Introduction
Endocrine neoplasms comprise a variety of benign and malignant tumors that arise from the endocrine glands or other neuroendocrine tissues, such as the paraganglia. Most childhood endocrine tumors, typified by papillary thyroid carcinoma, are sporadic and not attributable to an identifiable germline mutation whereas others, epitomized by catecholamine-producing tumors ( Table 15.1 ) and medullary thyroid carcinoma (MTC), are familial and occur within the context of a broader hereditary tumor syndrome. In familial endocrine neoplasms, the mode of inheritance is autosomal dominant and mutations are primarily inactivating mutations causing loss of function in a tumor suppressor gene. Advances in genetic testing and research have led to the ongoing discovery of novel tumor-susceptibility genes, in addition to a better understanding of the underlying pathophysiology of these unique disorders. Knowledge regarding genotype-phenotype relationships continues to evolve, as has clinical practice regarding the age of performing predictive genetic testing, screening for endocrine tumors in an asymptomatic carrier, and the timing of therapeutic intervention. Given the rapidly changing field, it is beneficial for patients with an endocrine tumor to be evaluated in programs with known multidisciplinary expertise. In addition, the results of genetic testing and formal genetic counseling should be fully incorporated into treatment planning and long-term follow-up. This chapter reviews the pathophysiology, diagnosis, and management of pediatric endocrine tumors and the most common genetic syndromes associated with their diagnosis.
Hereditary Syndrome | Gene (Chromosome) | Tumor Type | Clinical Phenotype a | Earliest Age b | Screening Guidelines c |
---|---|---|---|---|---|
Multiple endocrine neoplasia type 2A (MEN2A) | RET (10q11.21) | PHEO/rare PGL & composite PHEO d |
| 8 | Start by age 11 yrs. in codon 634 mutations; by age 16 yrs. in other codons |
Multiple endocrine neoplasia type 2B (MEN2B) | RET (10q11.21) | PHEO/rare PGL |
| 10 | Start by age 11 yrs. in codon 918 and 883 mutations |
Neurofibromatosis type 1 (NF1) | NF1 (17q11.2) | PHEO/rare PGL & composite PHEO d |
| 7 | Screen any NF1 patient with HTN, signs/symptoms of catecholamine excess, or an incidental adrenal or paraaortic mass |
Familial paraganglioma syndrome 1 (PGL1) | SDHD (11q23.1) | PGL (primarily head & neck > thorax/ abdominal/pelvic)/PHEO |
| 5 | Start by age 10 yrs. in patients with paternal inheritance of disease e |
Familial paraganglioma syndrome 3 (PGL3) | SDHC (1q23.3) | PGL (primarily head & neck/ thorax) |
| 13 | Start by age 10 yrs f |
Familial paraganglioma syndrome 4 (PGL4) | SDHB (1p36.13) | PGL (Abdominal/pelvic > head & neck)/PHEO |
| 3 | Start by age 5 yrs g |
Familial paraganglioma syndrome 5 (PGL5) | SDHA (5p15.33) | PGL/PHEO |
| 8 | Start by age 10 yrs h |
von Hippel-Lindau disease (VHL) | VHL (3p25.3) | PHEO/PGL |
| 2 | Start by age 5 yrs |
a Noradrenergic tumors exclusively secrete norepinephrine/normetanephrine, whereas adrenergic tumors secrete both epinephrine and norepinephrine, in addition to their metabolites (metanephrine and normetanephrine). Nonfunctional tumors are parasympathetic tumors that arise in the head and neck.
b Earliest age of diagnosis of PHEO/PGL and reference.
c Data from consensus guidelines and other relevant publications. The age to start annual screening (blood pressure; fractionated plasma or 24-hour urine metanephrines) and the frequency/type of radiologic screening (for SDHx carriers) is not an absolute and should be tailored to patient symptoms, physical examination (blood pressure), biochemical test results, and family preference. Consider testing in young women of childbearing age who plan to become pregnant and in any patient before an elective surgical procedure, assuming they have not been recently screened.
d A composite PHEO is a mixed tumor comprised of PHEO and a developmentally related neurogenic tumor, such as neuroblastoma, ganglioneuroma, or ganglioneuroblastoma.
e There are parent-of-origin effects in PGL1, such that children develop clinical disease only if a mutation is inherited from their father. In children with maternal transmission of an SDHD mutation, routine screening is not needed, although comprehensive testing should be considered at least once, as there are reports of maternally inherited SDHD mutations causing disease.
f The authors also consider head and neck ultrasonography for screening of cervical PGL, in addition to biennial (or less frequent) whole-body magnetic resonance imaging (MRI) as recommended by others.
g Given the higher rate of malignancy, earlier screening is recommended for SDHB mutation carriers. In addition to biochemical screening, the authors consider ultrasound of the abdomen/pelvis starting at age 5 years and biennial whole-body MRI when the patient is old enough to tolerate an MRI without sedation.
h Disease penetrance in young SDHA mutation carriers is extremely low and thus intensive screening during childhood may not be warranted.
Genetic counseling and testing
A diagnosis of an endocrine tumor in a child, especially one that is neuroendocrine in origin, should raise concern for the possibility of an underlying genetic predisposition. Genetic counseling is a process of communication that promotes understanding, decision-making, and coping related to the impact of genetic disease. It should be incorporated into all stages of care, both at diagnosis and during long-term follow-up, because patients’ needs change over time and also because genetic testing and management recommendations are likely to evolve.
Genetic testing, nicely exemplified in multiple endocrine neoplasia (MEN) type 2, is a multistep process that begins with a patient who already has clinical manifestations of disease. In most cases, such as in MEN1 and MEN2A, this is likely to be a parent or another blood relative, but in other hereditable disorders with low disease penetrance, such as the familial paraganglioma (PGL) syndromes, genetic testing of the family may actually begin with the child who is the index case. Genetic testing serves multiple purposes, including confirmation of the genetic cause of an endocrine tumor, identification of asymptomatic patients who are at risk for clinical disease, and guidance regarding clinical management and family planning. When ordering predictive genetic testing, it is important to understand how the results will be used in patient management. In some cases (e.g., MEN2), knowledge of the genotype will lead to a disease-preventing intervention (early thyroidectomy), whereas in other hereditary syndromes, such as MEN1 and von Hippel-Lindau (VHL) disease, it will only lead to an earlier diagnosis.
The process of genetic testing in children is engrained with various ethical, legal, and psychosocial implications. Despite the clear medical benefits afforded from an early diagnosis, genetic testing in children has the potential for harm to the patient and family: alteration of the child’s self-image and of the parents’ perception of the child, modification of the patient’s outlook on life, worry about the potential for genetic discrimination, changes in family relationships, early “medicalization” of an otherwise healthy child, and concerns regarding future reproductive issues. Timely medical benefit to the child should be the primary justification for genetic testing. In all cases where the risks versus benefits of genetic testing are unclear, the provider should respect the decision of the family. There are several online resources regarding genetic counseling and testing, including the National Society of Genetic Counselors ( www.nsgc.org ), the National Cancer Institute’s “The Genetics of Cancer” website ( www.cancer.gov/cancertopics/genetics ), and the internet site GeneReviews ® ( https://www-ncbi-nlm-nih-gov.easyaccess1.lib.cuhk.edu.hk/books/NBK1116/ ).
Pheochromocytoma and paraganglioma
Pheochromocytomas (PHEOs) and PGLs are neuroendocrine tumors (NETs) that arise from neural crest–derived paraganglion cells. PHEO ( Fig. 15.1 ) is the term used for a catecholamine-producing tumor that evolves from chromaffin cells in the adrenal medulla, whereas PGLs ( Fig. 15.2 ) are extraadrenal tumors that arise from both sympathetic and parasympathetic paraganglia located in the autonomic nervous system outside the cerebrospinal axis. Historically, the term PHEO was used interchangeably with PGL, but it is best to maintain a distinction between these two neoplasms because of underlying differences in genetics, clinical presentation, and malignant potential (see Table 15.1 ).
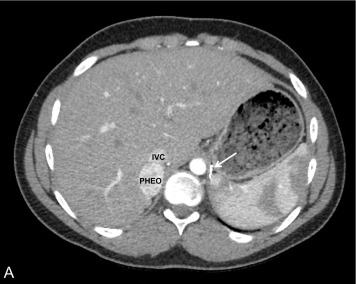
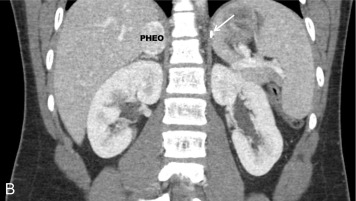
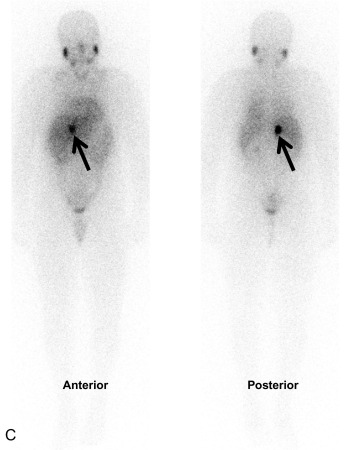
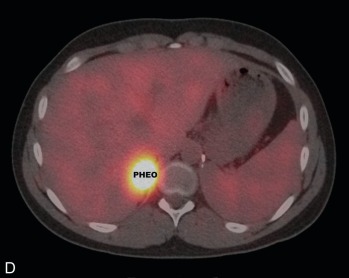
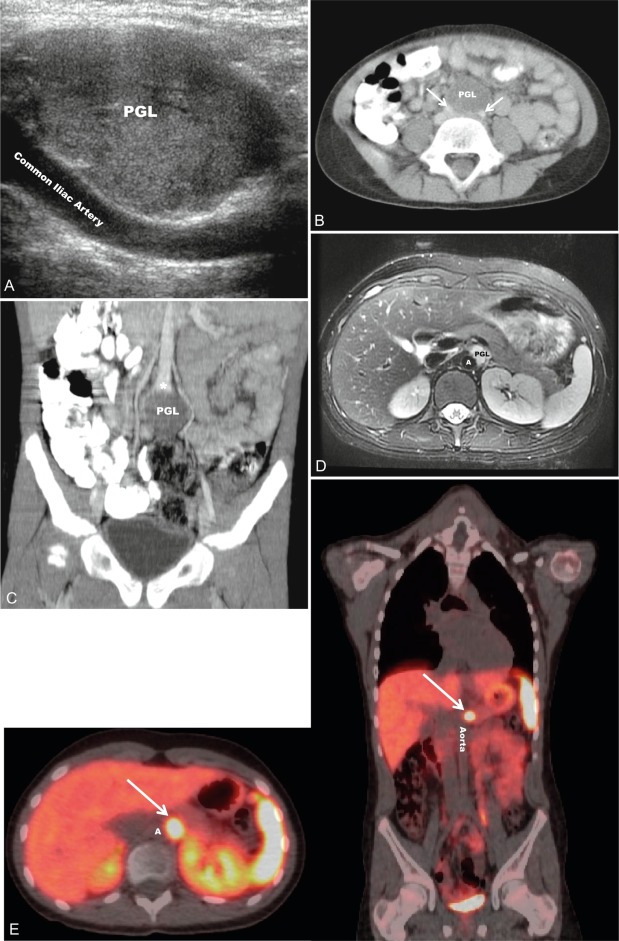
PHEO/PGL are rare tumors with an annual incidence in the United States of 500 to 1600 cases per year. Around 10% of PHEO/PGL are diagnosed during childhood at an average age of 13 years; there is a slight predominance in boys, particularly when diagnosed under the age of 10 years. Less than 2% of children diagnosed with hypertension will harbor a catecholamine-producing neoplasm.
PGL occur in all locations where paraganglia are found (from the skull base to the pelvis) and are either functioning (sympathetic) or nonfunctioning (parasympathetic) neoplasms, depending on the site of origin and underlying pathophysiology (see Table 15.1 ). PGL arising in the head and neck region, which are rarer in children, are almost exclusively nonfunctioning, parasympathetic tumors, whereas most intraabdominal PGL (commonly occurring within the organ of Zuckerkandl [see Fig. 15.2 ] in a periadrenal location, or around the renal hilum ) are sympathetic and thus secretory tumors. The majority of PHEO/PGL diagnosed during childhood are functional and synthesize and secrete catecholamines (dopamine, norepinephrine, and/or epinephrine) and their metabolites (including 3-methoxytyramine, normetanephrine, and metanephrine, respectively) ( Fig. 15.3 ). All functioning PHEO/PGL contain chromaffin tissue, which refers to the brown-black color resulting from the oxidation of catecholamines after staining with chromium salts. Multicentric tumors are common in childhood presentations of PHEO/PGL.
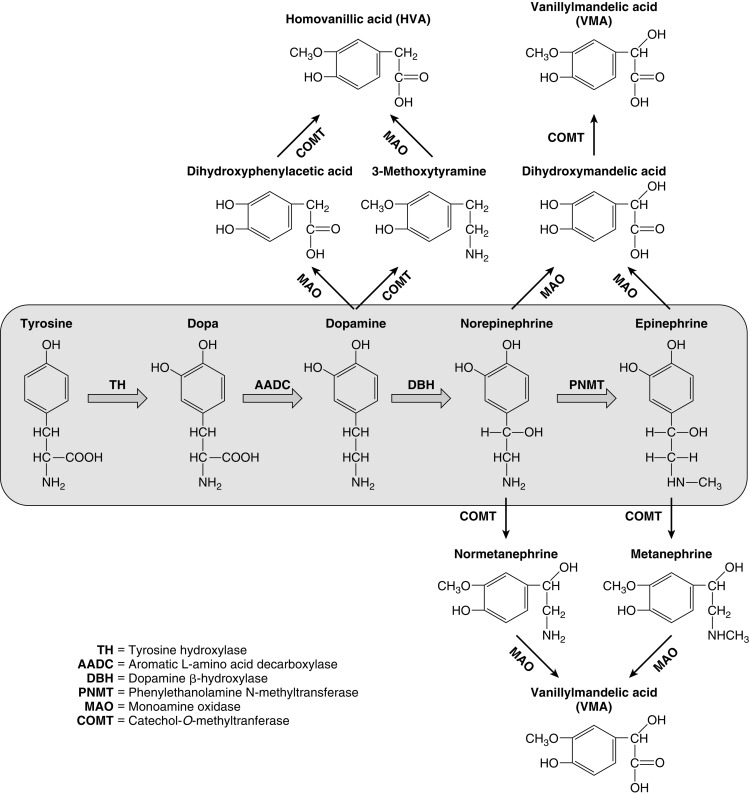
Biosynthesis and Actions of Catecholamines
Dopamine, norepinephrine, and epinephrine (collectively known as catecholamines ) are chemical neurotransmitters and hormones that play important roles in the regulation of numerous physiologic processes and the development of neurologic, psychiatric, endocrine, and cardiovascular diseases. The catecholamines are comprised of a catechol (1,2-dihydroxybenzene) moiety and a side-chain amine group. They are synthesized from the amino acid tyrosine, which is converted to 3,4-dihydroxyphenylalanine (dopa) by the enzyme tyrosine hydroxylase, the rate-limiting step in catecholamine biosynthesis (see Fig. 15.3 ). Subsequent enzymatic decarboxylation and hydroxylation of dopa yields dopamine and norepinephrine, respectively, and norepinephrine is subsequently converted to epinephrine via the cytosolic enzyme phenylethanolamine N-methyltransferase (PNMT).
The catecholamines are synthesized and stored in granules within the adrenal medulla, where they are released via exostosis into the systemic circulation in response to stressful stimuli. Dopamine and norepinephrine are also produced by postganglionic neurons in the sympathetic nervous system. Epinephrine is made only in the adrenal medulla, where it represents the predominant catecholamine (~ 80%) because PNMT expression is dependent upon and regulated by high local concentrations of glucocorticoids (as occurs only in the adrenal medulla, surrounded by the cortisol-synthesizing adrenal cortex, with a distinct concentration gradient towards the adrenal medulla). The effects of catecholamines are terminated via rapid reuptake into nerve terminals by the norepinephrine transporter and via metabolism by two major enzymes: monoamine oxidase (MAO) and catechol- O -methyltransferase (COMT) (see Fig. 15.3 ).
The complex actions of norepinephrine and epinephrine are mediated by the G-protein–coupled α- and β-adrenergic receptors, whereas dopamine binds to a different class of G-protein–coupled dopamine receptors (five distinct receptors [D1–D5] that are divided into two families: D1-like and D2-like) ( Table 15.2 ). The initial classification of adrenergic receptors was based upon epinephrine’s ability to both excite (α-receptor) and inhibit (β-receptor) smooth muscle. Specific agonists and antagonists characterize the adrenergic receptor subtype (α 1 , α 2 , β 1 , β 2 , and β 3 ) and can be used as therapeutic agents. The D2 receptor is the primary dopamine receptor that is targeted for drug therapy.
Receptor Type | Primary Pharmacologic Agonist(s) | Primary Pharmacologic Antagonist(s) | Major Biologic Effects |
---|---|---|---|
α 1 | Phenylephrine, midodrine | Doxazosin prazosin, terazosin, etc. | Vasoconstriction; promotes cardiac growth and structure |
α 2 | Clonidine, methyldopa, tizanidine | Yohimbine | Inhibition of norepinephrine release; vasoconstriction; inhibits adrenal catecholamine release and modulates CNS dopamine neurotransmission |
β 1 | Dobutamine | Atenolol, bisoprolol, esmolol, metoprolol, etc. | Increases heart rate and contractility |
β 2 | Albuterol, levalbuterol, salmeterol, terbutaline, etc. | Propranolol (prototypic β 2 antagonist that is also a β 1 antagonist) and others | Smooth muscle relaxation (arteriolar and venous dilation; relaxation of tracheobronchial muscles) |
β 3 | Mirabegron | Investigational | Metabolic effects in adipose tissue and skeletal muscle |
D1-like (D 1 and D 5 ) | Levodopa | No primary agents | Vasodilation; increased renin secretion; promotes norepinephrine and epinephrine release; various CNS functions |
D2-like (D 2 , D 3 , and D 4 ) | Bromocriptine, cabergoline, pramipexole, ropinirole | Aripiprazole, chlorpromazine, haloperidol, metoclopramide, prochlorperazine, etc. | Decreases prolactin secretion; decreases renin secretion; inhibits norepinephrine and epinephrine release; various CNS functions |
Clinical Presentation
The clinical presentation of pediatric PHEO/PGL is highly variable. Children with these tumors can come to attention because of symptomatic catecholamine hypersecretion, symptoms caused by tumor mass effect (e.g., pain), an incidental radiographic finding, or because of prospective screening in one of the associated hereditary tumor syndromes (see Table 15.1 ). PHEO/PGL may also arise in the setting of cyanotic congenital heart disease. Given their neuroendocrine origin, PHEO/PGL can very rarely cosecrete other hormones that result in a clinical syndrome of ectopic hormone excess, primarily Cushing syndrome, because of overproduction of adrenocorticotropic hormone (ACTH).
The clinical presentation of a functioning PHEO/PGL depends on inherent differences in catecholamine production, as well as individual patient sensitivities to catecholamines. Signs and symptoms of catecholamine excess include: hypertension, which is typically sustained in the majority of pediatric cases; severe headaches; paroxysmal episodes with the classic triad of headaches, palpitations, and diaphoresis (less common in children); orthostatic hypotension and syncope; pallor; tremor; and/or anxiety. PHEO/PGL in children can also cause nonspecific signs and symptoms, such as blurred vision; abdominal pain, constipation, diarrhea and other gastrointestinal symptoms; weight loss; hyperglycemia; polyuria and polydipsia; low-grade fever; and behavioral problems, attention deficit hyperactivity disorder, and/or a decline in school performance. Bladder PGL can present with hematuria and paroxysmal symptoms during micturition.
Complications of catecholamine excess can include hypertensive crisis, cardiomyopathy (takotsubo cardiomyopathy), arrhythmias, pancreatitis, severe constipation and intestinal pseudoobstruction, stroke, seizures, and even multisystem crisis and death. Symptoms of parasympathetic PGL (usually located in the head and neck region) include hearing loss, pulsatile tinnitus, neck mass, and other symptoms of mass effect, such as voice hoarseness, pharyngeal fullness, and dysphagia.
Up to 80% of the pediatric population with PHEO/PGL have a hereditary predisposition syndrome (see Table 15.1 and later section), and a subset of these will be diagnosed only after a tumor is identified during a screening program. Compared with sporadic disease, hereditary PHEO/PGL identified during the course of prospective presymptomatic screening are smaller and less symptomatic (or even asymptomatic) tumors. In all cases of pediatric PHEO/PGL, genetic counseling and testing should be pursued ; identifying a germline mutation early after diagnosis appears to have a positive impact on the management and clinical outcome of patients with heritable disease. The American Association of Cancer Researchers developed guidelines for screening the pediatric population with hereditary PHEO/PGL syndromes, and others have also published screening recommendations in pediatric populations with MEN2 and VHL disease.
Evaluation
Biochemical Diagnosis
The diagnosis of PHEO/PGL has been simplified by advances in the assays used to detect and quantify levels of catecholamines and their metabolites in blood and urine. The measurement of fractionated plasma and/or urine metanephrines (metanephrine and normetanephrine) is the most sensitive test (approaching 100% sensitivity) for the diagnosis of PHEO/PGL and should be the primary diagnostic test ( Fig. 15.4 ).
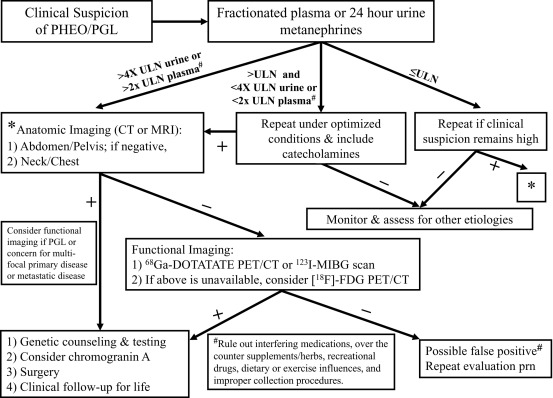
The high sensitivity of metanephrine testing is based upon the fact that there is continuous intratumoral metabolism of catecholamines, a process that occurs independently of catecholamine release, which can occur intermittently or at low rates. An elevation of metanephrines greater than 4-fold above the reference range is associated with almost 100% probability of the presence of a catecholamine-secreting tumor ; however, a PHEO/PGL can still be present with metanephrine levels more than two times the upper limit of normal, especially in the setting of hereditary disease. Any drugs known to interfere with these assays (e.g., acetaminophen, tricyclic antidepressants, phenoxybenzamine, and decongestants, among others ) should be discontinued before testing. Dietary restrictions need not be routinely used but should be considered if the assay used measures only deconjugated normetanephrines or if a dopamine-secreting tumor is suspected. The measurement of the catecholamine metabolites vanillylmandelic acid (VMA) and homovanillic acid (HVA) is no longer recommended for the evaluation of PHEO/PGL. However, testing for HVA and VMA in spot urine samples remains a critical component of the evaluation of neuroblastoma, where these analytes have a high sensitivity and specificity for tumor detection.
In patients with mildly elevated metanephrine levels, in whom a false positive test result is suspected, consideration should be given to measuring these plasma analytes drawn from patients in the supine position, 30 minutes after an indwelling needle or catheter is inserted into the vein. Clonidine suppression and glucagon stimulation tests have been a component of the diagnostic algorithm in adults but are rarely required and have not been validated in the diagnosis of childhood PHEO/PGL.
Catecholamine-producing tumors can be subclassified as being either noradrenergic or adrenergic based upon their pattern of catecholamine release. Noradrenergic PHEO/PGL secrete norepinephrine and normetanephrine, as seen in VHL disease and in tumors associated with the hereditary PGL/PHEO syndromes. Adrenergic tumors secrete both epinephrine and norepinephrine and their metabolites, and these tumors are more commonly PHEO that arise sporadically or within the clinical context of MEN2 or neurofibromatosis type 1 (NF1). This differential secretion of catecholamines is caused by decreased expression of PNMT in noradrenergic tumors secondary to PNMT promoter hypermethylation.
Dopamine-secreting tumors are rare and are typically extraadrenal succinate dehydrogenase ( SDH )x-associated PGLs, as well as head and neck PGLs. The measurement of methoxytyramine, a metabolite of dopamine, (see Fig. 15.3 ) may help to identify more aggressive tumors, particularly in the context of an SDHx mutation, but this test is not widely available. A dopamine-secreting tumor should be considered in normotensive patients identified to have a mass that appears consistent with a PHEO/PGL, in which case dopamine and its metabolites, HVA and methoxytyramine (if available), should be measured. For prospective screening in patients with a known SDHx mutation, total catecholamines should be checked in addition to total metanephrines because of this possibly.
Chromogranin A is a major secretory protein present in the soluble matrix of chromaffin granules that serves as a tumor marker that may correlate with PHEO/PGL size and malignant potential. Chromogranin A also appears to be a useful marker in the biochemically silent, SDHB -related PGL, making it a potentially useful test in the screening of asymptomatic SDHx mutation carriers. However, chromogranin A can be falsely elevated in many circumstances, including concomitant use of proton-pump inhibitors and serotonin reuptake inhibitors, renal failure, and pancreatic disorders, so caution is needed in the interpretation.
Radiographic Studies
Once a diagnosis of catecholamine excess is established from biochemical testing, radiographic studies should be performed to identify the location of the tumor(s) (see Figs. 15.1, 15.2 , and 15.4 ). Initial imaging studies should include anatomic cross-sectional imaging of the abdomen and pelvis (computed tomography [CT] or magnetic resonance imaging [MRI]), followed by imaging of the neck and chest if the initial studies are unrevealing (see Fig. 15.4 ). CT and MRI have similar diagnostic sensitivities, and so the imaging test of choice is best determined by local practices and patient preference. Abdominal ultrasound (US) may also be considered in young children, if local expertise permits (see Fig. 15.2 ). PHEO/PGL are highly vascular (and hence enhancing) neoplasms that commonly contain necrotic, cystic, and/or hemorrhagic areas. On CT, the noncontrast Hounsfield units are almost exclusively greater than 10, and on MRI, PHEO/PGL exhibit a classic hyperintense appearance on T2-weighted images (called the lightbulb sign ) and no loss of signal intensity on in and out of phase imaging (see Fig. 15.2 ).
Nuclear medicine, or functional imaging can be useful in certain circumstances to diagnose PHEO/PGL (especially to confirm the diagnosis in an extraadrenal tumor) or to evaluate the patient for multifocal or metastatic disease, particularly in those patients with risk factors for malignancy and a noradrenergic phenotype. There are several nuclear medicine scans that can be helpful. Metaiodobenzylguanidine (MIBG) is a synthetic compound that bears structural similarity to norepinephrine (except it also has a guanidine side chain that resists metabolism), and it accumulates preferentially in adrenergic tissues, namely because of reuptake via the norepinephrine transporter system. MIBG used for diagnostic purposes is radiolabeled with either I or I, although I MIBG is the agent of choice because of its superior imaging properties and substantially lower radiation dose because it is not a β emitter. I MIBG scanning (see Fig. 15.1 ) can confirm the catecholamine-producing nature of a tumor, localize tumors not seen with cross-sectional imaging, and potentially identify other sites of disease, although its sensitivity is not that high and therefore its use is limited for this reason, unless considering treatment with I-MIBG for metastatic disease. Before I-MIBG scanning, care should be taken to ensure that the patient is not taking medications (e.g., decongestants, calcium-channel blockers, or labetalol) that are known to decrease MIBG uptake, and potassium iodide should be administered to block thyroid uptake of radioactive iodine. Because of the limitations of MIBG testing, the use of other nuclear imaging modalities that incorporate positron-emission tomography (PET) with CT imaging have become more commonplace, especially [18F] fluorodeoxyglucose (FDG) PET/CT and 68Ga-DOTATATE PET/CT (see Fig. 15.2 ). 68Ga-DOTATATE PET/CT has become the functional imaging modality of choice, given its specificity for somatostatin receptors commonly found on NETs, such as PHEO/PGL and its superior sensitivity over FDG-PET/CT and I-MIBG imaging.
Genetic Issues
Knowledge regarding the hereditary causes of catecholamine-producing tumors is rapidly expanding. The syndromes most commonly associated with pediatric PHEO/PGL are VHL disease, followed by the hereditary PGL/PHEO syndromes, particularly those caused by mutations in SDHB (PGL4) and SDHD (PGL1), then NF1 and MEN2 (see Table 15.1 and separate sections later in the chapter). Additional genes associated with the development of PHEO/PGL, but with a much lower prevalence, include fumarate hydratase ( FH ) ; hypoxia-inducible factor 2 alpha ( HIF2A, also known as [aka] EPAS1 ) ; kinesin family member 1B (KIF1B); MEN1 ; MYC associated factor X ( MAX ) ; prolyl hydroxylase domain 1 ( PHD1 , aka EGLN2 ) and 2 ( PHD2 , aka EGLN1 ) ; succinate dehydrogenase complex assembly factor 2 ( SDHAF2 ) ; succinate dehydrogenase complex, subunit A ( SDHA ) ; succinate dehydrogenase complex, subunit C ( SDHC ) ; and transmembrane protein 127 ( TMEM127 ). Recently, even more genes have been described as associated with PHEO/PGL and further research is needed to determine causation. These include BRCA1-associated protein-1 ( BAP1 ) ; malate dehydrogenase 2 ( MDH2 ) ; MER protooncogene, tyrosine kinase ( MERTK ) ; MET protooncogene ( MET ) ; solute carrier family 25 member 11 ( SLC25A11 ) ; and others. Family history, clinical presentation of the patient, and differences in the biochemical phenotype (noradrenergic versus adrenergic) help to prioritize genetic testing, but the availability of next-generation sequencing has led to the development of validated targeted gene panels that are now more commonly used, especially when testing an index case.
Based on genomic analysis, the PHEO/PGL susceptibility genes are now divided into two major clusters: cluster 1 (a pseudohypoxia subtype, subdivided into tricarboxylic acid [TCA] cycle-dependent and VHL/EPAS1 -dependent) and cluster 2 (a kinase signaling subtype). Most pediatric PHEO/PGL will fall into cluster 1 ( SDHx , VHL ) instead of cluster 2 ( NF1 , RET ). PHEO/PGL that arise in the context of VHL or the hereditary PGL syndromes (cluster 1) occur at younger ages and are typically noradrenergic tumors, producing almost exclusively norepinephrine and normetanephrine. Adrenergic tumors (which secrete epinephrine and metanephrine, in addition to norepinephrine and normetanephrine) are seen in cluster 2 (MEN2, NF1) and sporadic cases.
Management
Surgical Therapy
Surgical resection is the mainstay in the treatment of PHEO/PGL. A preoperative biopsy is not indicated and potentially dangerous. The procedure of choice for most PHEO is laparoscopic adrenalectomy, either using transperitoneal or retroperitoneal approaches. Laparotomy should be contemplated in patients with large PHEO and/or a concern for underlying malignancy based upon the clinical presentation, genetic background, or radiographic appearance of the tumor. In the setting of bilateral PHEO or known hereditary PHEO, a cortical-sparing procedure should be performed to minimize the risk of the life long glucocorticoid and mineralocorticoid replacement and the attendant risks of primary adrenal insufficiency. Because it is extremely difficult to preserve a vascularized portion of adrenal cortex sufficient to prevent corticosteroid dependence without also leaving some residual adrenal medulla, there is a risk for recurrent PHEO in the remnant. Recent data suggest that recurrence rates after cortical-sparing surgery are 10% or less. The surgical approach for removal of a PGL depends upon the location of the tumor, but in selected cases of abdominal disease can also be performed laparoscopically. Head and neck PGL can be expectantly monitored, if small, or treated with surgery or radiation.
It is important that the anesthesiologist have experience with the intraoperative management of PHEO/PGL because dysrhythmias can occur and blood pressures can be quite labile. Both intravenous antihypertensive medications (e.g., nitroprusside, phentolamine, esmolol, labetalol, etc.) and vasopressors (e.g., phenylephrine and norepinephrine) should be readily available for intraoperative use. The greatest risk for hypertension occurs during anesthesia induction and manipulation of the tumor, whereas hypotension is most likely to occur after ligation of the adrenal vein, when the abrupt decline in catecholamine concentrations leads to vasodilation. Postoperatively, the patient should be monitored for the two major complications of hypotension and hypoglycemia. In patients who have had a cortical-sparing adrenalectomy in the context of bilateral PHEO resection, stress glucocorticoids should be provided perioperatively and a high-dose cosyntropin stimulation test obtained to determine the need for ongoing adrenal steroid replacement.
Medical Preparation for Surgery
Once the diagnosis of a PHEO/functioning PGL has been confirmed, medical therapy to normalize the blood pressure and mitigate the signs and symptoms of catecholamine excess should be initiated ( Table 15.3 ). If surgery is planned, medical treatment should be taken for at least 10 to 14 days before surgery to minimize the potential complications that may arise from acute catecholamine surges. No universal algorithm exists for the medical management of a PHEO/PGL before surgery. For adults, the Endocrine Society guidelines recommend first-line therapy with an α-adrenergic receptor blocker and second-line therapy with a calcium channel blocker. Limited data exist for children, but small studies suggest these regimens are safe.
Drug Class | Drug | Mechanism of Action | Initial Pediatric Dose |
---|---|---|---|
α-adrenergic receptor blockers | Doxazosin | α 1 -antagonist | 0.5–1 mg daily |
Phenoxybenzamine | α 1 – and α 2 -antagonist | 0.2–0.25 mg/kg/d divided BID (max 10 mg BID) | |
Prazosin | α 1 -antagonist | 0.05–0.1 mg/kg/d divided TID (max 1 mg TID) | |
β-adrenergic receptor blockers | Atenolol | β 1 – antagonist | 0.5–1 mg/kg/d daily or BID (max 50 mg daily) |
Metoprolol | β 1 – antagonist | 1-2 mg/kg/d divided BID (max 50 mg BID) | |
Propranolol | β 1 – and β 2 -antagonist | 1–2 mg/kg/d divided BID or TID (max 80 mg daily) | |
Calcium channel blockers | Amlodipine | Calcium channel blocker | 0.1 mg/kg/d (< 6 years); 2.5–5 mg daily (age ≥ 6 years) |
Nifedipine (sustained release) | Calcium channel blocker | 0.25–0.5 mg/kg/d daily or BID (max 60 mg total daily dose) | |
Inhibitors of catecholamine synthesis | Metyrosine | Tyrosine hydroxylase inhibitor | 125–250 mg QID |
Effective α-receptor blockade improves symptoms, lowers blood pressure, and expands the vascular bed and blood volume. Tachycardia without hypotension is a sign of good alpha blockade and should be treated with addition of a β-adrenergic receptor blocker rather than a dose reduction of the alpha blocker. Beta blockers should not be started without good alpha blockade because of the theoretical risk of reflex hypertension from uncontrolled α-adrenergic receptor activity. Phenoxybenzamine is a noncompetitive α-receptor antagonist that has a long half-life; therefore it may also increase the risk of postoperative hypotension. Selective α1-receptor blockers, such as prazosin and doxazosin, can also be used. Although labetalol and carvedilol, drugs with both α- and β-receptor antagonist activity, sound like attractive options for preoperative blockade, they are not recommended for primary medical treatment because of their lower α-adrenergic receptor blockade relative to their β-antagonist activity. Symptomatic postural hypotension may be seen at the beginning of medical therapy, particularly with large biochemically active tumors, so it is imperative to start at low doses and increase the dose and/or frequency every few days, until the blood pressure is normal for age and height and the patient is minimally orthostatic. Phenoxybenzamine is only supplied as a single dose (10-mg capsule) and so it will need to be compounded by the pharmacy to allow for the administration of the lower doses needed in younger children. Phenoxybenzamine is also an expensive drug, which makes the selective α1-receptor blockers a more attractive option in many cases.
Metyrosine is a competitive inhibitor of tyrosine hydroxylase, the rate-limiting step of catecholamine biosynthesis (see Fig. 15.3 ) and it can also be used as part of the preoperative preparative regimen, although data in children are limited. However, not all centers use metyrosine routinely because of its cost and potential significant side effects (sedation, diarrhea, and extrapyramidal manifestations) and unclear benefit in most cases. A few days before surgery, oral salt loading (either via increased dietary intake or with sodium chloride tablets) is recommended to expand the blood volume to mitigate postoperative hypotension. Some centers also routinely admit patients for intravenous fluids before PHEO/PGL resection, and this can be considered for very symptomatic children with large tumors.
Prognosis and Follow-Up
The long-term prognosis of most children diagnosed with PHEO/PGL is excellent. In all cases, however, there is risk of the development of synchronous and/or metachronous tumors in both adrenal glands, as well as extraadrenal sites. In fact, up to 50% of patients diagnosed with a childhood PHEO/PGL will develop an additional primary tumor in their lifetime (25% at 9 years and 50% at 31 years postdiagnosis), underscoring the need for life long follow-up and appropriate genetic counseling and testing.
Malignancy in PHEO/PGL is defined as the presence of metastases in a site where chromaffin cells and paraganglia are not normally located (primarily bones but also lymph nodes, liver, and/or lungs). In fact, the most recent World Health Organization (WHO) guidelines removed the term malignant PHEO/PGL in favor of metastatic PHEO/PGL. There is no single histologic feature or immunohistochemical profile that is independently able to predict metastatic potential in a resected PHEO/PGL, but histopathologic scoring systems (Phaeochromocytoma and the Adrenal Gland Scaled score and grading system for adrenal PHEO and PGL ) have tried to create predictions for features more frequently noted in metastatic tumors. Unfortunately, these scoring systems are limited by a lot of inter- and intraobserver variability. The most reliable features of an increased risk of metastatic potential include extraadrenal location, size greater than 5 cm, and a germline SDHB mutation. The highest risk for malignancy and death in the pediatric population is in SDHB -related sympathetic PGL, which represents a majority of metastatic tumors.
Based upon data from a British tumor registry, the incidence of malignant disease in children is estimated to be 0.02 per million per year. Approximately 9% to 16% of pediatric PHEO/PGL become metastatic, although some referral centers report a much higher malignancy rate (up to 65%) in pediatric PHEO/PGL, likely reflecting a referral bias. On the other hand, the latency period between diagnosis and confirmation of metastatic disease is 9 years on average ; thus the true rate of malignancy may be higher than previously recognized because it can only be identified during long-term systematic follow-up. Children with metastatic disease typically demonstrate a more indolent clinical course with average overall survival of more than 6 years after the diagnosis of metastatic disease.
Because PHEO/PGL can have unpredictable clinical behavior and because children are at risk for the development of metachronous primary tumors, delayed metastatic disease, and local recurrence, long-term follow-up with biochemical screening and intermittent imaging studies is required for all children with PHEO/PGL. For asymptomatic children with an identified genetic mutation predisposing them to the development of a PHEO/PGL, annual biochemical screening is advised, with the age of initial screening determined by the specific gene mutation (see Table 15.1 ). Furthermore, occasional cross-sectional imaging, typically MRI because of the lack of radiation exposure, is recommended periodically for follow-up of patients at high risk of recurrence or malignant disease (e.g., abdominal PGLs) or at risk for developing a PHEO/PGL that may not be identified on biochemical testing alone (e.g., the hereditary PGL-PHEO syndromes).
Medullary thyroid carcinoma
MTC is a malignant NET that arises from the calcitonin-producing, parafollicular C-cells of the thyroid gland. In childhood, MTC comprises 5% or less of thyroid malignancies and has an annual incidence of 0.3 cases/million/year ; it is the most common thyroid cancer in patients younger than 5 years of age. In the pediatric population, MTC has a fairly equal female: male ratio, unlike the differentiated thyroid carcinomas, which are much more frequent in girls than boys. Pediatric MTC almost always results from an inherited or de novo activating mutation in the rearranged during transfection ( RET ) protooncogene and is diagnosed in the context of either MEN2A or MEN2B, depending on the specific mutation. Because of the close association of MTC with a germline RET deoxyribonucleic acid (DNA) variant, genetic testing is recommended in all cases. Although sporadic nonheritable tumors account for 70% to 75% of adult MTC cases, such tumors are rare in children. Sporadic MTC is primarily associated with somatic (not germline) mutations in RET (specifically the p.M918T mutation) and RAS .
RET is a member of the cadherin superfamily and encodes a receptor tyrosine kinase that has an extracellular binding domain and an intracellular tyrosine kinase domain ( Fig. 15.5 ). The endogenous ligands (which activate RET via a high-affinity ligand-binding coreceptor, GFRα) are members of the glial cell derived neurotrophic factor (GDNF) family, which are involved in the regulation of neural tissue development. The RET protein, therefore plays a crucial role in the development of neural crest-derived cells, the urogenital system, and the central and peripheral nervous systems, notably the enteric nervous system. In MEN2, ligand-independent constitutive activation of the RET receptor tyrosine kinase, either as a dimer (because of mutations in the extracellular cysteine-rich domain; MEN2A) or monomer (because of mutations in the intracellular tyrosine kinase domain; MEN2B), stimulates multiple downstream pathways that promote cell growth, proliferation, survival, and differentiation.
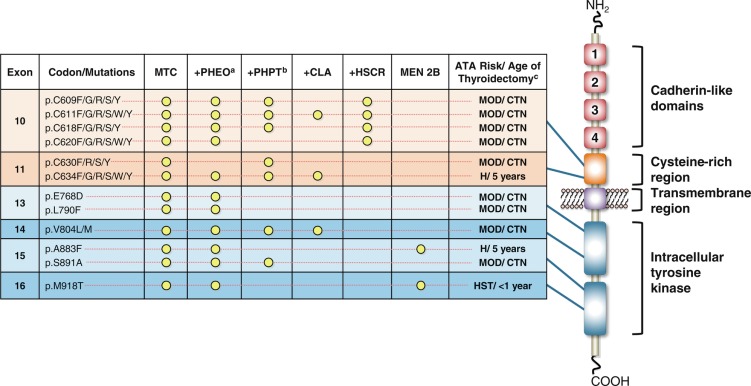
Hereditary MTC is typically multifocal, bilateral, and located in the middle to upper regions of the thyroid lobes ( Fig. 15.6 ), an area where C-cells are the most highly concentrated. Microscopic examination and calcitonin staining of the thyroid will often identify C-cell hyperplasia, the initial stage in an oncologic cascade that leads to the development of microscopic noninvasive MTC and ultimately lymph node and distantly metastatic disease because of frankly invasive carcinoma (see Fig. 15.6 ). Patients with familial MTC have an age-related progression of malignant disease, with lymph node and distant metastases typically occurring years after the onset of C-cell hyperplasia. The cervical and mediastinal lymph node basins are the most common sites of metastatic disease, whereas distant sites for MTC spread include the lungs, liver, and bone/bone marrow.
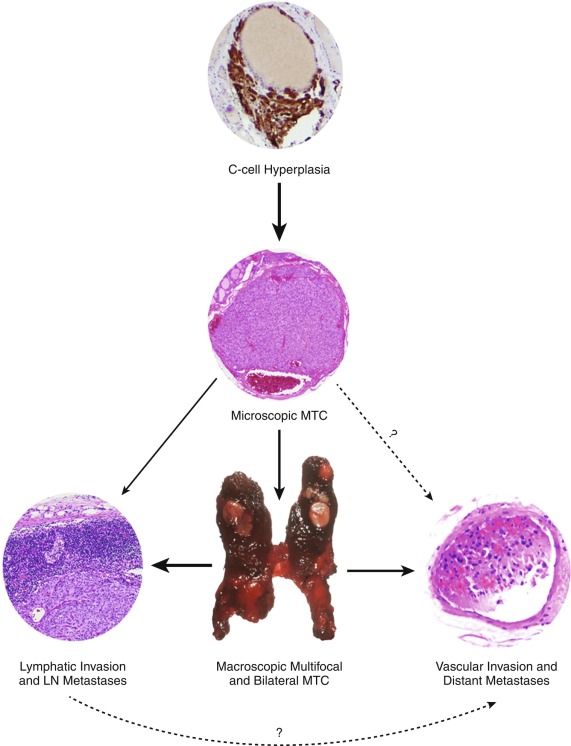
Clinical Presentation
Children with sporadic MTC present similar to other thyroid malignancies, typically with a palpable thyroid nodule and/or cervical lymphadenopathy; diarrhea also can rarely be the initial clinical presentation (primarily attributed to extremely high calcitonin levels). On the other hand, children with hereditary disease because of a germline RET mutation less frequently present with overt clinical disease, except for MTC associated with MEN2B, which is a diagnosis that remains uniformly delayed. Therefore in the current era, the predominant presentation of MTC during childhood is one of the presymptomatic identification of a RET mutation and the ultimate identification of microscopic MTC after early thyroidectomy.
Evaluation and Management
There are several guidelines and review papers available to assist the clinician in the specific evaluation and management of the child with suspected or proven MTC. Similar to other pediatric thyroid malignancies, surgery is the cornerstone of therapy and the only chance for MTC cure. Given the higher surgical complication rates in children and the unique aspects of the medical and surgical care of patients with MTC, treatment should be pursued at tertiary care centers with multidisciplinary, high-volume expertise. Apart from the decision of when to intervene, the thyroid surgeon must incorporate knowledge regarding the patient’s RET genotype and clinical data into the decision-making process to determine the optimal surgical approach. Meticulous and safe removal of all thyroid tissue, including the posterior capsule, is the goal of a prophylactic/early thyroidectomy for the MEN2 patient without clinical MTC. Routine central compartment (level VI) neck dissection is not performed in the setting of a purely prophylactic procedure because lymph node metastases are exceedingly rare in that setting. However, if the operation is for a structurally evident hereditary tumor (especially if the calcitonin level exceeds 40 pg/mL) or for sporadic MTC, total thyroidectomy and a concomitant central neck dissection should be performed. Dissection of the lateral cervical lymph node compartments (levels IIA–V) is generally performed, only in cases where there is clinical evidence of lateral neck involvement, although some centers base this decision on the preoperative calcitonin level.
Fortunately, life-threatening MTC rarely occurs during childhood and primarily only in the clinical context of MEN2B. In advanced MTC, response rates to traditional cytotoxic chemotherapy (dacarbazine, vincristine, and cyclophosphamide) are low. More recently, two targeted molecular therapies (vandetanib and cabozantinib) that inhibit RET and other receptor tyrosine kinases have been approved in adults for the treatment of metastatic MTC. Studies using these drugs in children with MEN2B have suggested comparable efficacy and safety. Novel therapeutics that selectively inhibit the RET kinase in tumors with a RET mutation or fusion are showing promise in clinical trials that commenced in 2017.
Prognosis
Overall, the prognosis of childhood MTC is good, with 5- and 15-year survival rates of 97% and 89%, respectively. In general, positive lymph node status and a higher stage at diagnosis predict lower disease-free survival and higher mortality in MTC. As the tumor stage increases, the risk of both locoregional and distant metastases rises, although lymph node metastases may still occur when the tumor is less than 1 cm in size. Patients with MEN2B have an especially guarded prognosis, with a fifth of patients dying at a median age of 25 years (range < 1–59 years), albeit not always caused by MTC.
Many children who present with clinical MTC already have metastatic disease at diagnosis. Consequently, the majority of cases of childhood MTC not diagnosed before lymph node metastases occur represent incurable yet indolent cancers. The aggressiveness of the clinical course can be predicted by the specific RET mutation (see MEN2 section later), the child’s clinical presentation, and by the use of the serologic tumor markers, calcitonin and carcinoembryonic antigen (CEA). The loss of calcitonin expression, a CEA level out of proportion to calcitonin, and a rapid CEA and/or calcitonin doubling time are all harbingers of an aggressive disease course.
Hereditary endocrine neoplasia syndromes
Carney Complex
First described in 1985, the Carney complex (CNC) is a genetically and clinically heterogeneous familial lentiginosis syndrome with autosomal dominant inheritance. It is characterized by blue nevi and/or spotty skin pigmentation affecting the lips, conjunctiva, and other mucosal surfaces ( Fig. 15.7 ); myxomas of the breast, heart, and skin; endocrine tumors and/or overactivity, classically primary pigmented nodular adrenocortical disease (PPNAD) and pituitary growth hormone/prolactin hypersecretion; and psammomatous melanotic schwannoma, among other clinical manifestations. Two or more distinct genetic loci are associated with CNC: the gene PRKAR1A on chromosome 17q24.2 and an unknown gene on chromosome 2p16. PRKAR1A encodes for the RI-α subunit of protein kinase A, the major mediator of intracellular cyclic adenosine monophosphate (cAMP) signaling. Most PRKAR1A mutations are inactivating point mutations, and about 30% of CNC patients have de novo disease. In patients with CNC, the overall PRKAR1A mutation detection rate is 62%.
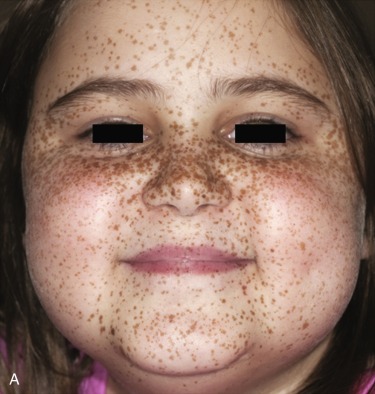
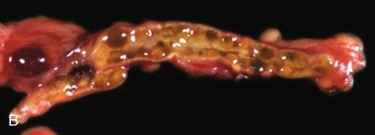
The major endocrine phenotype of the CNC is PPNAD, occurring either alone or with other CNC manifestations, in 60% of cases ; adrenocortical carcinoma also has been rarely reported. PPNAD is a rare form of ACTH-independent hypercortisolism pathologically associated with multiple small (< 1 cm) black or brown nodules (containing lipofuscin) in an otherwise atrophic cortex. (see Fig. 15.7 ). The median age of onset is 34 years (with about 20% of cases occurring during childhood), and there is a female predominance after puberty. The hypercortisolism is typically insidious in its onset, and a pathognomonic finding in PPNAD-related Cushing syndrome is the paradoxical increase in glucocorticoid excretion on the second day of high-dose dexamethasone administration during the Liddle test. Adrenal imaging is of limited value for the confirmation of PPNAD because the lesions are small, but it can suggest diffuse micronodular disease on contrast-enhanced CT. The treatment of symptomatic PPNAD is usually bilateral adrenalectomy, although this may not be necessary in all patients.
Growth-hormone hypersecretion (causing gigantism or acromegaly, depending on the age of onset) is the primary pituitary phenotype of the CNC, occurring in 10% to 12% of CNC patients. Pathologically, this may be associated with an adenoma or hyperplasia. Concomitant hyperprolactinemia is prevalent and is similarly caused by underlying hyperplasia or frank adenomas in rare cases. Subtle abnormalities in growth hormone and prolactin secretion may be identified in up to 75% of patients with CNC. Corticotroph adenomas have also been described in rare cases. Thyroid neoplasia occurs in 25% of CNC patients with most being benign nodules, although differentiated thyroid carcinoma (both papillary and follicular carcinomas) have been identified in 2.5% of cases. Involvement of the thyroid occurs around a median age of 33 years (range 12–57 years) and can be associated with thyrotoxicosis. Males with CNC are at risk for testicular tumors, primarily bilateral large-cell calcifying Sertoli cell tumors (LCCSCT), clinically identified in 33% to 41% of patients. Similar to the Peutz-Jeghers syndrome (see later), gynecomastia and/or testicular enlargement may be a clinical presentation of LCCSCT, which can be malignant in very rare cases.
Once CNC is diagnosed clinically or an asymptomatic child is found to harbor a germline PRKAR1A mutation, prospective monitoring for the development of the tumors and endocrine disorders characteristic of the syndrome can be undertaken using published recommendations for screening.
Familial Isolated Pituitary Adenomas
Hereditary pituitary adenomas can occur as a distinct entity within families who do not have MEN1, and the term familial isolated pituitary adenomas (FIPA) was first proposed in 2006. FIPA is defined by the occurrence of pituitary adenomas in two or more members of the same family with no other clinical manifestations suggestive of MEN1 or CNC. With all hormonal subtypes represented, pituitary tumors occurring within FIPA families present similarly to sporadic pituitary adenomas, and there can be significant intrafamilial clinical heterogeneity.
AIP -Associated Familial Isolated Pituitary Adenomas
In a subset of FIPA kindreds, the disorder was ultimately identified to be caused by germline mutations in the aryl hydrocarbon receptor-interacting protein ( AIP ) gene (chromosome 11q13.2). Mutations in AIP represent only 15% to 30% of FIPA cases and disease penetrance is incomplete, estimated to be less than 30%. FIPA individuals with AIP mutations have tumors that are larger (overwhelmingly macroadenomas) and diagnosed at a much younger age compared with AIP -negative patients. Median age of diagnosis is 16 to 23 years and 50% to 60% of cases present during childhood or adolescence. The vast majority of AIP -mutated adenomas are somatotroph or mammosomatotroph adenomas, which typically present with gigantism in up to 30% of cases (especially males) and are more recalcitrant to the typical therapies prescribed for gigantism/acromegaly. In patients younger than age 18 years presenting with an apparently sporadic pituitary macroadenoma, especially a somatotroph adenoma, 20% may harbor a germline AIP mutation. In a large series of pediatric patients with macroprolactinomas, 9% of tested cases were found to have an AIP mutation, and these children responded similarly well to a dopamine agonist as sporadic cases.
Given the relatively recent characterization of AIP -related FIPA, there are no evidence-based consensus guidelines as to the timing of genetic testing for AIP mutations in sporadic pituitary adenoma cases or for at-risk members of an affected kindred with an AIP mutation. Recommendations for prospective clinical screening in asymptomatic AIP -mutation carriers are also not well established, but some experts have proposed an approach to care that includes educating patients about symptoms of pituitary disease and performing an annual hormonal and auxologic evaluation. Because of the low clinical penetrance of AIP mutations and the high likelihood of functioning pituitary tumors, which most likely would be identified via biochemical testing and review of the growth chart in children, it is unclear if routine screening MRI is indicated.
Hereditary Paraganglioma-Pheochromocytoma Syndromes
Hereditary paraganglioma-pheochromocytoma syndromes (HPS) are characterized primarily by the development of parasympathetic and sympathetic PGL and PHEO. Five autosomal dominantly inherited HPS syndromes (PGL1-5) have been described (see Table 15.1 ) and are caused by germline mutations in discrete genes encoding any of the subunits of the succinate dehydrogenase enzyme gene ( SDHA, SDHB , SDHC , and SDHD ) or a protein necessary for the flavination of SDHA ( SDHAF2 ): PGL1( SDHD ), PGL2 ( SDHAF2 ), PGL3 ( SDHC ), PGL4 ( SDHB ), and PGL5 ( SDHA ).
The SDHx genes comprise the subunits for complex II of the mitochondrial respiratory chain that, when mutated, lead to stabilization of hypoxia inducible factor 1 with a subsequent state of pseudohypoxia. The clinical phenotype somewhat differs among the five syndromes and remains poorly characterized in some cases, such as PGL5 ( SDHA ). As an example, mutations in SDHD primarily cause benign parasympathetic head and neck PGL (also referred to as glomus tumors or chemodectomas ), whereas mutations in SDHB are associated more with abdominal PGL and a higher risk of malignancy (see Table 15.1 and Fig. 15.2 ) In all cases, penetrance of the clinical phenotype is less than 100% and increases with age. Mutations in SDHD (and also SDHAF2 ) demonstrate parent-of-origin effects, with disease occurring only when the mutation is inherited from the father, although recent reports of PHEO/PGL development in individuals with maternal inheritance of an SDHD mutation have been published. Besides PGL and PHEO, SDHx -related tumors include gastrointestinal stromal tumors (GIST), a finding that has also been denoted the Carney-Stratakis syndrome or dyad. Other nonparaganglial neoplasms attributed to an SDHx mutation include clear cell renal cell carcinoma, pituitary adenomas, and other NETs. The association of PHEO/PGL and pituitary adenoma occurring in the context of a germline SDHx mutation has been named the “ 3PAs ,” The optimal screening program for children with HPS (see Table 15.1 ) remains poorly defined but generally includes annual physical examination/blood pressure monitoring, annual plasma or urine metanephrines, and biennial whole body MRI. Similar to presymptomatic screening in other tumor-predisposition syndromes, MRI is the preferred imaging modality in HPS.
Hyperparathyroidism-Jaw Tumor Syndrome
The hyperparathyroidism-jaw tumor (HPT-JT) syndrome is a heritable disorder with incomplete penetrance characterized by parathyroid neoplasia/primary hyperparathyroidism (PHPT) associated with ossifying fibromas of the maxilla and/or mandible. First reported in 2002, CDC73 (chromosome 1q31.2; formerly known as HRPT2 ) is the only known gene in which mutations cause HPT-JT syndrome, and CDC73 mutations are identified in about 60% of HPT-JT syndrome kindreds. PHPT caused by a single benign parathyroid adenoma is the most common clinical manifestation, but parathyroid carcinomas are prevalent, representing 10% to 15% of cases. The youngest age of diagnosis of PHPT has been age 7 years. Ossifying fibromas, also known as cementoossifying fibromas , can be locally aggressive and occur in 30% to 40% of individuals with HPT-JT syndrome ; they are chiefly treated with surgical extirpation. Other clinical manifestations of the HPT-JT syndrome include renal lesions (cysts, hamartomas, and nephroblastoma [Wilms tumor]) and uterine tumors. Prospective clinical monitoring of asymptomatic individuals with a CDC73 mutation is recommended (starting at age 5–10 years) and includes annual biochemistries to assess for PHPT and imaging (panoramic dental x-ray and renal US) every 5 years; female adolescents of reproductive age should get a pelvic US if any abnormal uterine bleeding occurs.
Multiple Endocrine Neoplasia Type 1
MEN1 is an inherited tumor syndrome characterized by glandular hyperplasia and benign or malignant neoplasms occurring in two or more endocrine glands, classically the parathyroids, pituitary, and neuroendocrine pancreas ( Table 15.4 ). MEN1 tumors can be hormonally active or nonfunctioning and multifocality is common, except for pituitary tumors. Patients are also at risk for developing adrenocortical tumors (ACTs), PHEO, extraabdominal NETs (thymus and bronchopulmonary), benign tumors of the skin (angiofibromas, collagenomas, lipomas), central nervous system (CNS) tumors (meningiomas and ependymomas), and/or uterine leiomyomas. Breast cancer risk in women with MEN1 may be heightened.
Tumor | Earliest Age of Diagnosis (Years) With Reference | Age to Begin Screening (Years) | Clinical Signs/Symptoms of Disease | Biochemical b and Imaging Tests Used for Screening Asymptomatic Patients | |
---|---|---|---|---|---|
Pituitary | 5 c , | 5–10 | No symptoms, headaches, visual loss, abnormal linear growth, delayed puberty, galactorrhea, menstrual irregularity | Prolactin, IGF-1 | Pituitary MRI (start at 15 years and then every 3 years) |
Parathyroid | 4 d , 8 e , | 5–10 | No symptoms, nephrolithiasis, polyuria, difficulty concentrating, fatigue, poor appetite, abdominal pain, constipation | Total calcium, intact PTH | No routine imaging indicated |
Thymic Bronchial | 16 15 | 15 | No symptoms, chest/shoulder pain, cough, dyspnea, wheezing, hemoptysis | No routine biochemical testing (unless lesion or clinical syndrome identified) | Chest MRI (start at 15 years and then every 3 years) |
Gastric | NA | NA | No symptoms | No routine biochemical testing | No routine imaging |
Duodenal/pancreatic -Insulinoma | 5 | 5 | Confusion, loss of consciousness, neuroglycopenic symptoms, weakness, seizures | Glucose, insulin | No routine imaging (only once diagnosis made) |
-Gastrinoma | 7 | 15 | Peptic ulcer disease, dyspepsia, abdominal pain, diarrhea | Gastrin f | No routine imaging (will typically not identify duodenal tumors) |
-Nonfunctional | 12 | 15 | No symptoms | Chromogranin A, pancreatic polypeptide g | Abdominal MRI (start at age 15 years and then every 3 years) or endoscopic ultrasound |
-Other functional | NA | NA |
| No routine biochemical testing | No routine imaging |
Adrenocortical | 3 | 15 | Virilization (primary sign), Cushing syndrome, hypertension, abdominal/back pain | No routine biochemical testing (unless lesion or clinical syndrome identified) | Abdominal MRI (done in conjunction with screening for pancreatic NETs) |
a Data from consensus guidelines and other relevant publications. The age to start and the frequency of radiologic screening is not an absolute and should be tailored to patient symptoms and whether or not they already have clinical manifestations of MEN1. In current practice, the authors typically start clinical and biochemical screening at age 5 years and imaging in asymptomatic patients around age 15 years.
b Screening laboratories should always be done after an overnight fast.
c This patient had a mammosomatotroph tumor, cosecreting prolactin and growth hormone.
f Gastrin levels will be elevated in patients taking a proton-pump inhibitor, which should be stopped at least 7 days before testing.
g These tests have a low diagnostic accuracy but, if significantly elevated, should prompt imaging.
The hereditary nature of multiple endocrine adenomas and the link to a monogenic disorder was first proposed by Wermer in 1954. The precise genetic cause of the syndrome was identified and confirmed in 1997. The MEN1 gene is a tumor suppressor gene that encodes a ubiquitous nuclear protein (menin), which plays a role in transcriptional regulation, genome stability, cell division, and proliferation. Most patients with MEN1 inherit the mutation from an affected parent, but about 10% of individuals with MEN1 represent de novo disease; about one-quarter of patients younger than age 21 years will represent index cases.
MEN1 represents a prime example of the “two-hit” mechanism of disease ( Fig. 15.8 ), which was first described by Knudson in hereditary retinoblastoma and is applicable to any disease caused by inactivating mutations of a tumor suppressor gene. An inherited heterozygous germline MEN1 mutation is insufficient to induce tumor formation. Consequently, a somatic mutation (the second hit) in the wild-type MEN1 allele is required to cause disease. This second hit usually deletes the only normally functioning MEN1 gene, leading to a loss of heterozygosity at the MEN1 locus in tumor DNA and attenuating the ordinary constraints by menin on cell growth. More than 90% of tumors from MEN1 patients have loss of heterozygosity caused by a subchromosomal rearrangement or deletion of the entire chromosome; other mechanisms for the second hit include point mutations and small insertions/deletions within the wild-type MEN1 gene. To date, there have been several hundred unique germline mutations of the MEN1 gene characterized, and these occur via multiple mechanisms and are distributed throughout the MEN1 gene without hot spots. Testing by direct DNA sequencing identifies most MEN1 mutations, but approximately 5% to 10% of people with MEN1 do not have a mutation in the coding region or splicing sites of MEN1 . Such patients may represent a phenocopy (see section on MEN4) or they may harbor a mutation in untranslated regions or introns or a large gene deletion, the latter of which requires other technologies, such as multiplex ligation-dependent probe amplification (MLPA) to detect.
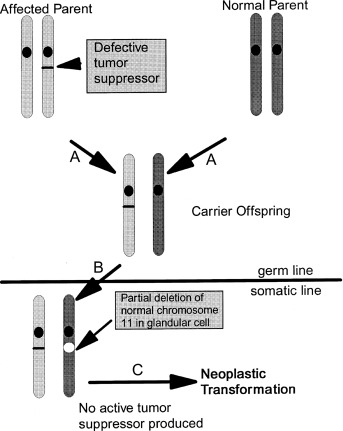
Clinical Presentation and Management
Most children will be diagnosed with MEN1 after the identification of a germline MEN1 mutation, as a result of cascade genetic testing in a known MEN1 family. The clinical presentation of MEN1-related disease is highly variable, even among members of the same kindred, and will depend on the location and functionality of the underlying tumor(s). Not surprisingly, functional tumors typically present 5 to 10 years earlier than nonsecretory neoplasms. Despite the variable expressivity, there is almost complete penetrance of the phenotype, such that MEN1 clinical and biochemical manifestations will generally develop in 80% and more than 98% of patients, respectively, by the fifth decade of life. Penetrance of the clinical phenotype in children with MEN1 has been previously estimated to be less than 1% before age 5 years, 7% by age 10 years, 28% by age 15 years, and 52% by age 20 years ( Fig. 15.9 ). Most of the more recent studies found similar high penetrance in childhood. In two studies, 73% to 78% of patients younger than age 21 years had at least one MEN1 clinical manifestation. In another series, 54.5% of children monitored using a specific screening program developed at least one clinical manifestation of MEN1 by a median age of 22.5 years (range 12–31 years), 83% of whom were clinically asymptomatic. However, another study documented a much lower penetrance (12%) of the MEN1 clinical phenotype before the age of 19 years. In contrast with MEN2, as discussed subsequently, there are no clear genotype-phenotype correlations in MEN1, so a provider cannot rely on the specific mutation or family history to predict the age of onset, severity, or type of clinical manifestation.
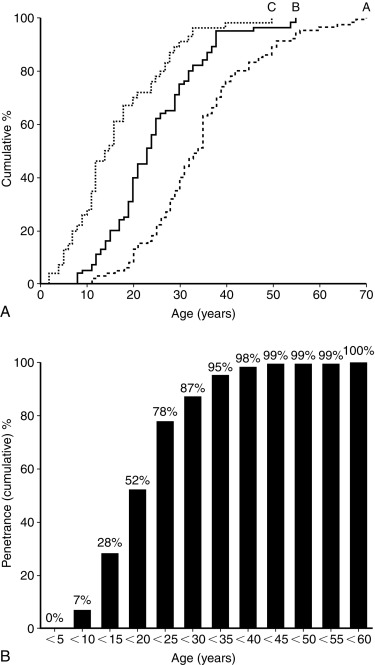
In the absence of treatment, MEN1-associated endocrine tumors are associated with higher mortality (50% probability of death by the age of 50 years.), and the cause of death in 50% to 70% of patients with MEN1 is usually a malignant tumor process or sequela of the disease. Currently, the greatest risk of mortality in MEN1 is chiefly from malignant duodenopancreatic and thymic NETs.
The specific management of each MEN1-associated endocrine neoplasm is generally similar to that for the respective sporadic tumors occurring in patients without MEN1, with notable exceptions for the parathyroid and pancreas (see later). This section will therefore focus more on the unique aspects of these disorders as they relate to MEN1 in children. As with most hereditary tumor syndromes, patients with MEN1 and their families should be managed by a multidisciplinary team consisting of relevant specialists with experience in the management of endocrine tumors.
Primary Hyperparathyroidism
PHPT is the most prevalent and earliest endocrine manifestation of MEN1. It is diagnosed in 75% to 91% of cases of MEN1 patients with clinical manifestations before age 21 years, with or without other MEN1 manifestations; most diagnoses are made after 10 years of age. MEN1 patients have multigland parathyroid hyperplasia rather than single gland adenomas. The majority of children with MEN1-related PHPT are asymptomatic, and nephrolithiasis is the major presenting sign of symptomatic disease.
The diagnosis of PHPT is based on an elevated serum calcium level in the setting of an inappropriately normal or frankly elevated PTH level; at times, the PTH can be elevated with a normal calcium level. Given the multifocal parathyroid hyperplasia in MEN1 patients, preoperative imaging is generally not beneficial. However, in the case of reoperation, localization studies, such as technetium ( 99m Tc) sestamibi scan (typically with concomitant single-photon emission CT [SPECT] imaging), US, contrast-enhanced CT, four-dimensional (4D)-CT, or less commonly MRI can be helpful for surgical planning.
Surgical removal of the hyperfunctioning parathyroid glands is the definitive treatment of PHPT in MEN1 patients, but the surgical approach remains controversial. Subtotal (3.5 gland) parathyroidectomy with transcervical thymectomy or total (four gland) parathyroidectomy with transcervical thymectomy and parathyroid autotransplantation to the nondominant forearm are the primary options for surgical therapy. Resection of less than three glands results in the highest risk of persistent and recurrent disease (odds ratio [OR], 3.11; 95% confidence interval [CI] = 2.00–4.84). Although the risk for persistent or recurrent HPT is not significantly different between a subtotal versus total procedure, the risk for permanent hypoparathyroidism is significantly lower with subtotal parathyroidectomy, which makes this the preferred approach. However, some studies have shown equivalent outcomes between the two procedures in young patients. In patients operated for PHPT before age 21 years, 67% remain normocalcemic at last follow-up, whereas 22% have persistent hypercalcemia and 11% have hypoparathyroidism. At the initial surgery, concomitant transcervical thymectomy is recommended to remove any potential ectopic parathyroid glands and to prophylactically remove the bulk of the thymus, which is at risk for the development of a thymic NET.
Surgical indications for asymptomatic PHPT in children with MEN1 remain poorly developed, and the risk of permanent hypoparathyroidism must be balanced against the risks of PHPT. In addition to the calcium level, factors, such as surgical experience, patient or parent preference, and availability of long-term monitoring of calcium levels, should be considered in the timing of surgery. In select MEN1 patients with PHPT for whom surgery is not feasible, medical treatment with the calcimimetic agent, cinacalcet, can be considered.
Pituitary Adenomas
Pituitary tumors are the second most common clinical manifestation in children, occurring in 29% to 55% of MEN1 patients with penetrance of the MEN1 phenotype younger than 21 years of age, a little over half of whom will be symptomatic. Diagnosis before age 10 years is unlikely. Similar to adults, prolactinomas are the most prevalent, although all subtypes of pituitary adenomas have been reported.
In MEN1, there does seem to be a higher prevalence of pituitary adenomas in females, a higher rate of invasiveness and macroadenomas (especially in males), and more pluripotent tumors. Hypersecreting MEN1-related pituitary tumors may be more resistant to standard medical therapies, although other data suggest that medical treatment is equally effective compared with the general population. There is no increased risk of death from having an MEN1-associated pituitary tumor, although one case of pituitary carcinoma occurring in the context of MEN1 has been published.
Duodenopancreatic Neuroendocrine Tumors
NETs that arise from the neuroendocrine cells of the duodenum and pancreas represent the third most common clinical manifestation of MEN1 in children, reported to be present in 9% to 42% of all patients with MEN1 manifestations occurring before age 21 years. These gastrointestinal (GI) NETs can often be multifocal in the setting of MEN1. Gastrinomas primarily occur in the duodenum (within the so-called gastrinoma triangle ), whereas other hormonally active tumors arise from the pancreatic islet cells at various locations throughout the pancreas. Furthermore, pancreatic NETs can ectopically secrete growth hormone–releasing hormone and ACTH. Nonfunctioning pancreatic NETs by definition are not associated with clinical symptoms from hormonal hypersecretion and thus are diagnosed via routine imaging. Such tumors can overproduce clinically silent hormones, such as pancreatic polypeptide and chromogranin A, although these serologic markers have a low diagnostic accuracy.
Because NETs can be small and grow slowly, localization can be challenging and often multiple techniques may need to be used. Guidelines recommend multiphasic CT, MRI, and/or endoscopic ultrasound (EUS) for the evaluation of pancreatic NETs. EUS affords the highest diagnostic sensitivity, especially with smaller tumors, but this improved sensitivity may not change management because small tumors less than 2 cm in size are typically monitored in the absence of symptomatic hormonal hypersecretion. NETs generally express somatostatin receptors. Thus somatostatin receptor scintigraphy, especially Ga-DOTATATE PET/CT, also plays an important role in the management of pancreatic NETs, especially in the identification of metastatic disease. FDG-PET is not routinely recommended because of the low proliferation rate of these neoplasms, but poorly differentiated tumors can be localized with FDG-PET. A diagnosis of a malignant NET would be extraordinarily rare in childhood. Guidelines regarding the diagnosis and management of NETs are available.
Insulinoma
Insulinomas are the most frequent functioning pancreatic NET in children with MEN1, having been reported to account for 54% to 78% of pancreatic NETs in large published series and diagnosed in 12% to 25% of all patients with MEN1 manifestations that occur at younger than age 21 years. Patients present with hypoglycemic symptoms that improve with glucose intake, thus fulfilling Whipple’s triad. Two main symptoms attributed to hypoglycemia include loss of consciousness or coma (55%) and weakness (45%), associated with seizures in some cases. The gold standard diagnostic test is a 72-hour fast. The diagnosis is established if the serum glucose is less than 55 mg/dL with a concomitant insulin of 3 μU/mL or higher by immunochemiluminescent assay (ICMA), c-peptide of 0.2 nmol/L or higher, and no detectable oral hypoglycemic in the blood. One-third of insulinoma patients will develop hypoglycemia at 12 hours, 80% at 24 hours, 90% at 48 hours, and 100% by 72 hours. Because medical treatment with frequent carbohydrate meals, diazoxide, and/or somatostatin analogs is not always successful, surgery is considered the standard of care. In contrast with other functioning NETs, insulinomas have a lower malignant potential.
Gastrinoma
Gastrinomas occur primarily in the duodenum and are usually multiple, small (< 1 cm) tumors that result in elevated gastric acid production and recurrent peptic ulcer disease, referred to as the Zollinger-Ellison syndrome ( ZES ). Other symptoms can include diarrhea and steatorrhea. They are the most common functional tumor in adults with MEN1. Exceedingly rare during childhood and occurring in 0% to 7% of patients (0% to 22% of pancreatic NETs) in series that include children, gastrinomas are usually diagnosed during the second decade of life in pediatric patients.
The diagnosis is established by an increased serum fasting gastrin concentration (often ⩾ 1000 pg/mL) and elevated basal gastric acid secretion (gastric pH < 2). Patients with borderline hypergastrinemia should be considered for the secretin stimulation test; after a 2-U/kg intravenous bolus, a rise in gastrin of 120 pg/mL or more from baseline affords the highest diagnostic sensitivity and specificity. False positive elevations of gastrin can occur because of use of proton-pump inhibitors or antacids, conditions of autoimmune chronic atrophic gastritis with achlorhydria, and/or chronic renal insufficiency. Patients should therefore be off proton-pump inhibitors for at least 1 week before testing, but this may not be possible in highly symptomatic patients. Hypercalcemia can also stimulate gastrin secretion.
Medical therapy with a potent oral proton-pump inhibitor is the primary treatment of gastrinoma in MEN1 patients. Some patients may also require the addition of an H 2 receptor antagonist. The role of surgery in treating MEN1 patients with gastrinoma is controversial. Tumors are generally multiple and in the duodenum, so cure is less likely, unless extensive surgery is undertaken. If surgery is performed, both duodenal inspection and lymph node dissection are required. For pancreatic gastrinomas or lesions larger than 2 cm, surgery should be considered to reduce the risk of lymph node or hepatic metastasis.
Other Functional Pancreatic Neuroendocrine Tumors
Glucagonomas secrete glucagon and have not been previously described in children with MEN1, outside of a single case of an apparently silent glucagonoma. The classic glucagonoma syndrome is characterized by a skin rash in the groin and extremities (called necrolytic migratory erythema ) in 70% of patients, mild diabetes mellitus in 87%, weight loss in almost all patients, stomatitis, and anemia.
VIPomas, which also have not been reported during childhood, are very rare tumors that secrete vasoactive intestinal peptide (VIP) and are associated with the Verner-Morrison syndrome of watery diarrhea, hypokalemia, and achlorhydria (i.e., pancreatic cholera).
Nonfunctioning Pancreatic Neuroendocrine Tumors
In children with MEN1, nonfunctioning pancreatic NETs represent the second most common NET arising in the duodenum/pancreas (40% of pancreatic NETs; 9% of all cases) and are identified via imaging alone. With improved screening methods, they have been identified in 42% of adolescents subjected to testing and represented 75% of pancreatic NETs, and so their true penetrance may be underestimated in older studies that primarily include functional, and thus symptomatic, tumors. Epidemiologic studies have shown that 27% of patients with nonfunctioning pancreatic NETs measuring 2.1 to 3.0 cm have metastasis compared with 11% of those 2 cm or less. More importantly, nonfunctioning tumors are now understood to confer an increased risk of death in MEN1 patients, comparable with thymic NETs and functioning pancreatic NETs, except insulinomas. Although controversies remain as to the indications for surgical therapy, general consensus is that tumors 2 cm (or larger) or tumors that demonstrate a relatively rapid rate of growth should be considered for resection.
Other Clinical Manifestations
Thymic, Bronchial and Gastric Neuroendocrine Tumors
In MEN1, NETs also occur outside of the duodenum and pancreas and can be found in the thymus, bronchopulmonary system, and stomach. Historically referred to as carcinoid tumors , the preferred term is NET . The diagnosis of such tumors almost universally occurs in adulthood, but one case of a malignant thymic NET has been reported in a 16-year-old MEN1 patient, and a bronchial NET has been found in a 15-year-old girl.
Thymic NETs are more prevalent in smokers and males (male/female ratio 20:1), whereas bronchial NETs are more prevalent in women (male/female ratio 1:4). Bronchial NETs behave more indolently compared with thymic tumors, which impart a significant increased risk for death in MEN1 patients. Thymic NETs can still develop despite previous prophylactic transcervical thymectomy, presumably because the entire thymus is not removed via a cervical approach, underscoring the importance of continuing prospective screening even if thymectomy has already been performed. Thymic and bronchial NETs can ectopically secrete growth hormone–releasing hormone and ACTH.
Gastric NETs are well-differentiated tumors with malignant potential that arise from the enterochromaffin-like cells of the stomach mucosa. Type II gastric NETs are those that occur in MEN1, developing secondary to chronic hypergastrinemia in the setting of gastrinoma/ZES. Given the rarity of gastrinoma in childhood, type II gastric NETs have not been reported in a pediatric patient.
Adrenocortical Tumors
Unilateral and bilateral ACTs are reported in 10% to 26% of MEN1 patients, with a mean age at diagnosis of an adrenal lesion of around 45 years of age ; in children with clinical MEN1 younger than age 21 years, the prevalence ranges from 0% to 4%. Adrenocortical hyperplasia, adenomas, and adrenocortical carcinoma are the types of adrenal lesions seen; PHEO has also been reported in adults with MEN1. Most ACTs are nonfunctioning, and functioning tumors primarily present with hypersecretion of aldosterone or cortisol, although hyperandrogenism/virilization is seen in children. In the large MEN1 series published by Goudet et al. and in the study by Gatta-Cherifi and colleagues, all cases of ACT arising in childhood before age 20 years presented with hyperandrogenism and were classified as malignant.
Cutaneous Manifestations
Often underappreciated as a component of the MEN1 phenotype, skin manifestations ( Fig. 15.10 ) include angiofibromas, collagenomas, and lipomas. More commonly identified in older MEN1 patients, the dermatologic manifestations can also be found in children, even as an initial manifestation of disease. Angiofibromas are benign tumors of blood vessels and connective tissue, similar to those seen in tuberous sclerosis. They present as pink to tan, dome-shaped papules that occur on the face, especially the nose. Collagenomas are well-defined, skin-colored cutaneous papules that have a predilection for the neck and trunk. The prevalence rates of angiofibromas, collagenomas, and lipomas in MEN1 patients are 64% to 88%, 63% to 72%, and 3% to 34%, respectively. In general, MEN1-related skin lesions do not require treatment, although lipomas can grow quite large and may need to be resected because of local symptoms.
