Summary of Key Points
- •
While 85% to 90% of lung cancer is attributable to cigarette smoking, substantial evidence exists to support genetic susceptibility to this disease.
- •
A family history of lung cancer is associated with a 1.5 to 4-fold increased risk of lung cancer after adjustment for the clustering of smoking in families.
- •
A family-based linkage study has identified a region on chromosome 6q that segregates with lung cancer in high-risk lung cancer families. PARK2 has been identified as one possible lung cancer susceptibility gene in this region.
- •
Genome-wide association studies have identified several regions associated with lung cancer risk. These include chromosome 15q25, containing CHRNA3 and CHRNA5 , chromosome 6p21, containing BAT3 and MSH5 , and chromosome 5p15, containing TERT and CLPTM1L.
- •
Challenges remain in better defining lung cancer susceptibility genes, with studies underway using whole genome and whole exome sequencing methods and considering other populations including African-Americans and never-smokers.
Acknowledgments
This work was supported in part by the Intramural Research Program of the National Human Genome Research Institute, National Institutes of Health (NIH), and by funding from NIH R01CA148127 and P30CA022453.
Lung cancer is the most common cause of cancer death in the United States, with an estimated 158,080 deaths in 2016 (accounting for 27% of all cancer deaths), and the second most frequent cancer diagnosed, behind breast cancer in women and prostate cancer in men, with an estimated 224,390 new diagnoses in 2016. Both lung cancer incidence and mortality rates have decreased with the reduction in tobacco smoking; however, lung cancer continues to be the cause of substantial morbidity and mortality. Survival remains poor, with a 5-year survival rate of about 17%. The 5-year survival rate has changed little over time because lung cancers are still most often diagnosed at advanced stages when treatment is less effective. Only recently has there been evidence that screening for lung cancer using low-dose computed tomography is an effective means of reducing mortality. In 2013, the US Preventive Services Task Force issued a recommendation for lung cancer screening for high-risk individuals. Advances in the treatment of lung cancer have also been slow. Since the early 2000s, treatment targeted to molecular signatures in lung tumors, such as epidermal growth factor receptor ( EGFR ) inhibitors, has resulted in improved survival in particular subgroups of patients. Unfortunately, the development of drug-resistant mutations is a problem that affects overall survival for patients with lung cancer, and continued drug development is crucial. To better understand the profile of a high-risk individual and to aid in the development of chemopreventive agents and targeted treatments, it is essential to understand the genetics underlying lung cancer development.
Cancer of the lung has frequently been cited and is a well-established example of a malignancy that is solely determined by the environment, with risks associated with cigarette smoking and certain occupations, such as mining, asbestos exposure, shipbuilding, and petroleum refining. About 85% to 90% of lung cancer risk is attributable to cigarette smoking. However, lung cancer develops in only 15% of smokers, suggesting a differential susceptibility to the effects of tobacco carcinogens. It is possible that variation in genetic profiles contributes to this differential susceptibility. In addition, 10% to 15% of lung cancers occur in never-smokers. Little is understood about risk in never-smokers, although exposure to secondhand cigarette smoke certainly contributes to the risk of lung cancer. Environmental tobacco smoke exposure has been associated with a 20% to 30% increased risk for the development of lung cancer among never-smokers. In a meta-analysis of 22 studies, the authors reported that exposure to tobacco smoke in the workplace increased the risk of lung cancer by 24% and this increased risk was highly correlated with duration of exposure.
There is overwhelming evidence of the carcinogenic effects of cigarette smoking and other environmental exposures and the occurrence of multiple somatic mutations in lung tumors. Known mutations and loss of heterozygosity in oncogenes and tumor suppressor genes involved in lung carcinogenesis accumulate in individual somatic cells during lung tumor initiation and progression. In 2013, the systematic genetic analysis of alterations in lung tumors was described in several published reports. The Cancer Genome Atlas noted that the results of sequencing 178 squamous cell carcinomas demonstrated the complexity of lung tumors, with a mean of 360 exonic mutations, 165 genomic rearrangements, and 323 copy number alterations per tumor. These observations highlight the genetic complexity of lung cancers compared with most other cancers. Recurrent mutations were identified in 11 genes. A total of 64% of cases carried a somatic alteration in a gene for which a targeted treatment could be proposed based on currently existing therapies (although many of these therapies are not currently indicated for lung cancer). Similarly, sequencing of 183 lung adenocarcinoma tumor/normal DNA pairs showed a mean exonic somatic mutation rate of 12 events per megabase. Higher mutation rates were seen among smokers than among never-smokers, and the mutation signature varied with smoking. Several previously identified mutations were reported, including those in tumor protein p53 ( TP53 ), Kirsten rat sarcoma ( KRAS ), EGFR , serine/threonine kinase 11 ( STK11 ), and v-raf murine sarcoma viral oncogene homologB ( BRAF ). In addition, novel candidates were identified. In total, 25 genes were significantly mutated and often associated with smoking history, age, stage, and progression-free survival. Smaller numbers of small cell lung tumors have been sequenced. In another study of 53 tumor samples/normal tissue pairs, investigators identified 22 significantly mutated genes, including members of the sex determining region Y (SRY)-box ( SOX ) family of genes. Susceptibility to selected mutations also varies according to host-specific factors. For example, mutations in EGFR are much more common in women, Asians, and never-smokers and individuals presenting with adenocarcinomas, while mutations affecting KRAS are more common in men, individuals of European descent, smokers, and those with squamous histology. Susceptibility to somatic mutations may be due to individual differences in risk associated with the inhalation of known carcinogens; i.e., individuals differ in their susceptibility to these environmental insults. The potential role that inherited germline genetic variation plays in influencing lung cancer susceptibility is the topic of this chapter.
Evidence indicates that allelic variation at genetic loci affects inherited susceptibility to lung cancer. Epidemiologic evidence demonstrates familial aggregation of lung cancer after adjusting for familial clustering of cigarette smoking and other risk factors, and differential susceptibility to lung cancer is inherited in some families. Studies of inherited susceptibility to lung cancer, including major susceptibility loci and loci with less pronounced effects, are described in this chapter. Also discussed is how these genetic risks relate to well-known environmental factors, particularly cigarette smoking.
Biologic Risk Factors
When determining whether susceptibility to a complex disease or trait such as lung cancer has a genetic component, three questions are typically addressed in family-based studies.
- 1.
Does the lung cancer cluster or aggregate in families? If risk of lung cancer is inherited, one would find more clustering of lung cancer beyond what would be expected by chance.
- 2.
If lung cancer does aggregate in families, can it be explained by shared environmental/cultural risk factors? For lung cancer, one must assess whether familial aggregation of lung cancer is driven solely as a result of clustering of smoking behaviors or other environmental exposures within families.
- 3.
If the excess familial clustering is not explained by measured environmental risk factors, is the pattern of lung cancers in families consistent with mendelian transmission of a major gene; i.e., transmission in some families of a rare, moderately high-penetrance risk allele, and can this gene(s) be localized and identified in the human genome?
In addition, inherited susceptibility may be acquired through a more common, low-penetrance risk allele that may interact with environmental exposures. Evidence in support of this type of inheritance of risk is most likely derived from case-controlled, and not family-based, studies.
Evidence for Familial Aggregation of Lung Cancer
Twin Studies
For common diseases, investigators often perform twin studies as a first approach to estimating the impact of genetic factors in disease causation. In twin studies, investigators typically report the observed concordance rates for lung cancer among monozygotic (MZ) twins compared with the observed concordance rates for lung cancer among dizygotic (DZ) twins. Identifying enough twins to conduct a meaningful analysis of cancer risk has been challenging; the combination of data from the Swedish, Finnish, and Danish registries with national cancer registries allowed investigators to analyze data on 44,708 pairs. The results of this analysis showed substantial increases in risk for cotwins, with relative risks of 7.7 and 6.7 for male MZ and DZ cotwins, respectively; for female MZ and DZ cotwins, the risks were 25.3 and 1.8, respectively. Furthermore, a biometrical analysis of these data was performed, and investigators estimated that 26% of variance in risk was attributable to heritable factors, 12% to shared environmental factors, and 62% to individual-specific risks. The estimated heritable proportions are comparable to estimates for breast and ovarian cancer but lower than estimates for colorectal or prostate cancers. Strong evidence against the so-called constitutional hypothesis, proposed by Sir Ronald Fisher, was found in studies of MZ twins discordant for smoking, and the same host factors predispose these individuals to both smoking and lung cancer; therefore smoking does not have any direct causal link to lung cancer risk. However, a large US study showed a much lower relative risk when comparing smoking with nonsmoking MZ cotwins (5.5), and higher risk in DZ cotwins (11.0), which suggests a partial role of genetic factors in both smoking and lung cancer.
Case–Control and Case–Family Cohort Studies
In 1963, Tokuhata and Lilienfeld showed familial aggregation of lung cancer. After they accounted for personal smoking, the results suggested the possible interaction of genes, shared environment, and common lifestyle factors in the etiology of lung cancer. In their study of 270 people with lung cancer and 270 age-, sex-, race-, and location-matched controls and their relatives, the authors found a 2.0-fold to 2.5-fold increased lung cancer mortality among smoking relatives of people with lung cancer compared with smoking relatives of control participants. Nonsmoking relatives of people with lung cancer were also at higher risk than nonsmoking relatives of control participants. Smoking was a more important risk factor than family history of lung cancer for men, but family history was more important for women. The authors also noted a synergistic interaction between family history of lung cancer and smoking, with a much higher risk of lung cancer among smoking relatives of people with lung cancer than among either nonsmoking relatives of people with lung cancer or smoking relatives of control participants. Additionally, the authors found a substantial increase in mortality related to other respiratory diseases in relatives of people with lung cancer compared with relatives of control participants, suggesting that the relatives of people with lung cancer have a common susceptibility to respiratory diseases. No significant differences were noted between the spouses of people with lung cancer and control participants with respect to lung cancer mortality, mortality from other respiratory diseases, or smoking habits. One strength of this study was that risk factor data, including age and smoking status, were collected for the relatives; however a major weakness of the study was that smoking status alone, and not smoking intensity or duration, was used, so there was potential for residual confounding due to clustering of smoking habits in families.
Since this initial study, authors of several other studies have reported familial aggregation of lung cancer. The best designed studies considered the number of relatives in the families and the risk factor profiles of each relative so that the effect of familial clustering of smoking habits could be taken into account, and the discussion focused on these types of studies. In southern Louisiana, authors of case-controlled studies reported an increased familial risk of lung cancer and other smoking-related cancers among relatives of lung cancer probands (the index case leading the family to be studied) after the effects of age, sex, occupation, and smoking history had been accounted for. In these studies, investigators performed familial aggregation analyses on 337 lung cancer probands (cases), their spouses (controls), and the parents, siblings, half-siblings, and offspring of both the cases and controls. The probands were white men and women who died of lung cancer between 1976 and 1979 in a 10-parish (county) area of southern Louisiana. A strong excess risk of lung cancer was detected among first-degree relatives of probands compared with relatives of controls after adjustment for age, sex, smoking status, total duration of smoking, number of cigarette pack-years, and a cumulative index of occupational/industrial exposures. The risk of lung cancer for parents of probands was fourfold compared with parents of controls. Women older than age 40 years who were relatives of probands were at a nine times higher risk of lung cancer than similar female relatives of controls, even among nonsmokers without excessive exposure to hazardous occupational materials. Heavy-smoking female relatives of probands had a fourfold to sixfold increased lung cancer risk compared with heavy-smoking female relatives of controls. Overall, the lung cancer risk was greater for male relatives of probands than for their female counterparts. After controlling for the confounding effects of the measured environmental risk factors, the authors found that relationship to a lung cancer proband (i.e., having a family history of lung cancer) remained a determinant of lung cancer, associated with a 2.4-fold higher lung cancer risk.
This same set of families was evaluated to determine whether cancers other than lung cancer were associated with similar familial aggregation. Proband families were 1.7 times more likely than control families to have one family member other than the proband with cancer, and 2.2 times more likely to have two family members with cancer. Comparing case relatives and control relatives, families that had three cancers or four or more cancers occurred with relative risks of 3.7 and 5.0, respectively. The most striking differences in cancer prevalence between case and control families were noted for cancers of the nasal cavity/sinus, mid-ear, and larynx (odds ratio, 4.6); trachea, bronchus, and lung (odds ratio, 3.0); skin (odds ratio, 2.8); and uterus, placenta, ovary, and other female organs (odds ratio, 2.1). After controlling for age, sex, cigarette smoking, and occupational/industrial exposures, the authors found that the risk of cancers other than lung cancer remained significantly increased for relatives of cases compared with relatives of controls ( p < 0.05).
Etzel et al. conducted a large case–control study in Texas that adjusted for smoking histories among relatives and discovered similar findings. The authors studied 806 lung cancer cases and 663 matched controls in the Houston area and reported familial aggregation of lung cancer and smoking-related cancers after adjustment for smoking histories of the cases and controls and their relatives. In this study, familial aggregation was not stronger in families of early-onset cases (defined as diagnosed at age 55 years or younger) or in families of never-smokers.
Familial aggregation of lung cancer in families of early-onset lung cancer cases was evaluated by Cote et al. in a large study in metropolitan Detroit that enrolled 692 white and black individuals with early-onset lung cancer (defined as diagnosed before the age of 50 years), along with 773 frequency-matched population-based control participants. Data on risk factors, including smoking pack-years, age, sex, and a history of other lung diseases, were collected for each first-degree relative of the people with early-onset lung cancer and control participants. After adjustment for these risk factors, relatives of people with early-onset lung cancer were twice as likely to have lung cancer compared with relatives of control participants. The lung cancer risk associated with family history of lung cancer was highest in black families. Increased lung cancer risk in relatives of never-smoking individuals with lung cancer was not reported in this study, however the sample size was small. The authors of the study reported a 1.5-fold increased risk of tobacco-related cancers. This risk was even higher when the analysis included only black families.
The largest study of familial aggregation involved the evaluation of people with lung cancer and control participants in Iceland. The authors of the study used a population-based approach and obtained familial risks of 2.69 when parents of people with lung cancer were compared with parents of control participants, and this risk increased to 3.48 when parents of people younger than age 60 years (early-onset lung cancer) were compared with age-matched control participants. The risk to siblings was increased 2.02-fold in general but increased to 3.3-fold for siblings of people with early-onset lung cancer.
Studies of never-smokers with lung cancer are limited. Schwartz et al. found an increased risk of lung cancer among relatives of younger, population-based nonsmokers with lung cancer compared with relatives of younger control participants after adjustment for smoking, occupation, and medical history of each family member. Mayne et al. studied never-smokers and former smokers (cessation at least 10 years before they were interviewed) and reported that a positive family history of lung cancer was associated with increased lung cancer risk after adjustment for age and smoking status.
In addition to studies that include an analysis of risk factor data among relatives, a meta-analysis of 28 case–control studies and 17 cohort studies demonstrated fairly consistent findings, with an approximately twofold increased risk of lung cancer associated with family history across a range of study designs. Risk was generally higher for relatives of people in whom lung cancer was diagnosed at a young age and when multiple family members were affected. A recent pooled analysis from the International Lung Cancer Consortium included data from approximately 24,000 lung cancer cases and 23,000 controls, making it the largest study to date. The authors reported a 1.5-fold increased risk of lung cancer associated with a family history, after adjustment for smoking and other potential confounders in cases and controls, and a 1.25-fold increased risk for never-smokers. No variation in familial risk by histology was noted. When the analysis was limited to studies that included risk factor data for each family member, relative risks for lung cancer among relatives with a family history of lung cancer were 1.55 overall, 1.53 for white, 2.09 for black, and 1.97 for relatives of people with early-onset lung cancer (diagnosed before 50 years of age). The findings from these studies help to answer the first two questions posed at the beginning of this chapter: there is substantial evidence for familial aggregation of lung cancer and it remains after adjustment for clustering of cigarette smoking within family members.
High-Risk Syndromes Conferring an Increased Risk of Lung Cancer
Further evidence suggesting an inherited component to lung cancer is the occurrence of lung cancer in families with inherited, well-defined cancer syndromes. Leonard et al. reported that survivors of familial retinoblastoma may be at increased risk for small cell lung cancer (SCLC). The standard mortality ratio for SCLC estimates a 15-fold increased risk. Kleinerman et al. reported lung cancer developing among individuals with germline retinoblastoma mutations and a history of heavy smoking. Overall, retinoblastoma survivors tend to smoke less than the general population, suggesting that targeting counseling to avoid this risky behavior in this high-risk population may be effective. The retinoblastoma gene is inactivated in 90% of SCLCs, indicating the biologic relevance of this gene in SCLC etiology.
Germline mutations in the p53 gene cause the inherited Li-Fraumeni syndrome. Individuals with this syndrome are at greater increased risk for many other cancers, including breast and lung cancers, sarcomas, leukemias and lymphomas, and adrenocortical tumors. The standard incidence ratio for lung cancer was estimated at 38 in a prospectively collected cohort of p53 mutation carriers. Cigarette smoking further increased an individual’s risk by threefold.
Mutations in the EGFR gene are often found in adenocarcinomas of the lung arising in never-smoking women and in Asian populations. One family with multiple lung adenocarcinomas was found to have segregation of an EGFR mutation, indicating that rarely inherited mutations of this locus can increase the risk of lung cancer. However, in another study of 237 familial lung cancer cases from families with three or more affected relatives, including 45 bronchoalveolar cancers, investigators failed to find any germline EGFR -T790 mutations, suggesting that inheritable mutations in this gene are uncommon in the general US population.
Segregaton Analyses of Lung and other Tobacco-Related Cancers
Given the evidence for familial aggregation of lung and other tobacco-related cancers, after adjustment for familial clustering of smoking habits, determining whether patterns of transmission within families is consistent with at least one major, high-penetrance genetic locus is the next step in answering the last question posed at the beginning of this chapter. Sellers et al. performed genetic segregation analyses on the lung cancer proband families in the study by Ooi et al. The trait was expressed as a dichotomy, affected or unaffected with lung cancer. These analyses used the general transmission probability model, which allowed for variable age of onset of the lung cancers. The likelihood of the models was calculated using a correction factor appropriate for single ascertainment, that is, conditioning the likelihood of each pedigree of the probands being affected by their age at examination or death.
Age of onset of lung cancer was assumed to follow a logistic distribution that depended on pack-years of cigarette smoke exposure and its square, an age coefficient, and a baseline parameter. Results indicated compatibility of the data with mendelian codominant inheritance of a rare major autosomal gene that produces cancer at an earlier age of onset. Segregation at this putative locus may account for 69% and 47% of the cumulative incidence of lung cancer in individuals up to ages 50 and 60 years, respectively. The gene was predicted to be involved in 22% of all lung cancer in persons up to age 70 years, a reflection of an increasing proportion of noncarriers becoming affected by long-term exposure to tobacco.
Gauderman et al. reanalyzed these same data from Ooi’s study using a Gibbs sampler method to examine gene by environment interactions and found evidence of a major dominant susceptibility locus that acts in conjunction with cigarette smoking to increase risk. This analysis was very similar to the previous study results because the codominant mendelian models predicted very small numbers of homozygous susceptibility allele carriers.
Yang et al. performed a complex segregation analysis on the families of never-smoking lung cancer probands in metropolitan Detroit from the study by Schwartz et al. The authors found evidence of mendelian codominant inheritance with modifying effects of smoking and chronic bronchitis in families of never-smoking cases. The estimated risk allele frequency was 0.004. Although homozygous individuals with the risk allele were rare in the study population, penetrance was very high for early-onset lung cancer (85% in men and 74% in women by age 60). The probability of lung cancer developing by 60 years of age in individuals heterozygous for the rare allele was low in the absence of smoking and chronic bronchitis (7% in men and 4% in women), but in the presence of these risk factors it increased to 85% in men and 74% in women, which was the same level predicted for homozygotes. The attributable risk associated with the high-risk allele declined with age, when the role of tobacco smoking and chronic bronchitis become more important. Investigators conducted a small study in Taiwan that analyzed the families of 125 female never-smoking lung cancer probands and found evidence for effects from a dominant genetic locus.
The Taiwan, Detroit, and Louisiana studies share remarkably similar results and provide substantial evidence for at least one major gene that acts in conjunction with smoking and possibly with chronic bronchitis to increase the risk of lung cancer in families. Segregation studies have some limitations. The studies did not include all potential risk factors for lung cancer, such as passive smoking or occupational exposures, in each relative, and only one study included history of other lung diseases in the models. Furthermore, segregation analyses are not sufficient to prove the existence of a major locus because only a subset of all possible models can be tested. Segregation analyses are useful, however, because they provide a model that can be used in family-based linkage studies aimed at the identification of a specific lung cancer gene. These analyses also provide insights into the best study design for identifying genes that confer a high risk of disease.
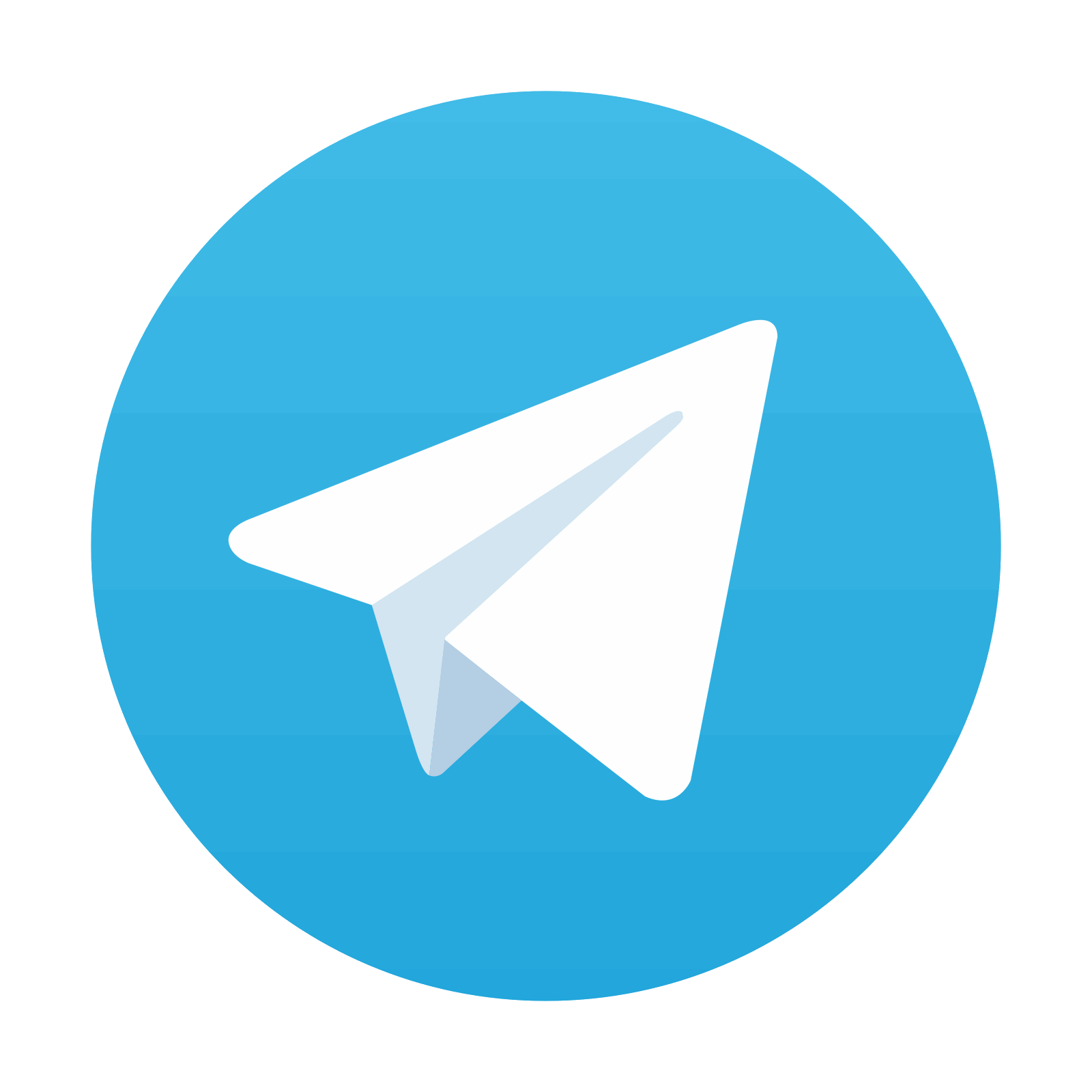
Stay updated, free articles. Join our Telegram channel

Full access? Get Clinical Tree
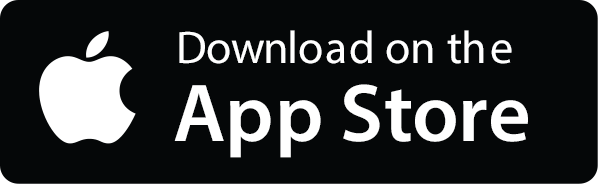
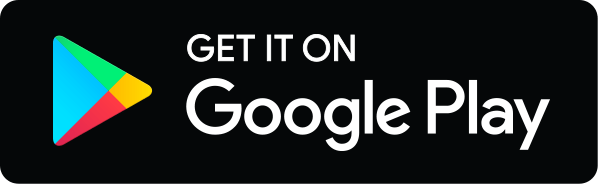