Adrenocortical adenoma
Micronodular disease
Macronodular disease
MAS
PPNAD/iMAD/CNC
BMAH
A. Genetic alterations
1. cAMP/PKA signaling pathway
–
–
MC2R a (missense)
–
GNAS a
–
GNAS a
GNAS a (postzygotic)
PRKAR1A b (allelic losses)
PRKAR1A b (LOH)
–
–
PRKACA a (missense or insertion)
PRKACA c
PRKACA c
–
PDE8B b
PDE8B b
PDE8B b
–
PDE11A b
PDE11A b
–
2. Armadillo proteins
–
–
ARMC5 b (LOH, nonsense or missense)
–
CTNNB1 a AXIN2 a
CTNNB1 a
–
–
3. Other
–
–
MEN1 b , FAP b , FH b , EDNRA, DOTL1, HDAC9, PRUNE2
–
B. Abnormal protein expression
GPCR
–
GPCR
ACTH
Serotonin, vasopressin
–
PRKAR1A
–
PRKAR1A
–
–
PRKACA
–
–
–
Glucocorticoid receptor
–
–
–
Estrogen receptor
–
–
Genetic Alterations Leading to Abnormal Steroidogenesis
Role of cAMP/PKA Signaling Pathway in Adrenal Steroidogenesis and Proliferation
In primary adrenal causes of CS, the production of corticotropin-releasing hormone (CRH) in hypothalamus and of adrenocorticotropin (ACTH) by the corticotroph cells is suppressed by excess secretion of cortisol. The binding of ACTH to its specific melanocortin type 2 receptor (MC2R) regulates cortisol secretion; MC2R is a seven transmembrane domain receptor that belongs to the family of G-protein-coupled hormone receptor (GPCR) [32, 33]; it is expressed on zona fasciculata cells that interacts with MC2R-associated proteins [34] and induces the dissociation of Gs-α subunit, which generates cAMP from ATP by activation of adenylate cyclase (AC) [35]. The second messenger cAMP and its effector protein kinase A (PKA) are key regulators of adrenocortical cells. PKA is a prototypical serine/threonine kinase consisting of a dimer of two regulatory (with four known isoforms RIα, RIβ, RIIα, RIIβ) and two catalytic subunits (with four isoforms Cα, Cβ, Cγ, Prk) [36]. They constitute a tetramer in its inactive holoenzyme form [37] where two cAMP molecules are needed to bind to specific domains of the R subunits of PKA thereby dissociating the tetramer and releasing the C subunit (PRKACA ) from its inactivating regulatory subunits; activated PRKACA phosphorylates different intracellular targets, including the transcription factor c-AMP-responsive element-binding protein (CREB). The latter activates the transcription of cAMP-responsive element containing genes in the nucleus including cholesterol transporters and steroidogenic enzymes, which stimulates acutely cortisol synthesis and chronically cellular proliferation [38, 39]. Specific phosphodiesterases (PDEs) are responsible of the degradation of the intracellular cAMP in order for the two R and C subunits of PKA to be reassembled to return to their inactive state [10] (Fig. 1a). Therefore, the cAMP signaling pathway appears to play a fundamental role in regulation of metabolism, cell replication, differentiation, and apoptosis in adrenal tissues; this implies that any defect in this pathway leading to its constitutive activation would be expected to result in cell proliferation and excess hormone production [40] (Table 1).


Fig. 1
(a) Schematic representation of the cAMP signaling pathway involved in the control of cortisol secretion in primary adrenal Cushing’s syndrome. The binding of corticotropin (ACTH) to the melanocortin type 2 receptor (MC2R) leads to dissociation of Gs-α subunit and activation of adenylate cyclase (AC) generating cAMP from ATP. The binding of cAMP to specific domains of the regulatory subunits of protein kinase A (R1 α) dissociates the tetramer thereby releasing the catalytic subunit (C α), which phosphorylates different intracellular targets, including the transcription factor CREB; the latter activates the transcription of cAMP-responsive element-containing genes in the nucleus including cholesterol transporters and steroidogenic enzymes. Specific phosphodiesterases (PDEs) are responsible of the degradation of the intracellular cAMP in order for the two R1 α and C α subunits of PKA to be reassembled to return to their inactive state. Genetic defect in this pathway leading to its constitutive activation can underlie tumor development and excess hormone production. (b) Bilateral macronodular adrenal hyperplasia cells can express several functional aberrant G protein-coupled hormone receptors (GPCR). Activation of these receptors by their natural ligands induces the activation of intracellular cascade similar to the one activated normally by the binding of ACTH to MC2R thereby stimulating the release of both cortisol and locally produced ACTH which also triggers cortisol production through autocrine and paracrine mechanisms involving the MC2R. (c) Armadillo repeat-containing 5 (ARMC5), a new indirect or direct regulator of steroidogenesis and apoptosis. ARMC5 inactivating mutations induce a decreased steroidogenic capacity and a protection against cell death
MC2R Mutations
MC2R mutations are extremely rare causes of adrenal hyperplasia or tumor formation [41, 42]. In only two patients with BMAH, constitutive activation of the MC2R with consequent enhanced basal receptor activity resulted either from impaired desensitization of a C-terminal MC2R mutation (F278C) [43] or from synergistic interaction between two naturally occurring missense mutations in the same allele of the MC2R: substitution of Cys 21 by Arg (C21R) and of Ser 247 by Gly (S247G) [44].
Gs-α Subunit Mutations
Activating mutations of the Gs-α subunit of heterotrimeric G protein also termed gsp mutations (GNAS) were the first identified in primary adrenal CS [45, 46]. It occurred in a mosaic pattern in some fetal adrenal cells during early embryogenesis resulting in the local constitutive activation of the cAMP pathway. This mutation was identified initially in the McCune–Albright Syndrome (MAS) where a minority of patients develops nodular adrenal hyperplasia and CS among other more common manifestations such as café au lait spots and bone fibrous dysplasia or other endocrine tumors causing ovarian precocious puberty, acromegaly, or hyperthyroidism [45, 47]. In MAS patients with CS, GNAS mutations are found in the cortisol-secreting nodules, whereas the internodular adrenal cortex which is not affected by the mutation becomes atrophic as ACTH becomes suppressed. Isolated somatic GNAS mutations can also occur in 5–17 % of cortisol-secreting adenomas [48–50] and in rare cases of BMAH [51, 52] without any other manifestations of MAS. This suggests that the somatic mutation in MAS occurs at an early stage of embryogenesis in cells which are precursors of several tissues. In isolated BMAH, the somatic mutation probably occurs in mosaic pattern in more differentiated adrenocortical progenitor cells only which will migrate to generate bilateral macronodular adrenal glands; a somatic GNAS mutation giving rise to a unilateral adenoma occurs later in life in a single committed zona fasciculata cell.
PRKAR1A Mutations
PRKAR1A is an adrenocortical tumor suppressor gene according to in vitro and transgenic mouse studies. Its inactivation leads to ACTH-independent cortisol secretion [36, 53]. Constitutive PKA activation due to PRKAR1A mutations results either from reduced expression of the RIα subunits or from impaired binding to C subunits [54]. Loss of RIα is sufficient to induce autonomous adrenal hyperactivity and bilateral hyperplasia and was demonstrated for the first time in vivo in an adrenal cortex-specific PRKAR1A KO mouse model referred to as AdKO. Pituitary-independent CS with increased PKA activity developed in AdKO mice with evidence of deregulated adrenocortical cells differentiation, increased proliferation, and resistance to apoptosis. Moreover, RIα loss led to regression of adult cortex and emergence of a new cell population with fetal characteristics [53]. In vitro and in vivo models of PPNAD (AdKO mice) showed that PKA signaling increased mTOR complex 1, leading to increased cell survival and possibly tumor formation [55]. Tumor-specific loss of heterozygosity (LOH) involving the 17q22-24 chromosomal region harboring PRKAR1A and inactivating mutations of PRKAR1A are responsible for CS in isolated or familial PPNAD and CNC [54, 56–58]. They are found in more than 60 % of patients with CNC and in up to 80 % of CNC patients who develop CS from PPNAD [57, 59]. Furthermore, somatic allelic losses of the17q22–24 region and inactivating mutations in PRKAR1A were identified in 23 and 20 % of adrenocortical tumors, respectively [60]. Although, PRKAR1A mutations are not found in BMAH, somatic losses of the 17q22–24 region and PKA subunit and enzymatic activity changes show that PKA signaling is altered in BMAH similarly to what is found in adrenal tumors with 17q losses or PRKAR1A mutations [61]. CS presenting in persons younger than 30 years of age with bilateral, small (usually 2–4 mm in diameter), black-pigmented adrenal nodules are all characteristics of PPNAD. A distinctive feature of PPNAD compared to BMAH is the presence of atrophy in the internodular adrenal tissue. CNC is a familial autosomal variant that includes PPNAD among other tumors such atrial myxomas, peripheral nerve tumors, breast/testicular tumors, and GH-secreting pituitary tumors along with skin manifestations [62]. Patients with CS due to PRKAR1A mutations tend to have a lower BMI with evidence of increased PKA signaling in periadrenal adipose tissue, which is in concordance with the role of PKA enzyme in the regulation of adiposity and fat distribution [63].
PRKACA Mutations
The most frequent mechanism of adrenal CS secondary to unilateral adrenal adenoma involves somatic mutations in the gene encoding the catalytic subunit of PKA (PRKACA). They occur in patients diagnosed with CS at a younger age (45.3 ± 13.5 vs. 52.5 ± 11.9 years) [49] with a female predominance [64]. The first two mutations identified in a cohort of ten cortisol-producing adrenal adenomas were shown to inhibit the binding of the R subunit making the Cα subunit constitutively active [65]. A combination of biochemical and optical assays, including fluorescence resonance energy transfer in living cells showed that neither mutant can form a stable PKA complex, due to the location of the mutations at the interface between the catalytic and the regulatory subunits [66]. The most common mutation p.Leu206Arg was present in 37 % of these adrenal tumors [65]. It consists of substitution of a small hydrophobic leucine with a large positively charge hydrophilic arginine at position 206. It is located in the active cleft of the C subunit and it inactivates the site where the regulatory subunit RIIβ binds leading to cAMP-independent PKA activation. The second mutation (Leu199_Cys200insTrp) entails the insertion of a tryptophan residue between the amino acid 199 and 200 and was present in one case only. Later, two novel mutations were identified in a study of 22 adrenal adenomas with CS with p.Cys200_Gly201insVal and p.Ser213Arg + p.Leu212_Lys214insIle-Ile-Leu-Arg being found in three and one adenomas, respectively. They indirectly interfere with the formation of a stable PKA holoenzyme by impairing the association between C and R subunits [67]. Other groups confirmed the presence of these mutations in unilateral adrenal adenomas with overt hypercortisolism at a rate of 23–65 % [48, 49, 64, 67, 68]. However, they are seldom present in adenomas with mild cortisol secretion, which might justify why subclinical CS rarely becomes overt CS [65, 67, 68]. These observations suggest that subclinical CS has a different genetic etiology than overt CS rather than being a part of the same pathophysiological spectrum [69]. In contrast to somatic mutations causing cortisol-secreting adenomas, germline complex genomic rearrangements in the chromosome 19p13.2p13.12 locus, resulting in copy number gains that includes PRKACA gene rarely lead either to micronodular or macronodular hyperplasia depending on the extent of gene amplification [65, 70, 71]. Finally, Bimpaki et al. demonstrated that adrenal adenomas of patients with CS could have functional abnormalities of cAMP signaling, independently of their GNAS, PRKAR1A, PDE11A, and PDE8B mutation status most probably due to epigenetic events or other gene defect s [72].
PDE Mutations
PDE play a role in the hydrolysis of cAMP . There are two types of PDE8 enzymes coded by two distinct genes, PDE8A and PDE8B, which are highly expressed in steroidogenic tissues such as the adrenal, ovaries, and the testis as well as in the pituitary, thyroid, and pancreas [73, 74]. Genetic ablation of PDE8B in mouse models or long-term pharmacological inhibition of PDE8s in adrenocortical cell lines was shown to increase the expression of steroidogenic enzymes such as StAR and p450scc (CYP11A); furthermore, they potentiated ACTH stimulation of steroidogenesis by increasing cAMP-dependent PKA activity [75]. A PDE8B missense mutation (p.H305P) was described in a young girl with isolated micronodular adrenocortical disease (iMAD), which is a nonpigmented micronodular hyperplasia without PRKAR1A [76]. HEK293 cells transfected with the PDE8B mutant gene exhibited higher cAMP levels than with wild-type PDE8B, indicating an impaired ability of the mutant protein to degrade cAMP [76]. Other inactivating mutations in phosphodiesterase 11A isoform 4 gene (PDE11A) and 8B (PDE8B) have been also described in adrenal adenomas, carcinomas, and BMAH [1, 50, 72, 75, 77–79].
Role of Armadillo Proteins in Adrenal Tumorigenesis
Armadillo Proteins form a large family of proteins that are characterized by the presence of tandem repeats of a 42 amino acid motif with each single ARM-repeat unit consisting of 3 α-helices [80]. The most well-known protein of this family is β-catenin , which is crucial in the regulation of development and adult tissue homeostasis through its two independent functions, acting in cellular adhesion in addition to being a transcriptional coactivator (Fig. 2b). Deregulation in the Wnt⁄ β-catenin signaling pathway is involved in the pathogenesis of adrenocortical adenomas and carcinomas (Fig. 2c). Armadillo repeat containing five (ARMC5) is a novel Armadillo (ARM)-repeat-containing gene and encodes a protein of 935 amino acids; its peptide sequence reveals two distinctive domains: ARM domain in the N-terminal and a BTB/POZ in the C-terminal (Bric-a-Brac, Tramtrack, Broad-complex/Pox virus, and Zinc finger) [81]. ARMC5 mutations were recently identified to be related to primary bilateral macronodular adrenal hyperplasia [80] (Fig. 1c).


Fig. 2
(a) Schematic representation of deregulation in the Wnt⁄β-catenin signaling pathway involved in the pathogenesis of adrenocortical adenomas and carcinomas. In the absence of a Wnt signal, β-catenin is captured by adenomatous polyposis coli (APC) and axin within the destruction complex, facilitating its phosphorylation by casein kinases 1 α (CK1 α) and glycogen synthase kinase-3 β (GSK3β) through the ubiquitin pathway involving interactions with β-transducin repeat-containing protein (β-TrCP). (b) The presence of Wnt ligand inhibits the destruction complex activity. Therefore, β-catenin is accumulated in the cytoplasm; it may migrate to the nucleus where it activates the transcription of target genes, upon its activation. (c) β-catenin mutations block its phosphorylation within the destruction complex preventing its ubiquitinylation and proteasomal degradation; consequently, β-catenin accumulates in the nucleus and forms active transcription factor complexes with T cell factor/lymphoid enhancer factor proteins (TCF/LEF)
β-Catenin Mutations
Regulatory mechanisms of cortisol production in adrenocortical carcinomas remain not fully elucidated. Decreased activity of steroidogenic enzymes translates into elevated urinary metabolites of several androgens or glucocorticoid precursors [82]. However, the Wnt⁄β-catenin signaling pathway appears to play a key role in the pathogenesis of both adrenal adenomas and carcinomas. β-catenin forms a complex with other proteins (adenomatous polyposis coli and axin, which facilitates its phosphorylation, making it available for degradation in the absence of Wnt signaling [83] (Fig. 2a). Adrenocortical carcinomas can harbor among other mutations in conserved serine/threonine phosphorylation sites at the amino terminus of β-catenin that block its phosphorylation within the destruction complex, thereby preventing its ubiquitinylation and proteasomal degradation; consequently, it accumulates in the nucleus and forms active transcription factor complexes with T cell factor/lymphoid enhancer factor proteins [83] (Fig. 2c, Table 1). Although CTNNB1 mutations are mainly observed in larger and nonsecreting adrenocortical adenomas, suggesting that the Wnt/β-catenin pathway activation is associated with the development of less differentiated tumors, Bonnet et al. described β-catenin mutations in 6 and 8 out of 19 and 46 subclinical and overt cortisol-producing tumors, respectively [84]. Recently, Goh et al. identified β-catenin (CTNNB1) mutations as responsible for 16 % of the cortisol-secreting adenomas [49]; they were also noted by other groups in some cases of adrenal adenomas with CS or SCS [64, 85, 86]. Somatic β-catenin mutations were also found in 2 out of 18 patients with PPNAD (11 %). In both cases, the mutations occurred in relatively larger adenomas that had formed in the background of PPNAD [87] (Table 1). Somatic CTNNB1 mutations may explain only about 50 % of β-catenin accumulation observed in adrenocortical tumors, indicating that other components of the Wnt pathway may be involved; in fact, genetic alterations of the negative regulator of Wnt signaling, “AXIN2 gene” were identified in adrenocortical adenomas and carcinomas yet at a low prevalence , 7 and 17 %, respectively [88] (Fig. 2c, Table 1).
ARMC5 Mutations
Inactivating germline mutations in ARMC5 gene were first described in apparently sporadic cases of BMAH [89] (Table 1). The bilateral nature of macronodular hyperplasia as well as its long and insidious onset motivated the search for a genetic predisposition that could result in earlier diagnosis and better management to avoid bilateral adrenalectomy. Single-nucleotide polymorphism arrays, microsatellite markers, whole-genome and Sanger sequencing were applied to genotype leucocyte and tumor DNA obtained from patients with BMAH. The search for the responsible genes was conducted in apparently sporadic and familial cases [89–94]. The initial germline mutation in the ARMC5 gene, located at 16p11.2 was detected in 18 out of 33 apparently sporadic tumors 55 % of cases of BMAH with Cushing’s syndrome [89]. Further studies in sporadic cases found that the prevalence of germline ARMC5 mutations was closer to 25 % [90, 92, 93]. Inactivation of ARMC5 is biallelic, one mutated allele being germline and the second allele being a somatic secondary event that occurs in a macronodule; these findings are consistent with its role as a potential tumor suppressor gene according to Knudson’s 2-hits model [89, 92]. Correa et al. demonstrated that ARMC5 has an extensive genetic variance by Sanger sequencing 20 different adrenal nodules in the same patient with BMAH [95]. They found the same germline mutation in the 20 nodules (p.Trp476* sequence change) but uncovered 16 other mutation variants in 16 of the nodules. This suggests that the germline mutation is responsible for the diffuse hyperplasia but second somatic hits are required to enhance adrenal macronodular formation [89, 95]. In the first large BMAH family studied, a heterozygous germline variant in the ARMC5 gene (p.Leu365Pro) was identified in all 16 affected Brazilian family members as well as other mutations in two of three other families [92]. Interestingly, only two mutation carriers had overt CS and the majority had subclinical disease and one carrier had no manifestations despite being 72 year old. In addition, in one-third of the affected individuals only unilateral adrenal lesion was present as progression of the full-blown disease, needing many years and requiring the occurrence of additional somatic mutations in several macronodules. This raises the question of the prevalence of ARMC5 mutation in apparently unilateral incidentalomas in the general population. Other families with BMAH have also been identified with ARMC5 mutations or alterations [91, 94, 96, 97]. A germline deletion rather than mutation of ARMC5 was reported in a family presenting with vasopressin-responsive SCS and BMAH [96]. By applying droplet digital polymerase chain reaction, the mother and her son had germline deletion in exon 1–5 of ARMC5 gene locus. Furthermore, Sanger sequencing of DNA from the right and left adrenal nodules as well as peripheral blood of the son revealed the presence of another germline, missense mutation in ARMC5 exon 3 (p.P347S) [96].
The presence of ARMC5 mutation in patients with BMAH and aberrant GPCR has been reported, but the relationship has not been well established yet. The most frequent aberrant responses were to upright posture, isoproterenol, vasopressin, and metoclopramide tests [89, 90, 97]. In contrast, none of the patients with food-dependent CS carried ARMC5 mutations [89, 90]. ARMC5 inactivation decreases steroidogenesis, and its overexpression alters cell survival, which could argue why relatively inefficient cortisol overproduction is seen despite massive adrenal enlargement [7, 8, 98]. Despite this, the index cases operated for Cushing’s syndrome and carrying ARMC5 mutations carriers presented more severe CS than cases operated for Cushing’s syndrome without ARMC5 mutation; carrier patients had a more severe clinical phenotype and biochemical profile as well as larger adrenals on imaging with a higher number of nodules [90, 93]. ARMC5 mutations appear to be the most frequent genetic alteration in BMAH with 61 different mutations, 27 germinal and 30 somatic, found all along the protein in different domains. Thus, genetic counseling and screening for these mutations are highly encouraged in family members of patients with BMAH even without the evidence of a clinical disease [8, 81, 92]. As ARMC5 appears to be a tumor suppressor gene and is widely expressed in many tissues other than the adrenal, it was of interest to examine whether mutation carriers could develop other tumors. In a few families with BMAH, the occurrence of intracranial meningiomas was described and a somatic ARMC5 mutation was found in a meningioma of a patient with familial BMAH with a germline ARMC5 mutation suggesting the possibility of a new multiple neoplasia syndrome [94]. Finally, ARMC5 mutations have been identified in primary hyperaldosteronism where 6 patients of 56 (10.7 %, all Afro-Americans) had germline mutations in the ARMC5 gene; among these 6 patients, 2 suffered from BMAH [99].
Other Genetic Defects Associated with Abnormal Steroidogenesis
Several other gene mutations have been reported in patients with CS mainly presenting with BMAH including the multiple endocrine neoplasia type 1 (MEN1), familial adenomatous polyposis (APC), type A endothelin receptor (EDNRA) [52, 98, 100, 101]. Furthermore, somatic mutations other than ARMC5 have also been found in patients with BMAH such as the DOT1L (DOT1-like histone H3K79 methyltransferase) and HDAC9 (histone deacetylase 9) genes; these two nuclear proteins are involved in the transcriptional regulation; however, their mutations were found at a much lower frequency than ARMC5 [64] (Table 1).
In a Carney Complex patient without Cushing’s syndrome but with skin pigmentation, acromegaly and myxomas, gene triplication of chromosome 1p31.1, including PRKACB, which codes for the catalytic subunit beta (Cβ) resulted in increased PKA activity. It is likely that whereas the loss of RIα leads to the full Carney complex phenotype, the gain of function in Cα leads to adrenal adenomas and Cushing’s syndrome, while in this case, amplification of Cβ resulted in certain nonadrenal manifestations of CNC [102].
Major Molecular Mechanisms Involved in Adrenal CS Other than Genetic Mutations
Independently of circulating ACTH, many bioactive signals released in the vicinity of adrenocortical cells by chromaffin cells, neurons, cells of the immune system, adipocytes, and endothelial cells can influence the secretory activity of the normal adrenal cortex [103, 104]. In contrast to the mechanisms that mainly lead to constitutive activation of the cAMP system or deregulation in the Wnt⁄β-catenin signaling pathway, abnormal regulation of steroidogenesis can result from the aberrant adrenal expression of several hormone receptors, particularly GPCR [105–108] and from aberrant autocrine/paracrine loops [9] (Fig. 1). These concepts offer the possibility of targeted therapy using specific receptor-targeted peptide antagonists [108]. These mechanisms are implicated in the pathogenesis of adrenal CS (Table 1) as well as in other endocrine tumors such as primary hyperaldosteronism and pituitary tumors; yet they are the most frequently described mechanism of regulation of hypercortisolism in BMAH [108]. Despite being a rare disease representing less than 1 % of all causes of CS [1], the prevalence of incidentally discovered BMAH due to extensive development and use of abdominal imaging has markedly increased [109]. It is diagnosed in the fifth and sixth decades and occurs more frequently in women [7]. The adrenal glands are hypertrophied with a mass reaching 10–100 times the normal weight of an adrenal gland [110]; however, most of the patients have subclinical hypercortisolism [98]. This discrepancy might be explained by the unequal distribution of steroidogenic enzymes among the different adrenocortical cell types leading to inefficient steroidogenesis [110, 111] as well as to decreased expression of steroidogenic enzymes [89, 112].
Aberrant Expression and Function of GPCR
The expression of ectopic receptors that are not expressed at significant levels in normal zona fasciculata cells and the increased expression or coupling to steroidogenesis of eutopic receptors can lead to abnormal cortisol production by mimicking the cellular events that are triggered normally by MC2R [7] (Fig. 1b). There are ectopic receptors such as those for glucose-dependent insulinotropic peptide (GIPR), β-adrenergic receptors (β-AR), vasopressin AVP (V2-V3 R), serotonin (5-HT7R), glucagon (GCGR), and angiotensin II (AT1R). Among eutopic receptors are those for vasopressin (V1R), luteinizing hormone/human chorionic gonadotropin (LHCGR), or serotonin (5-HT4R) [108]. Five systematic studies have screened for aberrant expression of GPCR in overt and SCS; they demonstrated abnormal expression of more than one type of GPCR with 80 % showing aberrant cortisol responses to at least one stimulus. Multiple responses within individual patients occurred with up to four stimuli in 50 % of the patients; AVP and 5-HTR4 agonists were the most prevalent hormonal stimuli triggering aberrant responses in vivo [52, 113–116]. The percentage of aberrant responses in patients with unilateral adenoma and mild CS or SCS was similar to those in BMAH patients [114]. However, it was less frequent in patients with unilateral adenomas and overt CS [113] most probably due to higher prevalence of PRKACA mutations in these patients [65].
Food-Dependent CS
Initially described by Hamet et al. in a case of unilateral adenoma and CS [117], food-dependent CS was identified to be GIP dependent by two different groups in cases of BMAH [118, 119]. To date, more than 30 cases of ectopic GIPR expression were published, being the most extensively studied GPCR in BMAH and unilateral adenomas, though it is not the most prevalent [107]. The transfection of bovine adrenal cells with the GIPR and its injection under the renal capsule in mice led to the development of hyperplastic adrenals and hypercortisolism which supports the role of the GIPR in steroidogenesis and cell proliferation [120]. Low fasting plasma cortisol levels in the morning due to suppressed pituitary ACTH, which increases following meals and its physiological elevation of GIP, is the hallmark of GIP-dependent CS. Since other aberrant GPCR can be expressed with GIPR in the same tissue such as LHCGR and 5-HT4R, fasting cortisol levels may not always be suppressed [121–123]. Short-term control of hypercortisolism in BMAH patients with aberrant expression of GIPR was achieved by octreotide or pasireotide presumably because GIP suppression escapes as downregulation of somatostatin receptors in K cells occurs during chronic administration of the long-acting agonists [124, 125]. In vitro, ACTH-receptor antagonists were able to significantly inhibit cortisol secretion in perifused GIP-dependent BMAH tissues because GIP stimulated ACTH secretion and this effect was reduced by blocking ACTH binding to its own receptor [9].
Posture-Dependent CS
Upright posture induces abnormal steroidogenesis in BMAH with aberrant expression of either β-adrenergic receptors (β-AR), vasopressin AVP (V2–V3-vasopressin receptor), or angiotensin II (AT1R). Further, in vivo testing with β-agonists, vasopressin, and angiotensin II can identify each of these aberrant receptors, respectively [107]. Administration of antagonists of V1aR, AT1R, or β-AR was effective in reducing cortisol levels in patients with posture-related CS [126–129] [130]. Posture and specifically AVP were the most prevalent hormonal stimulus triggering aberrant responses in vivo [108].
LH/hCG-Dependent CS
Cases of transient CS during sequential pregnancies with spontaneous resolution following delivery were reported, while persistent CS occurred only after menopause, as a consequence of aberrant adrenal expression of LH/hCGR; coexpression of LH/CGR with GIPR and 5-HT4R was found in some patients [121–123, 131, 132]. Some cases of CS outside of pregnancy were also found with aberrant cortisol response to injection of GnRH and hCG [123, 133]. A heterozygous mutation of Gsα at codon 201 was found in addition to the aberrant LH/CG receptors [134]. Chronically elevated serum LH following gonadectomy induced functional LH receptor expression in mouse adrenal cortex, leading to adrenal hyperplasia and LH-dependent hypercortisolism [135]. Leuprolide acetate, a GnRH analog, was able to achieve long-term control of hypercortisolism in LH/hCG-dependent CS [136]. Aberrant expression of LH/CGR and GNRHR was described in pregnant and postmenopausal patients with primary hyperaldosteronism [137]. Recently, activating β-catenin mutations (CTNNB1) were identified in aldosteronomas which largely overexpressed GNRHR and LHCGR, suggesting that these mutations stimulate Wnt activation and cause adrenocortical cells to de-differentiate toward their common adrenal-gonadal precursor cell type [138].
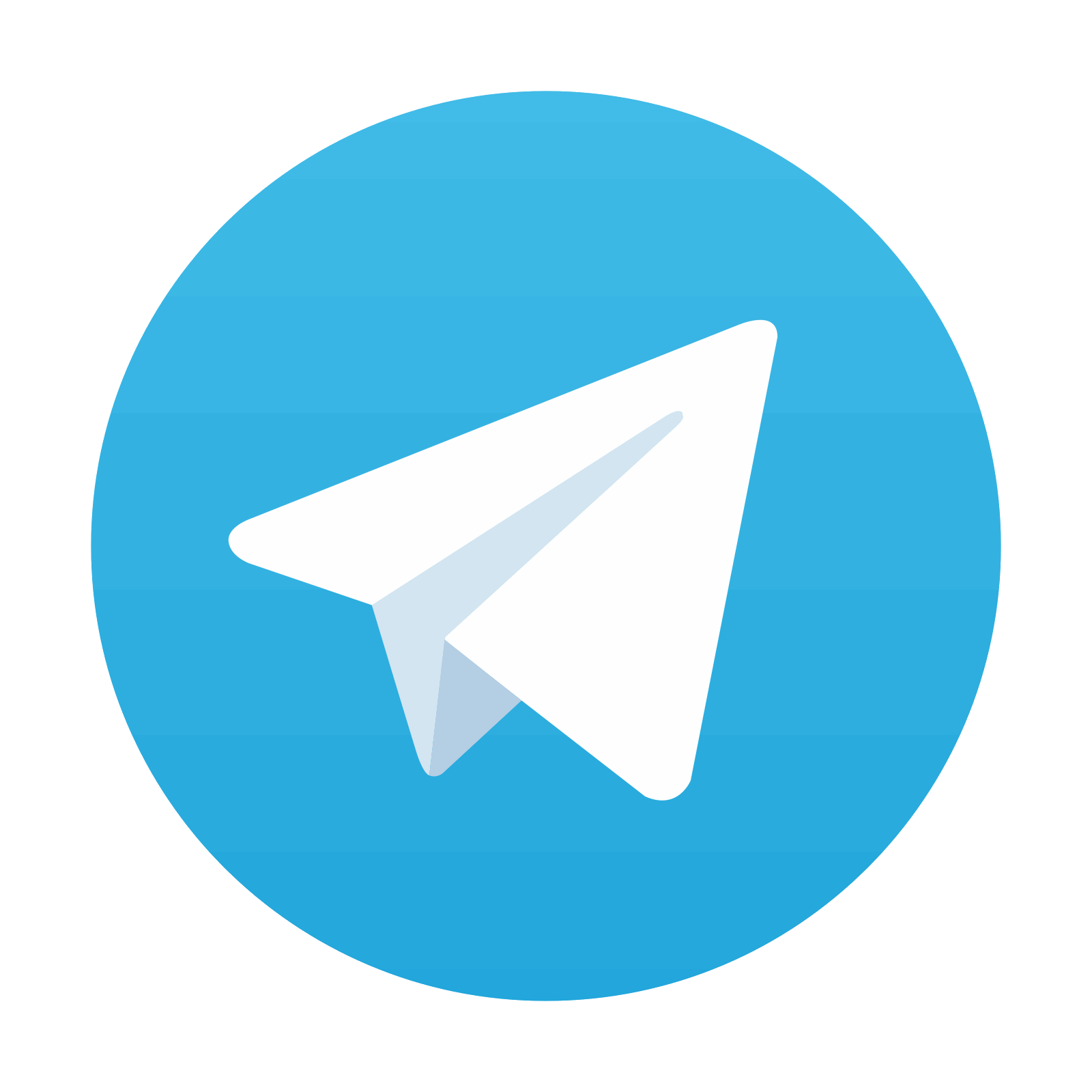
Stay updated, free articles. Join our Telegram channel

Full access? Get Clinical Tree
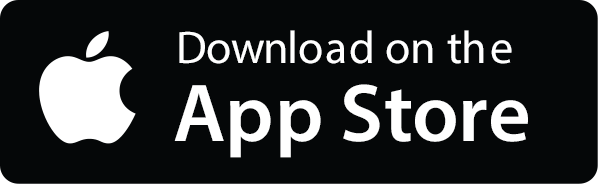
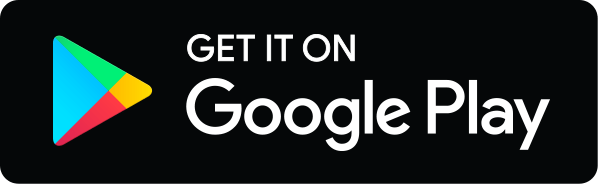