chiasm and nerve injury may manifest as a decrease in visual acuity, visual field changes, or blindness at doses >54 to 60 Gy. Onset of hormone insufficiency from irradiation of the hypothalamic–pituitary axis is variable but may be seen with doses as low as 20 Gy.
Cranial irradiation can produce neuropsychologic changes and neurocognitive impairment; other factors, such as tumor-related morbidity, as well as the effects of surgery and chemotherapy, may also contribute.53,55 Decline in list-learning recall has been observed in prospective clinical trials of therapeutic and prophylactic cranial irradiation.56,57 These changes are believed to be due to interactions between the vasculature and parenchymal cells. Hippocampal-dependent functions of new learning, memory, and spatial information processing appear to be most affected.58 Doses as low as 2 Gy can induce apoptosis in the proliferating cells in the hippocampus.59 Agents such as methylphenidate and memantine or radiotherapeutic strategies such as hippocampal avoidance may improve neurocognitive function.60–62
MANAGEMENT OF INDIVIDUAL TUMORS
Malignant Glioma
Malignant or high-grade gliomas account for approximately half of all primary brain tumors in adults. They are rapidly growing tumors that directly invade the brain parenchyma but almost never metastasize outside the CNS. They present in any age group, although most occur in late adulthood. Malignant gliomas correspond to anaplastic gliomas (WHO grade III) and GBM (WHO grade IV). Molecular genetics and results from clinical series have shown these to be two distinct diseases with unique behavior, response to treatment, and prognosis. Historical trials that included grade III and IV gliomas are described in the section on GBM because patients with GBM comprised the majority of subjects, and anaplastic gliomas are discussed in further detail in the following section.
Glioblastoma
GBM accounts for approximately 75% of all high-grade gliomas. The histopathologic features of GBM include nuclear atypia, mitotic activity, vascular proliferation, and necrosis; any three of these suffice to make the diagnosis. GBM is diffusely infiltrative, involving large portions of the brain. MRI characteristically shows vasogenic edema and ring enhancement around central necrotic regions.
The prognosis for patients with GBM is poor, with median survival time of approximately 14 months in highly selected patients receiving contemporary treatment. Pretreatment patient and tumor characteristics such as age at diagnosis, tumor histology, and Karnofsky performance status (KPS) are the best predictors of outcome. Extent of resection, duration of neurologic symptoms, and radiographic response to treatment have also been suggested as predictors of survival.
Curran et al.63 used nonparametric recursive partitioning analysis (RPA; a statistical tool that allows for the identification of significant prognostic factors and subsequent classification of patients into groups with similar outcomes) to analyze data from three RTOG trials that included 1,578 patients with malignant gliomas. Age was the most important predictor of survival, with patients younger than 50 years faring best; KPS ≥70 was the next-most-significant prognostic factor. Taking into account these and other variables, it is possible to divide patients into groups with similar outcomes, with 2-year overall survival ranging from 4% to 76% and median survival ranging from 2.7 to 58.6 months (Table 35.4). Although developed in patients receiving radiotherapy alone, the RPA classification retains prognostic significance in patients treated with radiotherapy plus temozolomide.64 More recent studies integrated molecular data from O6–methylguanine-DNA methyltransferase (MGMT) promoter methylation status and other novel biomarkers to develop novel risk-stratification models.65,66
TABLE 35.4 RADIATION THERAPY ONCOLOGY GROUP RECURSIVE PARTITIONING ANALYSIS OF MALIGNANT GLIOMA
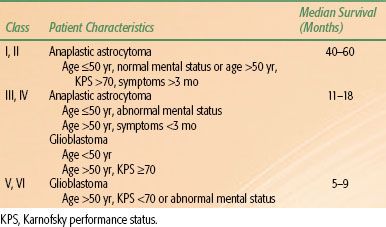
Molecular Genetics
Glioblastoma may arise de novo (primary) or from a low-grade glioma that has transformed into a higher-grade tumor (secondary). Some series report that up to 40% of GBM are secondary. Secondary GBMs arise in younger patients and are associated with a more favorable prognosis than for those with primary GBM. The more favorable prognosis may be related to the impact of younger age and better performance status of this group. Emerging data suggest that promoter methylation of the PTEN gene in low-grade gliomas may be causally linked to secondary malignant transformation to GBM. Secondary GBMs usually have a mutation in the isocitrate dehydrogenase gene and often have p53 mutations.
Treatment
Standard treatment consists of maximal safe surgical resection followed by radiotherapy with concurrent temozolomide chemotherapy and subsequent adjuvant temozolomide chemotherapy. Other approaches including alterations in the delivery of radiotherapy, newer chemotherapeutic agents, and radiosensitizers, and other agents are the subject of ongoing research.
Radiotherapy Target Volume
Randomized trials have demonstrated a clear survival benefit to the use of radiotherapy after surgery.67 Localized irradiation volumes are recommended despite the fact that GBM is usually more widely disseminated. Dandy,68 for example, identified recurrences in the contralateral hemisphere even after hemispherectomy, showing the phenomenal capability of malignant gliomas to spread along white matter tracts. Such findings, as well as autopsy studies,69–71 led to recommendations that the entire intracranial contents should be irradiated. However, Hochberg and Pruitt72 reported that in 35 patients who had a CT scan within 2 months prior to autopsy, 78% of recurrences of GBM were within 2 cm of the margin of the initial tumor bed and 56% were within ≤1 cm of the volume outlined by the CT scan. These findings were confirmed by Wallner et al.,73 who showed that 78% of unifocal tumors (25 of 32) recurred within 2 cm of the initial tumor volume, defined as the enhancing edge of the tumor on CT scan, and 56% of tumors (18 of 32) recurred within 1 cm of the initial tumor margin. No unifocal tumor recurred as a multifocal lesion, and large tumors were not more likely to recur farther from the initial tumor margin than were smaller tumors.
In a correlative study, Halperin et al.74 reviewed CT scans and pathologic sections of 15 brains of patients with GBM who received minimal or no radiotherapy. If radiation treatment portals had been designed to cover the contrast-enhancing volume and peritumoral edema with a 1-cm margin, the portals would have covered histologically identified tumor in only 6 of 11 cases. However, treatment of the contrast-enhancing area and all surrounding edema with a 3-cm margin around the edema would have covered histologically identified tumor in all cases.
Kelly et al.75 reported on 40 patients with intracranial glial neoplasms who underwent CT- and MRI-guided stereotactic serial biopsies. Histologic analysis of 195 biopsy specimens showed that contrast enhancement most often corresponded to tumor tissue without intervening parenchyma, and hypodensity most often corresponded to parenchyma infiltrated by isolated tumor cells, tumor in low-grade gliomas, or edema. Isolated tumor cell infiltration extended at least as far as T2 changes on MRI. T2-weighted MRI revealed much larger volumes of infiltrated parenchyma than shown by low attenuation on CT scans.
Therefore, inclusion of all radiographic evidence of tumor and associated edema with generous margins is the rule in the design of treatment portals. With advances in MRI technology, the definition of tumor margins may change. For example, Pirzkall et al.76 showed metabolically active tumor extending outside the region defined on T2-weighted MRI in 88% of patients. PET imaging with methionine and/or thymidine may also prove useful.
Radiotherapy Dose
Standard therapy is a total dose of 60 Gy in 30 to 33 fractions. Walker et al.77 reported a dose–response analysis using data from 420 patients treated on Brain Tumor Cooperative Group protocols. Doses ranged from <45 to 60 Gy, using daily fractions of 1.7 to 2 Gy; only one-third of the patients received <60 Gy. A significant improvement in median survival from 28 to 42 weeks in the groups treated with doses of 50 to 60 Gy was found. A Medical Research Council study of 443 patients also showed a significant survival advantage in patients who received 60 Gy compared to those who received 45 Gy (12 vs. 9 months; p = .007).78
For patients with poor pretreatment prognostic factors and a limited expected survival who are not able to tolerate conventional treatment, a shorter course of treatment may provide good palliation. Older patients (>65 years), especially those with poor performance status, have been shown to experience limited posttreatment improvement or rapid neurologic deterioration following conventional radiotherapy. Short-course radiotherapy has been tested in older patients, and results are described later.
Dose Escalation and Altered Fractionation
With the vast majority of tumor recurrences occurring within the previous irradiation field and the poor outcomes associated with standard therapy, regimens designed to deliver a larger dose have been attempted to improve local control and enhance survival.
A benefit for doses >60 Gy using conventional treatment has not been demonstrated. The RTOG and Eastern Cooperative Oncology Group (ECOG) randomized 253 patients to either whole-brain irradiation to 60 Gy given in 6 to 7 weeks or 60 Gy plus a 10-Gy boost to a limited volume given in 7 to 8 weeks.79 There was no benefit for the higher irradiation dose. Median survival was 9.3 months for patients receiving 60 Gy and 8.2 months for those receiving 70 Gy.
Dose intensification using 3D conformal radiotherapy or IMRT also has not consistently shown to improve clinical outcome. Chan et al.80 published the results of 34 patients with high-grade gliomas treated using 3D conformal IMRT to a dose of 90 Gy. At median follow-up of 11.7 months, median survival was found to be 11.7 months, and 1- and 2-year survivals were 47.1% and 12.9%, respectively, comparable to historical controls. In contrast, a retrospective study by Tanaka et al.81 suggested a survival advantage for patients treated with high-dose conformal radiotherapy.
Several groups have used hyperfractionated or accelerated regimens as a means to escalate dose, using twice-daily, three-times-daily, and even four-times-daily fractionation.82–84 Only the study of Shin et al.84 showed an improvement in survival using daily fractionation. In this study, 81 patients were randomized to 61.4 Gy in 69 fractions of 0.89 Gy given three times daily over 4.5 weeks or conventional fractionation to 58 Gy in 30 fractions given once daily over 6 weeks. Median survival in the two groups was 39 and 27 weeks, respectively, and the 1-year survival rates were 41% and 20%, respectively (p < .001).
Others have failed to confirm these results. In a prospective, randomized, phase I/II trial, RTOG 83-02 examined dose escalation using twice-daily fractionation in patients with malignant gliomas. Hyperfractionated regimens studied were 64.8, 72.0, 76.8, and 81.6 Gy given in 1.2-Gy fractions twice daily, and accelerated hyperfractionated regimens were 48 and 54.4 Gy given in 1.6-Gy twice-daily fractions. Patients also received chemotherapy with BCNU. In the final report on all 747 patients, there were no significant differences between the treatment arms with regard to median survival time.85 Late toxicities were slightly increased with higher doses. A phase III trial compared conventional radiotherapy to 60 Gy in 30 daily fractions to hyperfractionated radiotherapy to 72 Gy in 60 fractions of 1.2 Gy given twice daily.86 No difference in survival was found. Several other accelerated hyperfractionation regimens to doses over 70 Gy have been investigated, also without significant improvement in survival.87,88
Dose Escalation Using Radiosurgery and FSRT
A radiosurgical boost was reported as effective in patients with newly diagnosed malignant glioma in a retrospective analysis of 115 patients treated at three institutions with a combination of surgery, external-beam radiotherapy, and LINAC-based radiosurgery on similar institutional protocols.89 The actuarial 2-year and median survival for all patients was 45% and 96 weeks, respectively. In comparison to results for 1,578 patients treated on three RTOG external-beam radiotherapy protocols from 1974 to 1989, patients treated with radiosurgery had significantly improved 2-year and median survival (p = .01). This improvement in survival was seen predominantly for the worse prognostic classes (RPA classes 3 to 6). Patient selection may in part account for these results: it has been estimated that selection criteria limit the application of radiosurgery to approximately only 20% to 30% of patients with GBM.
In a prospective randomized trial, Souhami et al.90 compared conventional radiotherapy (60 Gy) plus adjuvant BCNU with and without radiosurgery in 203 patients with GBM. At a median follow-up of 61 months, no significant improvement in median survival was observed (13.5 vs. 13.6 months). There was no difference in failure pattern between the two groups, and measurements of quality of life and cognitive decline were found to be comparable as well.
The use of a boost using FSRT was tested prospectively in RTOG 0023.91 76 patients with GBM with postoperative residual tumor plus tumor cavity diameter <60 mm were treated with 50-Gy standard radiotherapy in daily 2-Gy fractions plus four FSRT treatments given once weekly during weeks 3 to 6 of radiotherapy. The FSRT dose was either 5 or 7 Gy per fraction for a cumulative dose of 70 or 78 Gy in 29 treatments over 6 weeks. Significant toxicity included three patients with acute grade 4 toxicity (neurologic, constitutional, metabolic) and one with grade 3 late necrosis. The median survival time was 12.5 months. Overall, no survival advantage was seen when compared to the RTOG historical database.
Dose Escalation Using Brachytherapy
Laperriere et al.92 used brachytherapy as a boost to conventional radiotherapy in patients with malignant gliomas. Patients were randomized to external-beam radiotherapy (50 Gy in 25 fractions) alone (n = 69) or external-beam radiotherapy plus a temporary stereotactic 125I implant delivering a minimum peripheral tumor dose of 60 Gy (n = 71). Median survival was not significantly different between the two arms (13.8 vs. 13.2 months; p = .49).
The results of the Brain Tumor Cooperative Group National Institutes of Health Trial 8701 reported by Selker et al.93 support these findings. In this randomized, prospective trial, 299 patients with newly diagnosed malignant glioma received surgery, external-beam radiotherapy, and BCNU with or without an interstitial radiotherapy boost with 125I. Treatment with an interstitial boost did not prolong survival as compared to conventional treatment.
Radiotherapy delivered by an inflatable balloon catheter is a newer approach to brachytherapy. Tatter et al.30 evaluated the safety and performance of one such device (GliaSite Radiation Therapy System; Cytyc, Marlborough, MA). Twenty-one patients with recurrent malignant gliomas underwent surgical resection and implantation of a subcutaneous port. At 1 to 2 weeks following implantation, the catheter was filled with an aqueous solution of organically bound 125I for delivering a minimum of 40 to 60 Gy over 3 to 6 days, with subsequent removal of the device. This treatment was well tolerated with no serious adverse effects. Median survival was 12.7 months. Prospective, randomized trials are needed for further evaluation.
Dose Escalation Using Proton Therapy
Dose escalation up to 60Co gray equivalent (CGE) with mixed photon and proton beam irradiation has been tested in two prospective trials.
Fitzek et al.94 reported on a phase II study of 23 patients with newly diagnosed GBM. The 2-year overall survival was 34%, and median overall survival was 20 months, which compared favorably to historical data. Recurrence was observed in regions treated to 60 to 70 CGE, but only 1 recurrence was observed in regions treated to 90 CGE. More recently, Mizumoto et al.95 reported on a phase I/II study of 20 patients with supratentorial GBM treated with mixed photon and proton beam irradiation to 96.6 Gy in 56 twice-daily fractions with concomitant nimustine chemotherapy. MRI-defined T2-enhancing region was treated to 50.4 CGE in 28 daily morning fractions. In daily evening fractions, patients were treated to 23.1 CGE for the first 14 fractions to the T1-enhancing region plus 1 cm and then 23.1 CGE for the subsequent 14 fractions to the T1-enhancing region only. Median survival was 22 months, and 2-year overall survival was 45%. Late radiation necrosis was noted in 1 patient, and late leukoencephalopathy was observed in a second patient. Given its high cost, proton therapy requires further prospective, randomized trials to validate these single-institution findings.
Radiosensitizers
Studies using radiation modifiers in conjunction with radiotherapy to overcome the hypoxia present in malignant gliomas have shown disappointing results. Chang96 reported on 38 patients treated with hyperbaric oxygen and irradiation using fractionation schedules ranging from 36 Gy given in 3 weeks to 60 Gy given in 6 to 7 weeks and compared them with 42 patients treated with radiotherapy alone. An improvement in 18-month survival rate from 10% to 28% and an increase of median survival from 31 to 38 weeks was noted. The increased cost and difficulty of the widespread application of this treatment make this approach impractical. Perfluorocarbon emulsions, such as Fluosol, which have enormous oxygen-carrying capacity, have been tested as hypoxic sensitizers without significant benefit.97 Randomized studies failed to show significant improvement with the addition of the hypoxic cell sensitizer misonidazole.82,98
Miralbell et al.99 reported the results of a European Organisation for Research and Treatment of Cancer (EORTC) trial examining the addition of carbogen and nicotinamide to overcome the effects of proliferation and hypoxia presumed responsible for radioresistance in GBM. In this prospective phase I and II trial, 107 eligible patients received radiotherapy (60 Gy) with carbogen breathing during each treatment session (n = 23), a daily oral dose of nicotinamide (n = 28), or both (n = 56). Patients receiving nicotinamide had higher rates of acute toxicity. Overall survival was similar in all three groups (median survival 10.1 vs. 9.7 vs. 11.1 months) and did not differ from results of series using radiotherapy alone.
The redox-modulating radiosensitizer motexafin gadolinium (MGd) showed encouraging results in a phase I clinical trial74; however, results from a single-arm phase II trial, RTOG 0513, of MGd and conventional therapy in newly diagnosed GBM showed no survival improvement.
Chemotherapy
The use of cytotoxic chemotherapeutic agents for glioblastoma dates back to the 1960s when the Brain Tumor Study Group conducted a controlled study using carmustine.100 After surgery, patients were assigned to one of four treatment groups: (a) no further therapy, (b) carmustine alone, (c) radiation therapy, and (d) radiation therapy followed by carmustine. At 18 months 23% of patients who received radiation therapy plus carmustine were still alive as compared to 5% with carmustine or radiotherapy alone. The U.S. Food and Drug Administration (FDA) approved carmustine and lomustine for the treatment of brain tumors (including glioblastoma) in the 1970s. Subsequent prospective studies failed to demonstrate a survival advantage to carmustine or other cytotoxic chemotherapy for glioblastoma, although two meta-analyses demonstrated a small survival benefit from chemotherapy.101,102
The only chemotherapeutic agent that has demonstrated efficacy in a randomized, controlled clinical trials is temozolomide, an oral imidazotetrazine derivative of dacarbazine that is metabolized in vivo to an active agent. Like the nitrosureas, it alkylates the O6 position on guanine, producing single-strand DNA breaks. It is well tolerated by patients; fatigue, constipation, and nausea are the most common toxicities. The drug is myelosuppressive in a minority of patients. Approval for the treatment of recurrent anaplastic astrocytoma was obtained from the FDA in 1999 based on the work of Yung et al.103 Its approval for use as adjuvant therapy for glioblastoma was based on a large phase III clinical trial conducted by the EORTC and the National Cancer Institute of Canada (NCIC).104,105 This phase III trial randomized 573 patients with newly diagnosed glioblastoma (between the ages of 18 and 70 years and KPS > 70) to either radiation therapy alone (total 60 Gy in 30 fractions; control arm) or temozolomide chemotherapy in combination with radiation therapy (total 60 Gy in 30 fractions; experimental arm). Patients on the experimental arm received temozolomide daily during radiation therapy (because of its radiosensitization effect in preclinical studies) at a dose of 75 mg/m2, followed by monthly temozolomide at a dose of 150 to 200 mg/m2 on a 5 of every 28 days schedule for six cycles.
Patients randomized to the experimental arm had a median survival of 14.6 months as compared to 12.1 months for the control arm. The 2-year survival of patients treated with radiation therapy plus chemotherapy was 26% as compared to 6% for radiation alone. This impressive increase in 2-year survival with minor improvement in overall survival suggested that there was an unspecified subpopulation of patients that responded to chemotherapy. Toxicity with chemoradiotherapy was acceptable with 7% grade 3 or 4 hematologic toxicities, compared to none in the group treated with radiotherapy alone.
The survival benefit from the addition of temozolomide has now been demonstrated for at least 5 years out from initial treatment and in all clinical prognostic subgroups, including patients aged 60 to 70 years and in RPA classes III through V.105 Five-year overall survival was 9.8% for patients who received combined temozolomide and radiotherapy as compared to 1.9% for those who received radiotherapy alone.
The RTOG recently completed a 1,100-patient, randomized, phase III trial comparing standard adjuvant temozolomide with a dose-dense schedule in newly diagnosed glioblastoma.66 A total of 833 patients were randomized to receive either standard therapy (temozolomide plus radiotherapy followed by 6 to 12 cycles of temozolomide at a dose of 150 to 200 mg/m2 on a 5/28 day schedule) or dose-intense temozolomide (temozolomide plus radiotherapy followed by 6 to 12 cycles of temozolomide at a dose of 150 mg/m2 on a 21/28 day schedule). There was no statistical difference between the experimental and standard arms for overall survival (16.6 vs.14.9 months, p = .63) or progression-free survival (5.5 vs. 6.7 months, p = .06), indicating no additional benefit from dose-intense temozolomide. The trial prospectively stratified for MGMT methylation status, and no survival benefit with dose-intense therapy was identified in any subgroup. As expected, the dose-intense arm resulted in increased toxicity. Thus, at the present time, there is no role for dose-intense temozolomide for newly diagnosed glioblastoma patients.
Because only a subgroup of patients benefit from temozolomide, their identification is desirable to avoid exposing them to the toxicity of a potentially ineffective therapy. Recent work has focused on identifying potential predictive laboratory markers. Epigenetic silencing of the MGMT DNA repair gene by promoter methylation has been associated with longer survival for malignant glioma patients treated with alkylating agents.106 A retrospective analysis of assessable cases from the EORTC/NCIC temozolomide study demonstrated a survival benefit for patients treated with temozolomide and radiotherapy if their tumor contained a methylated MGMT promoter (median, 21.7 months) as compared to patients with nonmethylated MGMT promoters (median, 12.7 months).107 Prospective data supporting the prognostic value of MGMT promoter methylation were obtained in the RTOG dose-intense temozolomide study (RTOG 0525).66 MGMT methylation was associated with improved median overall survival (21.2 vs. 14 months, p <.0001), median progression-free survival (8.7 vs. 5.7 months, p <.001), and response (p = .012).
Other chemotherapeutic regimens, such as the combination of CPT-11 and temozolomide, have shown promising results in a phase II trial with an objective response rate of 25% and 6-month progression-free rate of 38%.108 When tested prospectively in a single-arm RTOG trial, the regimen did not show improved survival. Buckner et al.109 reported on a phase III trial of carmustine with or without cisplatin before and concurrently with radiotherapy and observed increased toxicity but no survival benefit with the addition of cisplatin.
Conventional cytotoxic chemotherapeutic agents have also been combined with agents designed to modulate tumor biology. Two large phase III, randomized clinical trials investigating the addition of bevacizumab to the EORTC/NCIC regimen have completed accrual, and results are pending.
Investigational Approaches
Radioimmunotherapy
Radioimmunotherapy using monoclonal antibodies against EGFR tagged with 125I has been evaluated in the treatment of high-grade gliomas. In a phase II trial by Brady et al.,110 25 patients with malignant gliomas (10 with anaplastic astrocytoma and 15 with GBM) were treated with surgical resection or biopsy, followed by definitive external-beam radiotherapy and one or multiple doses (35 to 90 mCi per intravenous or intra-arterial infusion) of 125I-labeled monoclonal antibody. The total cumulative dose ranged from 40 to 224 mCi. At 1 year, 60% of patients were alive, and the median survival was 15.6 months. In an updated report of this study that included a total of 180 patients with a minimum follow-up of 5 years, median survival was 13.4 months for those with GBM.111
Another potential target is tenascin, an extracellular protein overexpressed in malignant gliomas but not found in normal tissue. Radiolabeled monoclonal antibodies to tenascin have been evaluated in phase I or II trials showing activity against newly diagnosed and recurrent malignant gliomas.112,113 In a phase II trial by Reardon et al.,112 131I-labeled murine antitenascin monoclonal antibody was injected directly into the surgical resection cavity in 33 patients with untreated malignant glioma. Patients were subsequently treated with external-beam radiotherapy and 1 year of alkylator-based chemotherapy. Even after accounting for prognostic factors, median survival (86.7 weeks) was longer than that of historical controls. Treatment-related toxicities were mild; only 1 patient required reoperation for radionecrosis.
Targeted Therapies
EGFR gene amplification is seen in approximately 40% to 50% of patients with GBM. EGFR is associated with control of cell growth through autocrine and paracrine effects of growth factors. Inhibitors of EGFR tyrosine kinase such as gefitinib and erlotinib and EGFR antibodies have shown activity against GBM in early clinical trials.114,115 However, in a study by Chakravarti et al.,116 EGFR levels as measured by quantitative immunohistochemistry were not of prognostic value for patients with newly diagnosed GBM. Response to gefitinib did not correlate with tumor EGFR status in a study by Uhm et al.117 The presence of an EGFR deletion mutant variant III (EGFRvIII), leading to a constitutively active variant of a key cell survival pathway, and the presence of intact PTEN, a downstream inhibitor of this signaling pathway, have been found to be significantly associated with clinical response to EGFR kinase inhibitors in patients with GBM.118
More recently, immunotherapeutic approaches involving peptide vaccines targeting EGFRvIII have been tested in multicenter phase II trials of newly diagnosed EGFRvIII-expressing GBM following gross total resection and conventional radiotherapy with concurrent temozolomide. Both peptide vaccines rindopepimut (CDX-110) and PEPvIII consist of 13 amino acids unique to EGFRvIII and conjugated to keyhole limpet hemocyanin. In a phase II study of rindopepimut,119 the primary endpoint of progression-free rate at 5.5 months was 66%. At a median follow-up of 29.5 months, overall survival was 21.3 months. In a phase II trial of PEPvIII,120 median progression-free survival and overall survival were 14.2 and 26 months, respectively. In both trials, results compared favorably to historical controls, although such comparisons are troublesome because patients enrolled in these studies were required to have good performance status and gross total resection, both of which are positive prognostic factors. Of interest, 82% of cases in the PEPvIII trial lost EGFRvIII expression at recurrence, suggesting the capacity of the EGFRvIII-targeted vaccine to potentially eliminate EGFRvIII-expression tumor cells in most patients. Similar results have been observed in preclinical models.121 Efforts to test these EGFRvIII vaccination strategies in the phase III setting are ongoing. Further data are needed to define the significance of EGFR mutations and downstream regulators of associated pathways.
Mutations and loss of the PTEN gene are encountered in approximately 70% of patients with GBM. PTEN inhibits signaling through the phosphoinositide 3-kinase and Akt signaling pathway. Loss of PTEN results in loss of effectiveness of EGFR inhibitors, probably due to constitutive signaling through phosphoinositide 3-kinase, which bypasses any upstream EGFR inhibitor effect. Specific inhibitors such as CCI-779 and everolimus are ineffective as single agents. Preclinical experiments suggest that these agents are potential radiosensitizers.122
Neovascularization is a major feature of GBM, and many studies demonstrate that GBMs secrete VEGF in abundance. The supporting endothelium strongly expresses receptors for VEGF. Bevacizumab, an anti-VEGF antibody, is FDA approved for the treatment of recurrent glioblastoma (see later discussion). Multiple phase II studies have demonstrated an impressive radiographic response rate and reduction in peritumoral edema. Other agents, such as enzastaurin, which inhibits VEGF signaling by inhibiting protein kinase cβ2, have been tested with approximately 40% response rates.123
Treatment of Elderly Patients
Approximately one-third of patients with GBM are 65 years or older. Older age has been demonstrated to be an important adverse prognostic factor in GBM. In a population-based survey of 3,298 patients with GBM in Ontario,124 each decade increase in age was associated with a reduction in overall survival, with patients older than 70 years having a median survival of 4 to 5 months. In the updated results from the EORTC/NCIC trial, Stupp et al.105 observed a survival advantage to radiotherapy with concomitant and adjuvant temozolomide compared to radiotherapy alone in all age subgroups, including patients older than 60 years of age.
However, due to concerns over the tolerance of conventional chemoradiotherapy for older patients, particularly those with poor performance status, nonconventional approaches to adjuvant therapy have been tested. A phase III Association des Neuro-Oncologue d’Expression Française (ANOCEF) group trial125 observed improved median survival (6.7 vs. 3.9 months) and progression-free survival but equivalent toxicity with adjuvant radiotherapy to 50 Gy compared to best supportive care in patients 70 years and older with KPS ≥70.
Short-course radiotherapy and single-agent temozolomide have also been investigated. As described previously, Roa et al.126 observed no difference between standard radiotherapy of 60 Gy in 30 fractions or a shorter course of 40 Gy in 15 fractions in GBM patients older than 50 years. A more recent European trial compared overall survival between standard radiotherapy (60 Gy in 30 fractions), short-course radiotherapy (34 Gy in 10 fractions), and single-agent temozolomide in patients 60 years or older.127 Preliminary results have demonstrated inferior median survival with 60-Gy radiotherapy versus temozolomide with subgroup analysis limiting this survival difference only to patients older than age 70. No other survival differences were observed. Toxicities have not yet been reported. Preliminary results from the NOA-08 phase III trial128 demonstrated inferior survival but higher toxicity with 1-week-on/1-week-off temozolomide compared to radiotherapy to 54 to 60 Gy in patients 65 years or older with anaplastic astrocytoma or GBM.
Treatment at Recurrence
Although several therapeutic options have been considered for patients with recurrent GBM, none are curative, and therefore the management goals should be palliative. Hospice referral for palliative care is reasonable for many patients. Palliative debulking may help selected patients by relieving mass effect and probably extends survival by about 4 to 6 months on average. Based on an impressive radiographic response rate in two nonrandomized, phase II clinical trials,129,130 single-agent bevacizumab was approved by the FDA in 2009 for the treatment of recurrent glioblastoma. In a study of 49 glioblastoma patients, Kreisl et al.130 reported objective response rate of 35%, 6-month progression-free survival of 29%, 3.7-month median progression-free survival, and 7.2-month median overall survival. Similarly, Friedman et al.129 reported an objective response rate of 28%, 6-month progression-free survival of 43%, median progression-free survival of 4.2 months, and median overall survival of 9.2 months in a total of 85 patients. Although approved as a single agent, controversy remains on whether bevacizumab should be combined with a cytotoxic agent as with other solid tumors.
Polymer-based local chemotherapy (carmustine wafers) has been tested in a randomized trial that included 222 patients with recurrent glioma (mostly GBM); survival increased from 44% to 64% at 6 months (p = .02) for patients with GBM, and median survival increased from 23 to 31 weeks.46 Systemic chemotherapeutic agents have been tested mostly in the context of clinical trials and have been uniformly disappointing,131 as have targeted agents, with the notable exception of EGFR tyrosine kinase inhibitors in patients with recurrent GBM expressing wild-type PTEN and mutant EGFR.118 Of 37 patients with recurrent GBM treated with EGFR tyrosine kinase inhibitors at UCLA there were 7 responders, whereas 19 had early progression. Coexpression of mutant EGFR and wild-type PTEN had 86% sensitivity, 89% specificity, and a positive predictive value of 75% for response.
Repeat radiotherapy using one of several different methods (including radiosurgery, brachytherapy, GliaSite balloon brachytherapy, and even repeat external-beam radiotherapy) may be considered for carefully selected patients.132 Hypofractionated reirradiation with bevacizumab has shown promising results in small single-institutional reports, with surprisingly limited toxicity, attributed to “vascular stabilization and protection” resulting from bevacizumab, and more investigations of this approach are being considered.133 The hypofractionated approach with temozolomide and bevacizumab has been extended to the newly diagnosed setting, and at the Society of Neuro-oncology 2011 meeting, investigators from the Memorial Sloan-Kettering Cancer Center reported median survival of >21 months in a small cohort of unmethylated MGMT GBM patients.134
Evidence-Based Treatment Summary
1. Maximal surgical resection, although not tested in a prospective trial, is generally fit.
2. Postoperative radiotherapy has been shown to provide a survival advantage in several clinical trials. The typical radiotherapy dose is 60 Gy in 6 weeks; dose escalation strategies have generally failed. Although there is much interest in incorporating advanced imaging in treatment planning and in using newer treatment modalities, their benefits in GBM remain to be demonstrated.
3. Temozolomide, given during and after radiotherapy, provides a significant survival advantage that is greatest in patients with methylation of the promoter region of the MGMT gene.
Anaplastic Glioma
Anaplastic gliomas (WHO grade III gliomas: anaplastic astrocytoma, anaplastic oligoastrocytoma, and anaplastic oligodendroglioma) constitute approximately 25% of high-grade gliomas in adults, generally occurring during young to middle adulthood. Histologically, these tumors have increased cellularity, nuclear atypia, and marked mitotic activity, without necrosis or neovascularization. On imaging, anaplastic gliomas may show enhancement and necrosis similar to GBM, although up to one-third of tumors may not enhance.135
The prognosis for patients with anaplastic glioma is heavily influenced by a number of molecular genetic factors. Based upon traditional histology alone, patients with anaplastic astrocytoma have a median survival of approximately 3 years following diagnosis, although there is clinical and biologic heterogeneity. Prognostic factors include age at diagnosis, mental status, and performance status (see Table 35.4). Patients with histologically defined anaplastic oligodendroglioma, in general, have a better prognosis. However, the prognosis of these patients is better defined by whether or not chromosome changes characterized by loss of heterozygosity of 1p and 19q are present (see later discussion). The prognosis for patients with a mixed tumor, anaplastic oligoastrocytoma, varies depending on the dominant histologic cell type.136
Molecular Genetics
Allelic loss of 1p and 19q is believed to be an early genetic alteration in the transformation and progression of oligodendrogliomas. Combined 1p and 19q deletions have been found in 63% of patients with anaplastic oligodendroglioma and 52% of patients with mixed anaplastic oligoastrocytoma, whereas astrocytic tumors have a low incidence (8% to 11%) of combined 1p and 19q deletions.137 Deletions in 1p and 19p have been associated with longer progression-free survival and chemosensitivity and radiosensitivity.137–138,139
The impact of 1p19q codeletion status on outcome has been confirmed in two recent phase III trials. RTOG 94-02 retrospectively assessed 1p and 19q status in 206 of 289 enrolled patients (71%) with anaplastic oligodendroglioma/oligoastrocytoma randomized to receive chemotherapy with procarbazine, CCNU, and vincristine (PCV) followed by radiotherapy or radiotherapy alone.27 Combined loss of 1p and 19q was present in 43% of patients in the PCV plus radiotherapy arm and 50% in the radiotherapy-alone arm. Combined loss of 1p and 19q resulted in a longer median survival time of >7 years versus 2.8 years (p <.001). There was no effect of tumor genotype on overall survival by treatment. At first analysis, PCV did not appear to improve survival for any patient subgroup. In EORTC 26951, 368 patients with anaplastic oligodendroglioma/oligoastrocytoma were randomized to receive radiotherapy followed by PCV or radiotherapy alone.140 Patients with codeletions of 1p and 19q had significantly longer overall survival irrespective of treatment, and similar to the RTOG trial, the addition of PCV did not result in a better outcome compared to radiotherapy alone. Presence of 1p or 19q loss was found to be the most important predictor of outcome, with a hazard ratio of 0.27, confirming that patients with loss of 1p or 19q represent a unique biologic subset.
In a recent updated analysis of RTOG 9402, the RTOG reported survival benefit from the addition of PCV chemotherapy in the 1p19q codeleted subset.141 The analysis was performed with median follow-up of >11 years. For the entire study population, the median overall survival for patients receiving radiotherapy alone or radiotherapy plus PCV chemotherapy was similar. However, the 126 patients with 1p19q codeleted tumors had much longer median survival times than the 135 patients whose tumors did not carry the 1p19q codeletion: 8.7 versus 2.7 years. Even more impressive, however, was the finding that 1p19q codeletion predicted the benefit from adding chemotherapy to radiotherapy. Patients with 1p19q codeleted tumors who received PCV chemotherapy plus radiotherapy (59 patients) had a median overall survival time of 14.7 years, compared with only 7.3 years for patients with codeleted tumors who received radiotherapy alone (67 patients), therefore establishing 1p19q codeletion as both a prognostic and predictive marker.
Others molecular factors with clinical implications include MGMT promoter methylation and somatic mutations in the isocitrate dehydrogenase 1 (IDH1) gene. MGMT promoter methylation has demonstrated prognostic significance for anaplastic oligodendroglial tumors. However, unlike grade IV astrocytoma tumors, in which MGMT promoter methylation predicts survival benefit from temozolomide added to radiotherapy, MGMT promoter methylation has not shown similar predictive significance for outcome to PCV chemotherapy in anaplastic oligodendroglial tumors.142 Identified through genome-wide mutational analysis of grade IV glioma, IDH1 mutations have been observed in 55% to 80% of grade II and III gliomas.143,144 Although rarely present in primary grade IV gliomas, IDH1 mutations are frequently present in secondary grade IV gliomas that develop from lower-grade tumors, providing a biologic explanation for this clinical categorization.145 Prospective trials of anaplastic gliomas demonstrated IDH1 mutations to be a strong prognostic factor. EORTC 26951 observed IDH1 mutations in 46% of patients and demonstrated prognostic significance, independent of 1p/19q codeletion, in both arms of the trial for both progression-free survival and overall survival.146 A German multicenter phase III trial of up-front radiotherapy versus up-front chemotherapy for newly diagnosed anaplastic gliomas observed IDH1 mutations to be a stronger prognostic factor than 1p19q codeletion or MGMT promoter hypermethylation.145 Of note, however, is that both MGMT promoter hypermethylation and IDH1 mutations are correlated with 1p19q chromosomal loss.
Treatment
The current standard of care for patients with anaplastic gliomas is maximal surgical resection followed by postoperative radiotherapy.147 The radiotherapy target volume and dose are the same as for GBM.
Chemotherapy
Adjuvant chemotherapy added to radiotherapy has been justified on the basis of prospective trials, and a meta-analysis showing a small long-term survival benefit in patients with anaplastic glioma treated with alkylating agents plus radiotherapy.102,147 However, because treatment is associated with significant toxicity and because of the marginal survival benefit, the use of chemotherapy has not been universally adopted. Phase 3 studies are currently enrolling patients to more clearly define the role of chemotherapy for patients with anaplastic astrocytoma, or non-codeleted anaplastic gliomas, whereas for the codeleted subset of anaplastic gliomas, chemotherapy is now considered “standard of care.”
Anaplastic Astrocytoma
Levin et al.148 randomized patients with anaplastic gliomas or GBM to receive radiotherapy with adjuvant BCNU or PCV. The use of PCV was found to be associated with an improved outcome in patients with anaplastic glioma. In contrast, a retrospective review of 432 patients with newly diagnosed anaplastic astrocytoma treated with BCNU or PCV in RTOG studies showed no improvement in survival with chemotherapy.149
A prospective phase III trial by the United Kingdom Medical Research Council randomized 674 patients, of whom 117 (17%) had anaplastic astrocytoma after surgery, to radiotherapy alone or radiotherapy followed by PCV.150 There was no advantage for adjuvant PCV in any subgroup. The median survival of patients with anaplastic astrocytoma, 13 to 15 months, was substantially lower than the median survival of 2 to 3 years reported in previous trials, which has led to debate over the applicability of these results.
Temozolomide has shown activity in patients with recurrent anaplastic astrocytoma. In a phase II trial by Yung et al.,103 162 patients with anaplastic astrocytoma were treated with temozolomide (150 to 200 mg/m2 per day on days 1–5 every 28 days) at first relapse. The 6-month progression-free survival was 46%, and overall survival was 13.6 months. The objective response rate was 35% (complete response 8%, partial response 27%). The agent was well tolerated, with mild to moderate hematologic toxicity in <10% of patients. The results of this trial suggest that temozolomide has antitumor activity with an acceptable safety profile for anaplastic astrocytoma. Although it is controversial, many neuro-oncologists treat anaplastic astrocytoma patients with concurrent temozolomide chemotherapy and radiation therapy followed by adjuvant temozolomide chemotherapy based on the EORTC/NCIC clinical trial in glioblastoma. This practice is being investigated in an ongoing phase III clinical trial of non–1p19q-codeleted anaplastic glioma patients (the CATNON Intergroup trial).
Anaplastic Oligodendroglioma/Oligoastrocytoma
Anaplastic oligodendroglioma and oligoastrocytoma are generally thought of as chemosensitive primarily based on high response rates to PCV in several studies. Two large randomized trials, described earlier, investigated the use of sequential chemoradiotherapy compared to radiotherapy alone with chemotherapy reserved for salvage in patients with anaplastic oligodendroglioma and oligoastrocytoma.27,140 With 11-year follow-up, no difference in survival was found for the entire cohort, but for the codeleted patients, there was a near-doubling of survival, establishing chemoradiotherapy as a standard for this subset.
Because of the significant toxicity associated with PCV, many clinicians now use temozolomide, which is much better tolerated. The optimal number of chemotherapy cycles has not been established. Temozolomide has produced high response rates in patients with anaplastic oligodendroglioma. Chinot et al.151 administered temozolomide to 48 patients with anaplastic oligodendroglioma/oligoastrocytoma who had previously failed PCV chemotherapy. The objective response rate was 43.8% (complete response 16.7%, partial response 27.1%). Grade 3 thrombocytopenia occurred in 6.3% patients. Vogelbaum et al.152 reported the results of RTOG 01-31, a phase II trial in which temozolomide was given preradiotherapy to newly diagnosed patients with anaplastic oligodendroglioma/oligoastrocytoma. In the 27 patients available for review, the objective response rate was 33.3% (complete response 3.7%, partial response 29.6%). The 6-month-progression rate was 10.3%. Toxicity was acceptable. In a retrospective series from Ducray et al.,153 up-front temozolomide for patients older than 70 years with anaplastic oligodendroglioma or anaplastic oligoastrocytoma demonstrated median progression-free survival of 6.9 months and median survival of 12.1 months, with improved outcomes in patients with MGMT methylation. Response to temozolomide has also been shown to be significantly associated with loss of 1p in a small retrospective study.35
In addition, a recent international retrospective study of more than 1,000 adults with anaplastic oligodendroglial tumors observed that combination chemotherapy and radiotherapy was associated with longer time to progression and overall survival than either modality alone.127 In cases without 1p19q codeletion, Lassman et al.154 observed that combination chemotherapy and radiotherapy was associated with longer time to progression and overall survival than either modality alone. In cases with 1p19q codeletion, combination chemotherapy and radiotherapy was associated with longer median time to progression compared to either modality alone, but this difference was not associated with a survival advantage. In addition, longer time to progression was observed with PCV compared to temozolomide in codeleted cases. Therefore, although temozolomide is widely used in lieu of PCV, the data supporting this practice are weak at best and, based on Lassman’s retrospective review, is possibly deleterious.
Radiosensitizers
Prados et al.155 randomized patients with anaplastic astrocytomas to receive conventional radiotherapy with or without bromodeoxyuridine (BrdU) given as an infusion during each week of radiotherapy plus adjuvant PCV. The study was closed before full accrual, based on an interim analysis that predicted no survival advantage for the BrdU arm. In the 190 patients who were eligible for analysis, there was no survival benefit found with the addition of BrdU.
Evidence-Based Treatment Summary
1. Maximal resection, although not tested in a prospective trial, is generally associated with more favorable outcome and is recommended whenever feasible.
2. Postoperative radiotherapy has been shown to provide a survival advantage in several clinical trials. These trials included patients with WHO grade III and IV tumors; no trial for only grade III tumors has been conducted. The standard of care is as for GBM in terms of radiotherapy target volume and dose, typically 60 Gy in 6 weeks.
3. The role of chemotherapy remains undefined for the non-codeleted anaplastic gliomas. The most widely tested agent, BCNU, and combination, with PCV, have no definite survival benefit. Temozolomide is active in recurrent anaplastic astrocytoma and is currently being tested in the up-front setting.
4. Patients with codeletions of 1p and 19q have a more favorable prognosis and respond better to both chemotherapy and radiotherapy, and in this subset, PCV chemoradiotherapy has a proven survival advantage over radiotherapy alone. The exact role of temozolomide has not been defined.
Low-Grade Glioma
Low-grade gliomas are slow-growing tumors that are divided into pilocytic and nonpilocyitc subtypes. They account for 20% and 10% of gliomas and primary intracranial tumors in adults, respectively.
Pilocytic Astrocytoma
Pilocytic astrocytoma, also known as juvenile pilocytic astrocytoma, corresponds to WHO grade I. They are more common in children. On imaging, they are well circumscribed enhancing lesions, often with a cystic component.
Treatment
Pilocytic astrocytomas are more amenable to total resection than other low-grade gliomas. Fenestration of the cyst and resection of the mural nodule are usually curative. In tumors in which the wall of the cyst enhances, cystic degeneration of a larger tumor is more likely, and resection of the entire cyst is necessary. Complete resection of pilocytic astrocytomas is associated with excellent survival, with the majority (>90%) of patients cured of the tumor; no adjuvant therapy is necessary.
Incomplete resection is associated with long-term survival rates of 70% to 80% at 10 years. The benefit of postoperative radiotherapy is unclear. Although the usual recommendation is for close follow-up, there is evidence of improved progression-free survival in this situation,156 and immediate postoperative irradiation may be appropriate in some relatively uncommon cases, depending on the location of the tumor, the extent of residual disease, the feasibility of repeated surgical excision, and availability for follow-up. If radiotherapy is indicated, the dose is typically 50 to 55 Gy (1.8- to 2-Gy fractions).
Evidence-Based Treatment Summary
1. Maximal surgical resection, although not tested in a prospective trial, is associated with more favorable outcome and is recommended whenever feasible.
2. Postoperative radiotherapy may be considered in patients with incompletely resected tumors, based on risk factors for progression and consequences of progression.
3. Chemotherapy does not have an established role in pilocytic astrocytoma in adults.
Nonpilocytic/Diffusely Infiltrating Gliomas
Nonpilocytic or diffusely infiltrating low-grade gliomas are classified as WHO grade II tumors. They may arise from astrocytic, oligodendrocytic, or mixed lineage. Presentation usually occurs in the third or fourth decade of life, and only a small percentage of patients are younger than 19 years or older than 65 years. CT typically demonstrates an ill-defined, diffuse, nonenhancing low-density region, often in the frontal or temporal lobes. Calcifications are commonly seen with oligodendrogliomas. MRI is more sensitive in detecting and defining these lesions, which are hypointense and nonenhancing on T1-weighted images and hyperintense on T2-weighted images. Because these tumors are highly infiltrative, tumor always extends beyond the abnormality observed on imaging.
Histologically, there is increased cellularity compared to normal brain tissue, with mild to moderate nuclear pleomorphism and no evidence of mitotic activity, vascular proliferative changes, or necrosis. Differentiation from reactive gliosis can be difficult. Histologic subtypes are often identified in low-grade astrocytomas. Fibrillary and protoplasmic subtypes convey no specific prognostic information. Gemistocytic subtypes behave in a fashion more consistent with a malignant glioma.
Factors associated with improved outcome include young age, good neurologic status, oligodendroglial subtype, and low proliferation indices. Median survival is approximately 5 years for patients with astrocytoma and approximately 10 years for patients with oligodendroglioma.157 The 5-year survival rate is 37% for patients with astrocytoma, 56% for mixed oligoastrocytoma, and 70% for oligodendroglioma.34 A Ki-67 (MIB-I) index >3% has been shown to correlate with a worse prognosis.158 Malignant transformation portends a poor outcome.
Molecular Diagnosis and Prognosis
Smith et al.137 found loss of 1p and 19q in 44% of 52 patients with oligodendrogliomas, of which 34 (65%) were low grade. Combined loss of 1p and 19q was associated with an improved probability of survival, independent of other factors, such as age. Fallon et al.159 examined 139 tumor samples from 80 patients with primary and recurrent oligodendrogliomas, of which 74% were grade II at initial diagnosis. Combined loss of 1p and 19q occurred in 71% of patients, more commonly in pure oligodendrogliomas (75%) than in mixed oligoastrocytomas (39%). Patients with combined loss of 1p and 19q had an overall median survival of 14.9 years, compared to 4.7 years for those without 1p and 19q deletions.
Okamota et al.160 found a similar frequency of loss of 1p and 19q of 70% in patients with low-grade oligodendrogliomas in a population-based study. In a review of 44 cases of low-grade oligodendrocytic tumors, Sasaki et al.161 considered half of the cases classical oligodendroglioma and the remainder nonclassical oligodendroglioma with more astrocytic features on histopathology. Deletion of 1p was detected in 86% and 27% of these groups, respectively. Response to chemotherapy was assessed at time of recurrence in 13 patients. Of the 11 who responded to chemotherapy, 10 had loss of 1p. Both of the nonresponders were found not to have loss of 1p. A small prospective trial by Hoang-Xuan et al.162 found a similar trend. Loss of 1p with or without loss of 19q, which was detected in 12 of 26 patients with low-grade oligodendrocytic tumors treated with temozolomide, was found to have a significant association with response to chemotherapy (p <.004).
IDH1 mutations may be predictive of a better prognosis in patients with low-grade gliomas, but this marker does not appear to predict response to chemotherapy.163 Promoter methylation of the PTEN gene has been associated with poorer prognosis and a higher likelihood of malignant transformation.164 Other small studies have not shown an association between other molecular findings and chemosensitivity.165,166
Clinical Prognostic Variables
In addition to histology and molecular characteristics, several clinical variables have been found to be of prognostic importance. Pignatti et al.167 performed the most comprehensive of these analyses and constructed a scoring system to identify patients at low and high risk. This trial was based on data from two large European phase III trials for low-grade glioma designed to examine the dose and timing of postoperative radiotherapy, EORTC 22844 and 22845. Cox regression analysis was used to identify prognostic variables from 322 patients from EORTC 22844 and then validated on 288 patients from EORTC 22845. Multivariate analysis showed that age 40 years or older, astrocytoma histology, maximum diameter ≥6 cm, tumor crossing the midline, and presence of neurologic deficits negatively affected survival. A prognostic scoring system was derived: patients with up to two of these factors were considered low risk (median survival, 7.7 years), and patients with three or more were considered high risk (median survival, 3.2 years).
Treatment
Treatment—in particular the timing of intervention—for patients with diffusely infiltrative low-grade tumors remains controversial. The clinical course is variable, with some patients having long survival even without treatment and others suffering from progressive deterioration despite treatment. In general, early intervention is indicated for patients with increasing symptoms, radiographic progression, and high-risk features suggestive of transformation to a higher-grade tumor. Adequate tissue sampling is critical to ensure accurate diagnosis, and maximal surgical resection in this context may be advisable in appropriately selected patients. In younger patients (<40 years) who have undergone complete resection, observation with serial imaging are options. In those who have undergone a subtotal resection or those with high-risk features, postoperative radiotherapy may be recommended, typically 50 Gy in 1.8-Gy fractions. Recent evidence suggests that chemotherapy may have a role, particularly in patients with loss of 1p and 19q. Accrual of patients into well-designed clinical trials is imperative to define optimal treatment, and newer trials are also focusing on the effect of treatment or tumor progression on neurocognitive functioning.
Surgery
Although surgery is considered an integral part of treatment, the goal of surgery and its timing are still debated. In practice, most patients undergo surgery at presentation in order to establish the diagnosis and to determine histology, grade, and molecular characteristics that affect treatment. However, even under the best circumstances, total resection with an adequate margin is rarely achieved due to the diffusely infiltrative nature of these tumors and involvement of eloquent regions.168 Although it is controversial, most studies have found total or subtotal (>90%) resection to be associated with improved outcome.168–170 Moreover, with the advent of imaging to assist in identifying critical areas of the brain, resection may be achieved with less morbidity and mortality than in the past. Proponents of aggressive resection are also supported by studies that suggest that radical surgery results in more accurate histopathologic diagnosis.171 However, surgical resection is unlikely to be curative. In RTOG 98-02, 5-year progression-free survival in 111 good-risk patients, defined as patient age younger than 40 years and gross total tumor resection, was 48%.172 Review of postoperative MR imaging demonstrated crude recurrence rates of 26%, 68%, and 89% for residual disease <1 cm, 1 to 2 cm, and >2 cm, respectively.
Radiotherapy
Three recent phase III trials provide the best evidence with respect to the indications for radiotherapy, as well as the dose (Table 35.5).
Patients considered favorable by the scoring system introduced by Pignatti et al.167 are typically observed postoperatively and given radiotherapy at disease progression or recurrence. This practice is based on the results of a phase III trial by van den Bent et al.173 in EORTC 22845. In this multi-institutional trial, 314 patients with low-grade gliomas were randomized to receive postoperative radiotherapy to 54 Gy in fractions of 1.8 Gy (n = 157) or radiotherapy at progression (n = 157). A significant improvement in median progression-free survival was found with early radiotherapy (5.3 vs. 3.4 years; p <.0001), but there was no difference in median survival (7.4 vs. 7.2 years; p = .872). It is of note that only 65% the patients in the delayed-radiotherapy group received radiotherapy at progression. Malignant transformation occurred in 65% to 72% of patients, with no difference between the two groups. Although seizure control was superior in the early radiotherapy group, adequate data on quality of life were not obtained. The authors concluded that although early radiotherapy may be appropriate in some situations—for example, patients with symptomatic lesions—withholding radiotherapy until tumor progression does not jeopardize survival.
For patients given radiotherapy postoperatively, the dose has been established by two phase III trials. In EORTC 22844, 379 patients were randomized to receive 45 Gy in 5 weeks or 59.4 Gy in 6.6 weeks postoperatively.174 With a median follow-up of 74 months, overall survival (58% vs. 59%) and progression-free survival (47% vs. 50%) were similar in both arms.
In a joint North Central Cancer Treatment Group, RTOG, and ECOG study, 203 patients were randomized to low-dose radiotherapy to 50.4 Gy in 28 fractions (n = 101) or high-dose radiotherapy to 64.8 Gy in 36 fractions.175 There was no significant difference in progression-free survival or overall survival. Survival at 2 and 5 years was 94% and 72%, respectively, with low-dose radiotherapy and 85% and 64%, respectively, with high-dose radiotherapy. Grade 3 to 5 neurotoxicity occurred in 5% of patients in the high-dose cohort and 2.5% of patients in the low-dose cohort.
Consequently, low-dose radiotherapy—50 Gy in 1.8-Gy fractions—is the standard of care for patients with low-grade gliomas. The target volume is local, with a margin of 2 cm beyond changes demonstrated on traditional MRI sequences. Using FLAIR images, which usually show abnormality beyond any enhancing or nonenhancing tumor, a smaller margin of 0.8 to 1 cm may be used.
TABLE 35.5 PHASE III TRIALS OF PATIENTS WITH LOW-GRADE GLIOMA TREATED WITH RADIOTHERAPY
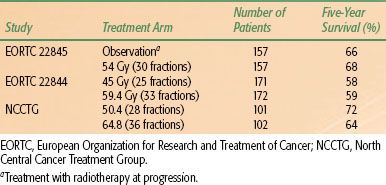
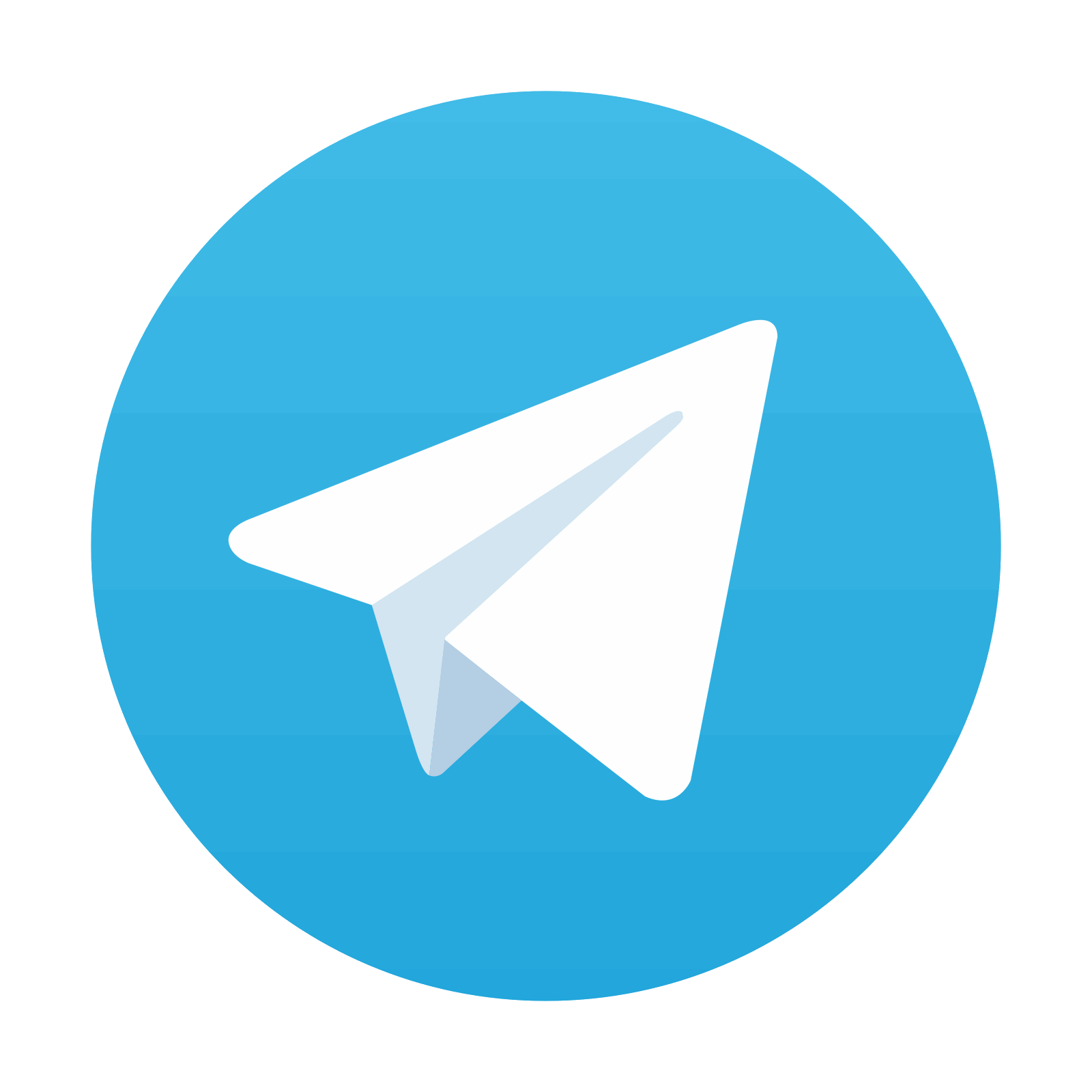