Pharmacology and Mode of Action of Heparin and Glycosaminoglycans
Meyer Michel Samama
Jeanine M. Walenga
Evi Kalodiki
Jawed Fareed
Heparin belongs to a class of linear acidic polysaccharides known as glycosaminoglycans (GAGs). Unfractionated heparin (UFH) came into clinical use in the 1930s, making extracorporeal circulation and cardiovascular surgery possible. Despite its discovery almost 100 years ago, heparin is still a life-saving compound, crucial for procedures such as treatment of deep venous thrombosis (DVT), pulmonary embolism (PE), and acute coronary syndrome (ACS), as well as for anticoagulation in surgery, interventional cardiology, and hemodialysis.
Low molecular weight heparins (LMWHs) are depolymerized heparin derivatives developed in the 1980s, with pharmacologic profiles distinct from UFH.1 Other heparin-related GAGs (heparinoids) of clinical interest include heparan sulfate, dermatan sulfate, chondroitin sulfate, and heparinoids.
There have been major developments recently for new anticoagulant drugs including advancements in new oral anticoagulants. However, heparins continue to be widely used as a clinical anticoagulant.
UNFRACTIONATED HEPARIN
History of the Discovery of Heparin
Jay McLean2 discovered heparin in 1916 by serendipity. He was at that time a medical student in the physiology laboratory of William Henry Howell at Johns Hopkins University Hospital in Baltimore, working on dog liver in search of a procoagulant substance, not an anticoagulant.3, 4, 5
The first publication was authored by McLean2 without the name of Howell, who was skeptical that a real anticoagulant had been discovered. Yet Howell made McLean fight the rest of his life for recognition that the great discovery of heparin was in fact McLean’s.
Howell6 gave the term heparin (Greek for liver “hepar”) to the anticoagulant material discovered by McLean. Finally in 1963, 6 years after McLean’s death, the New York Academy of Medicine presented to Johns Hopkins a plaque in recognition of McLean’s work.4, 7
Several researchers worked on the purification of heparin including Charles Best.8 The story of heparin also includes a history of the discovery of its primary mechanism of anticoagulant activity through binding to the endogenous protease inhibitor antithrombin (AT),9, 10 as outlined (see also Chapter 18):
1895: Discovery of thrombin inhibition by AT11
1916: Discovery of heparin (McLean)2
1935: Heparin active only in the presence of heparin cofactor (Brinkhous)9
1968: Isolation of AT (Abildgaard)10
1973: Procedure to obtain large amounts of AT (Rosenberg)14
1976: AT-binding site separation of active and inactive heparin15
Chemistry of Heparin
Heparin is a heterogeneous natural polysaccharide (FIGURE 105.1), the complexity of which makes its study of pharmacologic characteristics difficult.19, 20 UFH is a biologic product isolated from mammalian tissues, most commonly porcine intestinal mucosa or bovine lung. Heparin is synthesized in these tissues by mast cells as part of a high molecular weight (MW) proteoglycan (MW 750,000-1,000,000 Dalton [Da]), which consists of a center core protein composed of 20 to 25 residues each of glycine and serine.21 There are 15 carbohydrate chains22 (MW 40,000 to 300,000 Da) attached to the core peptide via a galactosyl-galactosyl-xylosyl trisaccharide sequence.23, 24, 25 These chains are polymers of disaccharide repeats that are highly sulfated and therefore negative in charge. Polysaccharide chain elongation and a series of modification reactions of the polymer occur in the final synthesis step.26, 27
The GAGs attached to the proteoglycan central core protein include chondroitin sulfate, heparan sulfate, and heparin.28 Chondroitin sulfate is composed of repeats of glucuronic acid and d-acetyl galactosamine with sulfate groups in the 4 or 6 position of the galactosamine. Its sulfate/carboxylate ratio is equal to 1.0; its sulfur content is ≥5%. Dermatan sulfate is a copolymer composed of repeated iduronic acid and d-galactosamine. It has anti-factor IIa anticoagulant activity.
Heparan sulfate consists of repeats of glucuronic or iduronic acid and N-acetylglucosamine. Total sulfate content and sulfate/carbohydrate ratios differ between GAG types, which play a role in the biologic processes of tissue physiology,29 particularly cell adhesion, cell migration, and cell differentiation, as well as in coagulation, fibrinolysis, and inflammation.28
Four enzymatic modifications of the polysaccharide backbone occur following its synthesis. The majority of the N-acetyl groups on the glucosamine residues are removed,30 and the N-deacetylated glucosamines are subsequently sulfated. In the next step, d-glucuronic acid residues are epimerized to l-iduronic acid units by uronosyl C-5 epimerase.31, 32 During the epimerization process, most iduronic acids are 2-O sulfated.
Finally, 3-O and 6-O sulfate groups are added onto the glucosamine units. N-sulfation allows more efficient O-sulfation to occur.22
Finally, 3-O and 6-O sulfate groups are added onto the glucosamine units. N-sulfation allows more efficient O-sulfation to occur.22
![]() FIGURE 105.1 Characteristics of UFH and LMWHs (Source Material = Porcine Intestinal Mucosa Heparin). |
Heparin consists of a mixture of GAG chains that are made of disaccharide subunits and possess a reducing end and a nonreducing end (FIGURE 105.2). The heparin backbone is composed of alternating sugar units of α-d-glucosamine (N-sulfated, or N-acetylated, O-sulfated) and O-sulfated uronic acids (α-liduronic acid or β-d-glucuronic acid).33 It is the linear pattern of the disaccharide units and the placement of specific disaccharide units within a certain chain length that impart the various and heterogeneous biologic activities of heparin. The most notable illustration of the heterogeneous sulfation pattern is the presence of a particular 3-O sulfate group required for specific interaction of heparin with AT.34 This 3-O sulfate group is present on only a small percentage of heparin chains.
The mean MW of UFH is around 12,000 to 15,000 Da, with individual polysaccharide heparin chains varying in MW from 3,000 to 30,000 Da (FIGURE 105.1).19, 35
Models for Evaluation of Anticoagulant Activity of Heparin
The Wessler model of human serum-induced thrombosis (1959), based on the teachings of Virchow,36 was a commonly used model to determine whether heparin fractions and LMWHs possessed an anticoagulant effect. In this model, an intravenous (IV) serum injection is followed by ligation of an isolated segment of the jugular vein.37 After a 10- or 20-minute period, a full red cell-fibrin thrombus forms in controls, but clot formation is inhibited in the presence of an anticoagulant. Variations of this thrombosis model utilize thromboplastin, activated prothrombin complex concentrate,
or factor Xa as the thrombogenic stimulus.38 Vena cava ligation using a steel coil, collagen, or kaolin has also been used.39 These methods are reproducible and allow for the evaluation of the antithrombotic activity of pharmaceutical preparations.40, 41
or factor Xa as the thrombogenic stimulus.38 Vena cava ligation using a steel coil, collagen, or kaolin has also been used.39 These methods are reproducible and allow for the evaluation of the antithrombotic activity of pharmaceutical preparations.40, 41
Investigations of the anticoagulant activity of heparin and LMWHs in the arterial system were largely performed in a model of vessel wall damage-induced thrombosis using chemical damage (FeCl3), mechanical crushing, or electrical stimulation.42, 43 The Folts model using stenosis and compression injury of the right common carotid artery in the rabbit was another popular model.44, 45
Mechanisms of the Anticoagulant Action of Heparin
The anticoagulant effect of heparin is primarily attributed to inhibition of the serine protease coagulation factors mediated through heparin binding to these plasma proteins.46 The most important anticoagulant activities are the inhibition of the coagulation factors thrombin (factor IIa) and factor Xa by heparin bound to AT.
Heparin has a polycomponent anticoagulant mechanism inhib iting numerous coagulation factors, inhibiting platelet function, and enhancing the antithrombotic functions of vascular endothelium as well as fibrinolysis. In addition, by inhibiting thrombin, heparin affects thrombin-mediated coagulation mechanisms involving factor V, factor VIII, protein C, and thrombin activatable fibrinolytic inhibitor (TAFI). It also releases tissue factor pathway inhibitor (TFPI),1, 47 inhibits the release of P-selectin (an adhesion molecule that in the presence of tissue factor and microparticles modulates the interaction between thrombosis and inflammation),48, 49 impairs vascular barrier properties, and attenuates nitric oxide-mediated vasodilatation during dynamic changes in blood flow.50
Antithrombin
The primary mechanism of heparin is through association with AT, a 55,000-Da single-chain glycoprotein, with a plasma concentration of 2 to 3 µM.51, 52, 53, 54 Heparin binding produces an allosteric modification of AT, which promotes stable complexes, exposing the arginine-385 residue of AT, allowing for interaction with the serine-active site of coagulation enzymes.14, 54, 55, 56
In the presence of heparin, inhibition of coagulation is accelerated 300 to 2,000-fold more than by AT alone.14, 57, 58, 59 Some of the higher MW heparin chains possess two binding sites for AT (FIGURE 105.3).60 The longer heparin chains (i.e., chains of >18 saccharide units; >5,400 Da) bind both AT and the activated coagulation factor, whereas the shorter heparin chains (e.g., pentasaccharide; 1,727 Da) bind only AT.61, 62, 63 Single-site binding of short heparin chains to AT is sufficient to inhibit factor Xa (anti-FXa activity), whereas a second binding site on the higher MW heparin chains is required for the binding and inhibition of thrombin (anti-FIIa activity), FXIIa, FIXa, kallikrein, etc. Thrombin is 10-fold more sensitive to inhibition than factor Xa.
Structure-activity studies have identified a unique minimum saccharide sequence that binds with high affinity to AT (FIGURE 105.2), causing inhibition of FXa but not thrombin.41 Heparin chains that lack this particular pentasaccharide sequence have minimal anticoagulant activity.34
Heparin-bound AT weakly inhibits coagulation factors IXa, XIa, and XIIa, as well as the enzymes trypsin, plasmin, and kallikrein,61, 64 and requires high concentrations of AT (˜5 units). A weak inhibitory activity of this complex toward factor VIIa has been described, but the mechanism is unknown.65
Heparin Cofactor II
Heparin cofactor II (HCII) is a second plasma inhibitor (MW 60,000 Da) that binds heparin and inhibits thrombin by forming a 1:1 stoichiometric complex with the enzyme. Thrombin interacts with the active site of HCII on the C-terminus and forms a covalent bond.64 The reaction of HCII and heparin is charge and chain length dependent, but independent of the pentasaccharide sequence.18
Whereas the activation of AT is dependent upon a specific sequence in the heparin chain, HCII can be activated by a wide variety of agents, including heparin, heparan sulfate, dermatan sulfate, pentosan polysulfate, and dextran sulfate.66, 67, 68 Agents with relatively little sulfation such as chondroitin 4-O- or 6-O-sulfate, keratan sulfate, and hyaluronic acid do not activate HCII.69 High and low AT affinity fractions of heparin equally activate HCII if charge density is equal.70
Tissue Factor Pathway Inhibitor
TFPI (MW 42,000 Da) is a coagulation protease inhibitor synthesized in the vascular endothelium,71, 72 the largest pool of which is bound to the endothelial cell surface.47, 73, 74 Also found in plasma and platelets,75 TFPI has three Kunitz domains linked in tandem between a negatively charged amino terminus and a positively charged carboxy terminus.76 TFPI simultaneously binds to and inhibits the factor VIIa-tissue factor complex and FXa via two of its Kunitz domains.
Heparin displaces TFPI bound to endogenous GAGs on the surface of the endothelium.77, 78, 79, 80, 81 Repeated heparin administration releases TFPI showing no diminishing releasability with TFPI levels reaching 2- to 10-fold over baseline.77 LMWHs have a variable effect on TFPI release.82 Neutralization of heparin by protamine sulfate results in a dramatic decrease in the plasma TFPI level.83, 84 The anticoagulant activity of TFPI can be detected by the prothrombin time (PT), the activated partial thromboplastin time (aPTT), and clot-based anti-FXa assays.85, 86, 87
Other Anticoagulation Properties of Heparin
Platelets
Heparin is a weak platelet agonist, but is also a potent inhibitor of platelet activation induced by thrombin, the LMWHs less so.88 A weak or no response occurs with very low MW heparins (<3,000 Da), having almost no reactivity on ADP, collagen, epinephrine, and thrombin-induced platelet aggregation. Heparin also binds to and inhibits von Willebrand factor
(vWF), the clinical implication being a reduction of thrombotic risk in general and control of platelet-endothelium interactions in specific.89 The weaker antiplatelet effect of LMWHs also is associated with a lower bleeding risk, along with a lesser antithrombotic effect than for UFH. The role of low affinity material which does not contain the binding site of AT is not well documented.90, 91, 92, 93
(vWF), the clinical implication being a reduction of thrombotic risk in general and control of platelet-endothelium interactions in specific.89 The weaker antiplatelet effect of LMWHs also is associated with a lower bleeding risk, along with a lesser antithrombotic effect than for UFH. The role of low affinity material which does not contain the binding site of AT is not well documented.90, 91, 92, 93
Fibrinolysis
A weak profibrinolytic effect has been described,94 but this was not clearly related to the MW of heparin.95, 96 Heparin regulates tissue plasminogen activator and plasminogen activator inhibitor release from endothelial cells, and the anti-FIIa activity of heparin induces a reduced activation of TAfithat facilitates fibrinolysis.97, 98 An increase in the porosity of the fibrin network has been demonstrated.99 At comparable anti-FXa in vitro concentrations, LMWHs exhibit different profibrinolytic activities.100 The clinical significance of these results remains to be established.
Nonanticoagulant Properties of Heparin
In addition to the heterogeneous mixture of saccharide chains, heparin also contains three functional groups that occupy multiple sites on its individual saccharide units: -OSO3–, -NHSO3–, and -COO–.19, 28 This structural diversity induces a diverse range of nonanticoagulant properties. Only 20% to 30% of GAG components produce anticoagulant activity by binding AT, the other 70% to 80% exhibit multiple biologic actions that are only partially understood.29, 101
Heparin-binding Proteins
Heparin-binding proteins can be divided into 1) proteins that enhance the anticoagulant activity such as AT and HCII found in blood and released by heparin such as TFPI and lipase and 2) proteins that inhibit the anticoagulant activity of heparin such as PF4, histidine-rich glycoprotein (HRG), protamine, and vitronectin.28, 104, 105, 106, 107 Some of these interactions are dependent on the MW of the heparin molecules, while others require calcium ions.106
There are different types of heparin binding.22, 107, 108 The first type relates to a specific saccharide sequence of heparin, present on only one-third of the heparin chains, the second is covalent bonding, and the third depends on charge density and chain length.
Histidine-rich Glycoprotein
HRG belongs to the group of proteins that neutralize heparin anticoagulant activity.109, 110 It has an MW of 75,000 Da, has a plasma concentration of approximately 100 mg/L, and binds to heparin and LMWHs. However, only the long-chain fragments of heparin have both anti-FXa and anti-FIIa activities completely neutralized.106, 111 The antiheparin action of HRG requires calcium.109
Vitronectin
Vitronectin, also called complement S protein, is present in the plasma (about 400 mg/L) and in the extracellular matrix. Binding of vitronectin to UFH or LMWHs is associated with neutralization of the anticoagulant activity, but this antiheparin effect is of minor importance.112
Vascular Cells
The direct interaction of endothelial cells with heparin depends on the MW of the heparin.113 Once attached, heparin molecules lose their anticoagulant effect and are internalized. Heparin also binds to smooth muscle cells, which could play a role in atherosclerosis.114, 115 LMWHs and pentasaccharide have the same beneficial activity against atherosclerosis.116 Heparin interacts with endothelial cell growth factors, which results in an enhanced mitogenic activity at the vascular cell lining.117
Platelet Factor 4
Heparin bound to PF4 produces an immunogenic response that can lead to the potential life-threatening disorder of heparin-induced thrombocytopenia (HIT)118, 119, 120, 121 (Chapter 108).
Lipases
Lipoprotein lipase and hepatic lipase are two critical enzymes in lipid and lipoprotein metabolism.122, 123 They are released after an IV injection of heparin or LMWH. Repeated injections of heparin or LMWH do not exhaust the release.124 Elevated levels cause a lipid-clearing lipolytic effect on the blood that is less for LMWHs than for heparin.125 Lipase release is associated with an anti-FXa activity that is not neutralized by protamine or PF4.126, 127
Anti-inflammatory Effect
Heparin has anti-inflammatory actions, for example, in inflammatory bowel disease128, 129 and ulcerative colitis.130 Removal of the 2-0, 3-0 sulfate groups of heparin reduces its anticoagulant activity without impacting its anti-inflammatory effect.131 Heparin inhibits the release of P-selectin from platelets and endothelial cells and also binds to P-selectin and L-selectin and CD11b/CD18 expressed on leukocytes.132 Heparin also binds to components of the complement cascade, reducing activation, a process that may account for the reduced early phase of the asthmatic reaction with inhaled heparin, and for protection of the heart from ischemic reperfusion injury.133, 134, 135 Heparin binding to cytokines inhibits the recruitment of neutrophils and reduces proinflammatory cytokine levels, thereby producing immunomodulating and anti-inflammatory actions.134, 135, 136
Antineoplastic Effect
A link between improved survival and heparin treatment was suggested by Lebeau137 in cancer patients receiving heparin or LMWH for the prevention or treatment of venous thromboembolism (VTE),137 an observation that launched fundamental research and clinical trials.138, 139, 140, 141 In both animal studies and clinical trials, heparin has an antiproliferative property, whereby it inhibits cell growth, cell adhesion, cellular microparticle formation, smooth muscle cell proliferation, and tumor growth.133, 142, 143, 144, 145, 146, 147 Fondaparinux is inactive in these respects.138, 139 Heparin has been postulated to have an antimetastatic effect due to a decrease in neoangiogenesis, possibly mediated by binding to vascular endothelial growth factors, cytokines, and adhesion molecules. Heparin could inhibit metastasis by interference with P- and L-selectin-moderated cell-cell interactions. Other mechanisms have been attributed to the modulation of the angiogenic activity of fibroblast growth factor by heparin140, 141, 142, 143, 144, 145, 146, 147, 148 or by nonanticoagulant components that inhibit cancer growth.149
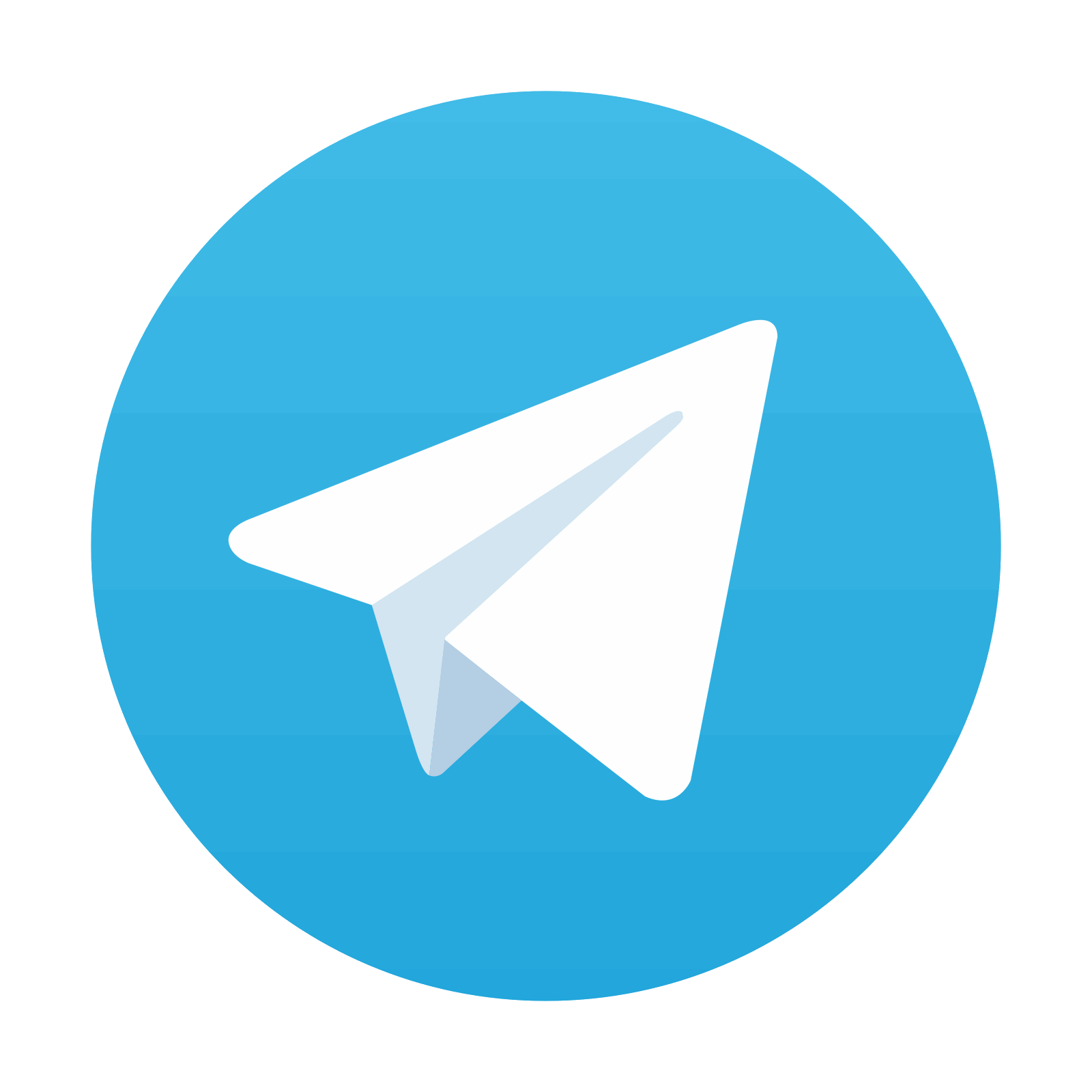
Stay updated, free articles. Join our Telegram channel

Full access? Get Clinical Tree
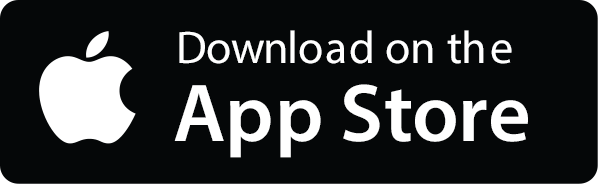
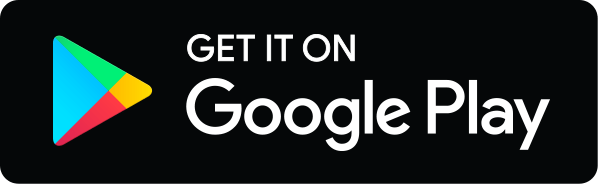
