Summary of Key Points
- •
Patient selection is crucial to ensure treatment selection and optimal outcome.
- •
The performance status, described by, for example, the Karnofsky or the Eastern Cooperative Oncology Group (ECOG) score, is the most important prognostic parameter.
- •
The benefit of concurrent chemotherapy and radiotherapy has only been demonstrated in patients with an ECOG score of 0–1.
- •
Patients with a bad performance status (ECOG 3 or even 4) with important local symptoms, for example, pain, obstruction, or superior venous cava syndrome, may still benefit from palliative radiotherapy.
- •
A poor pulmonary function either because of the presence of tumor or due to chronic lung disease is not a contraindication to high-dose radiotherapy.
- •
Comorbidities significantly impair the long-term survival of lung cancer patients, but are not necessarily a contraindication for high-dose radiotherapy; for example, patients with extensive emphysema show less pulmonary damage after stereotactic ablative radiotherapy.
- •
Interstitial lung disease and autoimmune disorders such as systemic lupus erythematosus and scleroderma have been associated with enhanced intrinsic radiosensitivity of normal tissues and, therefore, a higher risk of serious toxicity resulting from radiotherapy.
- •
Older and/or frail patients have a higher risk for important side effects, but should not necessarily be treated with palliative intent.
- •
Even in elderly patients with thoroughly staged stage III nonsmall cell lung cancer, 5-year survival rates of 15% to 20% are consistently reported with radiotherapy alone.
- •
Continued smoking during curative-intent radiotherapy reduces local tumor control and survival; smoking cessation is therefore essential.
- •
Adequate calorie and protein intake should be ensured.
- •
Physical activity should be encouraged; it also reduces fatigue.
Patient selection is of central importance in the management of lung cancer as it ensures that each patient receives the optimal treatment. However, in order to achieve this objective, clinically relevant parameters that are reproducible and quantifiable should be identified. A highly accurate prognostic model (or, even better, a predictive model) that has been validated on the basis of external data sets or, ideally, randomized studies should be the ultimate goal. However, no such model is currently available. An international task force failed to identify high-quality data for selecting patients for radical radiotherapy. Nevertheless, knowledge regarding these criteria is increasing and is needed in daily practice. In this review, we will discuss the most relevant patient and tumor-related factors that may influence the selection of patients for potentially curative radiotherapy.
Patient-Related Factors
Patient-related factors are often associated with overall survival, quality of life, and response to radiation. These factors (including, but not limited to, age, gender, race, performance status, weight loss, baseline pulmonary function, comorbidities, and smoking status) should be taken into consideration when the decision regarding high-dose radiotherapy is made.
Performance Status
Performance status, a measure of general well-being and activities of daily life, is one of the most important factors associated with outcome for patients with cancer. Various systems are used to evaluate performance status. The most generally used measures are the Karnofsky score and the Zubrod score (also known as the World Health Organization or ECOG score). The Karnofsky score, named after David A. Karnofsky, ranges from 100 to 0, with 100 indicating “perfect” health and 0 indicating death. The Zubrod score, named after C. Gordon Zubrod, ranges from 0 to 5, with 0 denoting “perfect” health and 5 denoting death. Translation between the Zubrod and Karnofsky scales was validated in a large sample of patients with lung cancer. A Zubrod score of 0 or 1 corresponds with a Karnofsky score of 80–100, a Zubrod score of 2 corresponds with a Karnofsky score of 60–70, and a Zubrod score of 3 or 4 corresponds with a Karnofsky score of 10–50.
In general, a poor performance status is not a contraindication to radiotherapy. However, the value of definitive radiotherapy for a patient with a poor performance status may be limited, as survival times are often shorter for these patients. The benefit of radiotherapy in terms of survival time should be weighed against the risk of treatment toxicity and the time needed to complete the definitive course of treatment. Similar to chemotherapy, radiotherapy may offer notable benefit to selected patients with a poor performance status. Radiotherapy is therefore recommended for this population. The regimen of radiotherapy and its combination with other therapy should be individualized for each patient to achieve a maximum therapeutic effect. Meanwhile, palliative radiotherapy often can be used to improve the quality of life for patients with poor performance status and, therefore, should be recommended for those with advanced disease in whom the tumor is causing clinical symptoms or syndromes. For example, a patient with an ECOG score of 3 or 4 as a result of superior venous cava syndrome, obstructive lung disease, or chest pain may have substantial improvement in quality of life after a short course of palliative radiotherapy. Patient selection for radiotherapy should thus be individualized on the basis of a balanced consideration of both the potential benefits and the potential side effects of such treatment.
Lung Function
Patients with lung cancer often present with poor baseline lung function because of the presence of a tumor or a chronic lung condition. Although it is clear that a patient who has poor lung function caused by a local tumor would benefit from radiotherapy, the impaired baseline lung condition from noncancer reasons can often make high-dose radiotherapy challenging. Traditionally, definitive radiotherapy has been considered to be contraindicated for patients with poor lung function. For example, some Radiation Therapy Oncology Group (RTOG) studies, such as RTOG 9311, have excluded patients with a forced expiratory volume in 1 second (FEV 1 ) of less than 0.85 L or 0.75 L from treatment with high-dose radiation. Other studies, such as RTOG 0617 and RTOG 1106, allow such treatment only for patients with an FEV 1 of 1.3 L or more. However, baseline lung function has not consistently been shown to be a risk factor for radiation-induced lung toxicity after conventionally fractionated three-dimensional conformal radiotherapy or hypofractionated stereotactic ablative radiotherapy (SABR). Moreover, the results of pulmonary function tests often are not changed remarkably after modern conformal radiotherapy. Modern dose-escalation studies such as that from the University of Michigan did not limit lung function for very high-dose radiation. In a study of 47 patients, the incidence of lung toxicity had no significant correlation with the results of pulmonary function tests after concurrent chemotherapy and radiotherapy. In a study of 438 patients, FEV 1 along with other patient-related factors seemed to be more important than dosimetric factors for predicting radiation pneumonitis. In a study of 260 patients, the addition of FEV 1 and age to the mean lung dose (MLD) slightly improved the predictability of clinically important radiation-induced lung toxicity. Similar to the SABR series, the study showed that patients with higher baseline lung function tests had significantly more clinical lung toxicity.
In summary, pulmonary function should be considered on an individual basis by balancing the improvement in lung function related to tumor shrinkage with the reduction in lung function related to radiotherapy. In the modern era, poor pulmonary function should not be considered a contraindication to definitive radiotherapy.
Comorbidities
Serious comorbidities are very common in patients with lung cancer and can severely affect outcomes. Long-term tobacco consumption is associated with chronic obstructive pulmonary disease (COPD), ischemic heart disease, cerebrovascular disease, and peripheral vascular disease. Furthermore, other tobacco-related cancers, including head and neck cancers, may be diagnosed before, after, or synchronously with lung cancer, thereby complicating the management of the lung cancer, the other cancer, or both. Luchtenborg et al. studied 3152 patients with nonsmall cell lung cancer (NSCLC) who had surgical resection and reported that serious comorbidity caused a decrement in survival equivalent to a single increment in stage grouping. Comorbidities also reduce the tolerability of chemotherapy to patients with NSCLC. High-dose radiotherapy is poorly tolerated by patients with limited cardiorespiratory reserve due to heart or interstitial lung disease, who are at risk of severe dyspnea or even death if they have insufficient reserve to tolerate impaired organ function after treatment. Smith et al. reported that for patients with NSCLC who were managed with curative-intent radiotherapy, a worse score on the Charlson Comorbidity Index correlated with inferior overall survival but not cause-specific survival. Paradoxically, the scarred lungs of patients with severe COPD may be less likely to be affected by severe radiation pneumonitis after SABR. SABR should not be withheld from patients solely because of COPD.
Although the risks of curative radiotherapy can be difficult to estimate for individual patients, it is usually possible to make a reasonable estimate of the consequences related to loss of a substantial proportion of residual lung or heart function. Apart from general comorbidities such as heart disease, several specific conditions may exacerbate the toxicity of radiotherapy.
Autoimmune disorders such as systemic lupus erythematosus and scleroderma have been associated with enhanced intrinsic radiosensitivity of normal tissues and, therefore, a higher risk of serious toxicity resulting from radiotherapy.
Age and Frailty
Age and frailty are obviously two separate entities: the former is expressed as an objective, trivial number, and the latter is derived from the Latin word fragilis , which means “fragile” (i.e., weak). Frailty clearly increases with age, but a young individual can be frail as well. In most geriatric literature, frailty has been defined as either a threshold beyond which the functional reserve of a person is critically reduced and the tolerance of stress is negligible or as a progressive reduction of functional reserve due to a progressive accumulation of deficits. Thus functional reserve should be measured objectively and used as a prognostic indicator of the survival of the patient and/or the tolerance of the treatment.
Many authors have shown that the older population is a very heterogeneous group in terms of physical, biologic, emotional, and cognitive functions. Nevertheless, increased age is associated with comorbidities as well as higher rates of hospitalization and chemotherapy-related toxicity, shifting the overall risk-to-benefit ratio. Older patients and patients with important comorbidities are underrepresented in clinical trials. Because of the lack of data as well as the fear of iatrogenic complications, older patients generally receive less aggressive treatment, which may result in suboptimal survival rates. In selected patients, it has been shown that intensive, state-of-the art therapy benefits older patients. Remarkably, the 5-year survival rate is 15% to 20% with radiotherapy alone for patients with stage III disease who are older than 75 years, have good performance status, and have thorough staging. Defeatism thus is definitively not appropriate. Moreover, oncologic assessment methods specifically designed for older patients have been developed. The clinical implementation of these methods will lead to more rational and appropriate care of older patients with cancer. These methods probably are also useful for younger, frail patients. These developments should move the field forward.
Concurrent Medication (Other Than Chemotherapy)
Many patients take medications because of comorbidities. The influence of common medications on the side effects associated with radiotherapy in patients with lung cancer is unknown. Angiotensin-converting enzyme inhibitors and statins are the groups of medications that have received the most interest as being potential protectors against radiation pneumonitis. However, at the present time, their influence on side effects has not been clarified.
Molecular Factors
Molecular and genetic markers that could be used to select patients for a specific radiation schedule are of great interest. However, although some single-nucleotide polymorphisms have been associated with radiation pneumonitis and although C4b-binding protein alpha chain and vitronectin have been associated with toxicity, these and other findings need to be validated in other data sets and their therapeutic role needs to be investigated in prospective trials.
A worse survival rate has been reported for patients with a high pretreatment level of C-reactive protein or interleukin-6 in addition to classic prognostic factors. Again, the practical utility of these findings needs to be determined.
Smoking Status
Tobacco consumption is the primary cause of most lung cancers, and smoking during treatment can have a substantial negative effect on outcomes. In a study of 237 patients with complete smoking histories who were treated with definitive radiotherapy or chemoradiotherapy between 1991 and 2001, Fox et al. reported worse survival for smokers with early-stage disease. Similarly, Rades et al., in a study of 181 patients who received radiotherapy for the treatment of NSCLC, reported that improved local–regional control was associated with a lower T stage ( p = 0.007) and with no smoking during radiotherapy ( p = 0.029) but not with hemoglobin levels or respiratory insufficiency. Jin et al., in a study of 576 patients, found that smoking status, when corrected for dose–volume effects, was the only factor that appeared to reduce the risk of treatment-related pneumonitis. Nguyen et al. reported that smoking at the time of the initial consultation was associated with reduced local and local–regional control in patients who were treated with radiotherapy after surgery for the management of NSCLC. It is therefore important to ensure that patients with lung cancer have access to smoking-cessation programs and that concerted efforts are made to help them to stop smoking before, during, and after treatment.
Nutrition
The nutritional status of patients with lung cancer can be compromised as a direct result of cancer-induced alterations in metabolism and the side effects of radiotherapy. Koom et al. reported malnutrition in more than one-third of patients with cancer in a multi-institutional study. Malnutrition can lead to weight loss and, in the most serious cases, cachexia. Nutritional status may be associated with survival and ideally should be assessed before the initiation of any treatment, including radiotherapy. Weight loss is associated with poor survival among patients who are treated with radiation, and cachexia is characterized by involuntary weight loss, muscle wasting, decreased quality of life, and poor survival. In general, these conditions are not contraindications to radiotherapy. Nutritional assessment and weight monitoring are vital during radiotherapy for the treatment of lung cancer. Patients who maintain good nutrition are more likely to tolerate the side effects of treatment. Adequate calories and protein can help to maintain strength and prevent further catabolism. Individuals who do not consume adequate calories and protein use stored nutrients as an energy source, which leads to protein wasting and further weight loss. Patients with lung cancer need to be educated about radiation esophagitis, a common side effect that decreases oral intake and compromises nutritional status.
Fatigue and Physical Activity
Fatigue is one of the most common and distressing patient-reported symptoms associated with cancer. Rather than being a selection measure for radiotherapy, fatigue is a condition that needs to be managed in order for treatment to be successful. Physical training has been shown to be beneficial for patients with breast and colorectal cancer. Patients with lung cancer often have a higher incidence and longer duration of cancer-related fatigue, leading to decreased lung function and increased functional impairment in daily living and dramatically reducing the tolerability of treatment, the quality of life, and the chance for prolonged survival. Physical activity has been shown to reduce fatigue in patients with lung cancer and COPD, and studies have indicated that exercise therapy may be an important consideration in the management of both early- and advanced-stage lung cancer. For patients receiving concurrent radiotherapy, the value of physical training or pulmonary rehabilitation is limited, although the level of physical activity appears to be an additional prognostic factor to the level of performance. Preliminary evidence from a small pilot study suggested that physical training was associated with an improvement in 6-minute walking distance. Until more evidence is available, we believe that patients who receive radiation-based therapy would behave similarly to those who have surgery or chemotherapy alone and would equally benefit from physical training. These patients should be advised to remain as active as possible and to continue to engage in regular physical activity and pulmonary training to the level of their tolerance.
Repeat Radiation
As the prognosis of patients with cancer improves, more individuals are at risk for the development of a local recurrence or a new primary tumor in organs that were previously treated with radiation. New radiation techniques, better imaging, and more knowledge of dose–volume relationships have led to the use of repeat radiation at high doses.
We are aware of only one prospective study of repeat radiotherapy for recurrent lung cancer, with the rest being retrospective. In the prospective trial, which included 23 patients with a local recurrence after external radiotherapy, the median interval between primary and repeat radiotherapy was 13 months. The median first dose was 66 Gy, and the median second course was 51 Gy (range, 46 Gy to 60 Gy), delivered in 1.8 Gy to 2 Gy per fraction. No clinically severe toxicity was noted, but the 1-year and 2-year survival rates were only 59% and 21%, respectively. The results of the prospective study were in line with those of the retrospective series, which included 29–48 patients. However, in one study, severe toxicity, including lethal bleeding, was reported in 3 of 11 patients with centrally located tumors that were treated with SABR. Cumulative doses in excess of a cumulative biologically effective dose of 120 Gy to the aorta clearly should be avoided.
Repeat radiotherapy can be considered for selected patients. In the future, individual radiosensitivity measurements, possibly with genetic profiles, may contribute to appropriate patient selection.
Tumor-Related Factors
The management of lung cancer is determined largely by the extent of the disease. Patients who are selected for treatment with definitive radiotherapy must have disease that can be encompassed within a tolerable radiotherapy target volume, and they should have no distant metastasis. The great majority of patients who are treated with curative-intent radiotherapy have stage III disease, but there has been an increasing trend toward the use of SABR for the management of stage I disease in patients who are unable to tolerate surgical resection. Accurate determination of stage involves a synthesis of all available sources of information, which may include the results of bronchoscopy, the operative findings at the time of thoracoscopy or thoracotomy, the results of pathologic evaluation of lymph node samples obtained by transbronchial biopsy, and the findings on imaging studies, both structural and functional. Only a small proportion of patients who are treated with definitive radiotherapy or chemoradiation therapy would have had full mediastinal staging at the time of thoracotomy, and therefore imaging plays the central role both in selecting patients for curative therapy and in defining the target for treatment. Ultrasound-guided transbronchial biopsy has been assuming an increasing role in confirming the status of suspicious nodes that are detected on imaging studies, ensuring both that patients with false-negative nodes are not inappropriately excluded from surgery and that equivocal nodes can be included within the radiotherapy target volume if they are true positive.
Accurate staging of patients with NSCLC involves correct allocation of T, N, and M stages according to the international staging system, the current edition of which resulted from the International Association for the Study of Lung Cancer Lung Cancer Staging Project. The most accurate imaging staging modality currently available is 18 F-2-deoxy- d -glucose-positron emission tomography–computed tomography ( 18 FDG-PET–CT), which has rapidly supplanted CT and 18 FDG-PET alone when the newer modality has become available. There is a large and growing body of evidence that shows that PET and PET–CT are superior to CT for the determination of mediastinal node status and for the detection of distant metastasis. However, 18 FDG-PET does not provide accurate staging of the brain, and therefore candidates for curative radiotherapy who are at high risk for occult brain metastasis (e.g., patients with involvement of mediastinal nodes) should have separate imaging of the brain, ideally with magnetic resonance imaging.
Three prospective studies have evaluated the use of PET and PET–CT to select patients for definitive radiotherapy. In the first study, 153 candidates for radical radiotherapy had staging with PET after being found suitable for radiotherapy on the basis of conventional imaging. PET and conventional staging evaluations were discordant approximately 40% of the time, and only two-thirds of the patients actually received definitive radiotherapy because, in the remaining patients, PET showed either distant metastasis (18%) or intrathoracic disease that was too extensive for high-dose radiotherapy (12%). The predominant impact of PET was upstaging, and this effect was greatest for patients with the most advanced tumors. In the second study, a group of Polish investigators reported that only 75 of 100 patients with NSCLC remained eligible for curative radiotherapy after PET–CT. In the third study, 25 of 75 candidates were found to be unsuitable for definitive radiotherapy after PET–CT was performed with the patient in the radiotherapy planning position. The survival rate for patients who actually received definitive radiotherapy after PET–CT was remarkably good, with 32% of patients with stage IIIA disease being alive at 4 years. Only 4% of patients who received palliative treatment in the study survived for 4 years, suggesting that that PET-based patient selection had been appropriate.
Radiated Volume and Toxicity
The relationship between the volume of normal tissue that is radiated and the risk of serious pulmonary toxicity, including radiation pneumonitis and later radiation-induced fibrosis, is complex. It is made even more complex by the interaction of radiation-induced lung damage and serious preexisting cardiopulmonary comorbidities that may influence the capacity of the patient to endure high-dose radiation without becoming disabled or even dying as a result of toxicity. Consequently, the volume of lung and other normal tissue, such as heart tissue, that will be included in the target volume is an important factor that determines the suitability of a patient for definitive radiotherapy. If the volume of normal tissue, especially lung tissue, is too great, curative radiotherapy is simply not possible and other therapeutic approaches, such as palliation, must be preferred.
This consideration raises the question “What volume of normal tissue is too large?” This decision often was subjective in the past, but the advent of advanced radiotherapy planning systems has made it possible to precisely estimate the volume of lung tissue that will be radiated to any particular dose level. With the increase in computing power, it has become straightforward to display radiation lung volumes as dose–volume histograms, and these dosimetric parameters have been compared with clinical outcomes in large numbers of patients. The risk of pneumonitis can be estimated from a number of different but related dosimetric parameters. These parameters include the percentage of total lung volume radiated to 5 Gy (V5), 20 Gy (V20), and 30 Gy (V30) and the MLD. As these values increase, the risk of complications increases. The risk tends to increase with concurrent chemotherapy and may be decreased by smoking. The use of intensity-modulated radiotherapy may lead to an increase in the volume of lung tissue that is exposed to lower doses of radiation, and this increase should be accounted for to avoid potentially fatal complications. The Quantitative Analysis of Normal Tissue Effects in the Clinic report recommended that V20 (the volume of both lungs minus the planning target volume receiving 20 Gy) should be limited to no more than 30% to 35% and that the total MLD should be limited to no more than 20 Gy to 23 Gy (with conventional fractionation) to limit the risk of radiation pneumonitis to 20% or less in patients with NSCLC who are treated with definitive radiotherapy.
These dose limits cannot be relied on in all cases. For example, patients who have had previous pneumonectomy or other major lung resections will be less capable of surviving pneumonitis, and it has been suggested that a V5 of less than 60%, a V20 of less than 4% to 10%, and an MLD of less than 8 Gy are reasonable dose limits for intensity-modulated radiotherapy in the setting of mesothelioma after pneumonectomy. Although these parameters are useful for conventional daily fractionation, limited data on their utility for multiple daily fractionation or accelerated schedules are available. Similarly, dose constraints for SABR applied to the lung are in the process of being established. Severe pneumonitis is relatively rare after SABR, but it is clear that increasing values of V20 and MLD are associated with an increased risk of pneumonitis.
F-2-Deoxy- d -Glucose Uptake
In lung lesions, the association between increased glucose metabolism and malignancy is powerful. Intensely metabolically active lesions are much more likely to be malignant than lesions with little or no FDG uptake on PET. Lesions with low FDG avidity are commonly benign or are of low-grade malignancy. The results of a systematic review showed that primary malignant tumors with greater FDG uptake are generally aggressive and are associated with a worse prognosis. However, it is less clear whether FDG uptake is an independent prognostic factor because FDG avidity is strongly correlated with the extent of disease and the size of the tumor. In surgically treated patients with stage I or II NSCLC, a higher standard uptake value (SUV) is associated with lower rates of disease-free survival and overall survival. It also has been reported that a higher SUV and metabolic tumor volume are associated with worse prognosis in patients with stage I NSCLC who are treated with SABR. A number of retrospective studies have indicated that a higher SUV is associated with a worse prognosis for patients who have definitive chemoradiation therapy for the management of locally advanced disease. However, a large prospective multicenter trial involving patients with locally advanced NSCLC who were managed with definitive chemoradiation therapy (ACRIN 6668/RTOG 0235) did not confirm a significant effect of the pretreatment SUV but did suggest that the posttreatment SUV was important. In that study, pretreatment SUV was evaluable for 226 patients and posttreatment SUV was evaluable for 173 patients. The mean pretreatment SUV peak and SUV max values (10.3 and 13.1, respectively) were not associated with survival. However, the posttreatment SUV peak value was associated with survival in a continuous variable model (hazard ratio, 1.087; 95% confidence interval, 1.014–1.166; p = 0.020). The authors concluded that a higher posttreatment SUV (SUV peak or SUV max ) was associated with worse survival in cases of stage III NSCLC, although a clear cutoff value for routine clinical use could not be recommended.
Computed Tomography and Positron Emission Tomography Metrics
Although small tumor volume may be associated with a better prognosis, this benefit is no longer evident at 5 years, and tumor size per se should not be a factor influencing radical chemoradiation therapy. Increasingly, researchers are aware that, in addition to the volume of tumors, which is partly reflected in the T stage, images show many other features that could be both prognostic and predictive. In addition to the primary tumor, all regional and distant metastatic deposits could be evaluated and classified on the basis of imaging studies.
PET has been used for this purpose. Beyond FDG, PET-labeled drugs such as 11 C-docetaxel and 11 C-erlotinib and other molecules that could be used together with radiotherapy are of great interest for the future.
As CT is widely available and highly standardized, features of CT images increasingly have been investigated. For example, texture on CT images is quantified as mean gray-level intensity, entropy, and uniformity. Goh et al. found a relationship between the texture features of NSCLC on noncontrast-enhanced CT and tumor metabolism and stage. In the study by Win et al., univariate analysis demonstrated CT-derived heterogeneity, 18 FDG-PET–derived heterogeneity, diffusion-enhanced (dynamic contrast-enhanced)-CT-measured permeability, and stage were prognostic for survival of patients with NSCLC. In the same study, multivariate analysis showed that permeability was the most important survival predictor, followed by stage and CT-derived textural heterogeneity. The same technology also could be used to characterize organs at risk in order to improve the individual therapeutic ratio.
Mutation Status
Although most NSCLC tumors do not express a molecular target that can be blocked with currently available drugs, it is conceivable that this proportion will grow dramatically in the coming years. The question arises whether these molecular characteristics influence the sensitivity for radiotherapy.
Unfortunately, we are not aware of any prospective studies on this subject. Overexpression of epidermal growth factor receptor (EGFR) is associated with resistance to chemotherapy and radiotherapy. EGFR modulates DNA repair via an association with the catalytic subunit of DNA protein kinase. However, although in vitro EGFR expression correlates with both cellular and tumor response to radiation, in vivo EGFR expression does not. In addition, the type of EGFR inhibition turned out to be important: only the monoclonal antibody cetuximab improved local tumor control in some, but not all, tumors, whereas EGFR tyrosine kinase inhibitors were not synergistic. Although several retrospective reports have indicated that patients who have a tumor with EGFR mutation have a better response to radiotherapy and a longer progression-free survival than patients who have a tumor with wild-type EGFR , it remains to be shown if the long-term survival will be increased.
The relationship between radiosensitivity and the echinoderm microtubule-associated protein-like 4-anaplastic lymphoma kinase ( EML4-ALK ) fusion gene has not been investigated in depth. Contrasting results have been reported on the radiosensitivity of tumor cells bearing an ALK fusion protein with or without crizotinib treatment. To our knowledge, at the time of writing, no clinical data are available.
At the present time, mutational status does not affect the treatment strategy with regard to the use of radiotherapy.
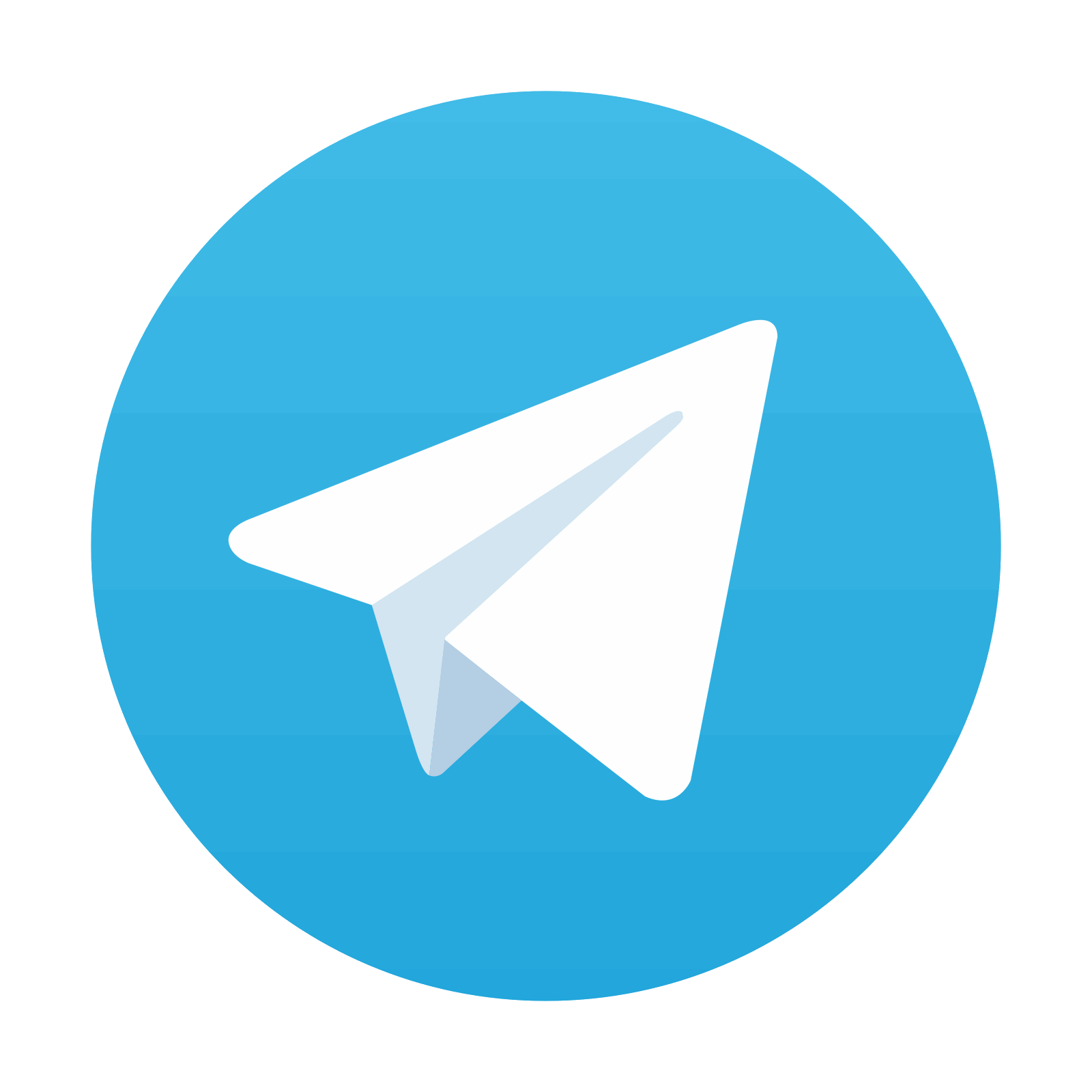
Stay updated, free articles. Join our Telegram channel

Full access? Get Clinical Tree
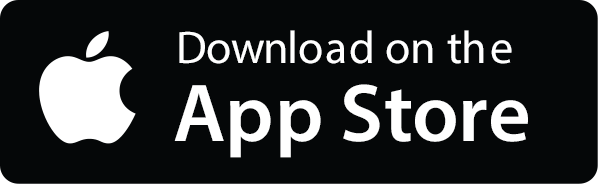
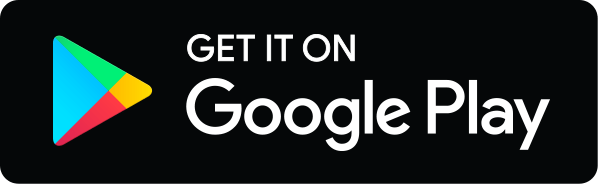