Palliation of Brain and Spinal Cord Metastases
BRAIN METASTASIS
Brain metastasis is very common, with an annual incidence of approximately 170,000 to 200,000. The rising incidence of brain metastasis is most likely from a combination of increasing survival from recent advances in systemic therapy and a greater availability and use of magnetic resonance imaging (MRI). The most common primary site is the lung followed by breast. Metastatic brain tumors outnumber primary brain tumors by a factor of 10 to 1, with autopsy series demonstrating a 10% to 30% incidence rate for all patients with a diagnosis of cancer (Table 93.1).1,2
Clinical Presentation, Diagnosis, and Prognosis
The majority of patients present with neurologic signs and symptoms (Table 93.2).3 Although differential diagnoses such as an abscess or a stroke must be considered, new-onset neurologic symptoms in a known cancer patient should always be presumed to be from brain metastasis until proven otherwise.
Given its ability to image in multiple orientations and sequences, including superior resolution and accuracy compared to computed tomography (CT), MRI has become the standard of care for imaging of the central nervous system (CNS) in cancer patients.4 MRI will frequently pick up smaller lesions not seen on CT scans, which can have a significant effect on the patient’s prognosis and treatment course. Full systemic workup (e.g., positron emission tomography [PET] and CT) should be promptly initiated if brain metastasis is the presenting event. The incidence of unknown primaries may subsequently decrease with the increasing popularity of integrated PET-CT scans.
Performance status and extracranial disease status have consistently been shown to impact prognosis. Gaspar et al.5 reported on the Radiation Oncology Therapy Group (RTOG) experience of 1,200 patients. This analysis revealed three recursive partitioning analysis (RPA) classes, with the RPA class I (Karnofsky performance score [KPS] ≥70, controlled primary, age <65 years, brain metastasis only), II (not meeting requirements of classes I or III), and III (KPS <70) having median survivals of 7.1 months, 4.2 months, and 2.3 months, respectively.
TABLE 93.1 EPIDEMIOLOGY OF BRAIN METASTASIS
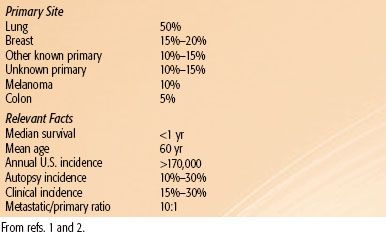
TABLE 93.2 CLINICAL PRESENTATION OF BRAIN METASTASIS
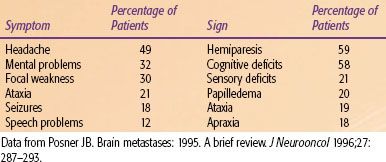
Corticosteroids
The initial therapy should promptly start with corticosteroids (e.g., dexamethasone or methylprednisolone), which effectively improve edema and neurologic deficits in approximately two-thirds of patients.6 The only randomized trial evaluating dosage was reported by Vecht et al.7 This trial included two successive groups of patients. The first group (n = 47) evaluated 8 mg per day versus 16 mg per day initial dexamethasone doses, with tapering schedules over 4 weeks. The second group (n = 49) evaluated 4 mg per day versus 16 mg per day of initial dexamethasone, with continuation of these doses for 28 days before tapering. The patients were scheduled for whole-brain radiotherapy (WBRT) and concurrent ranitidine. All arms had similar KPS improvements at 7 days (54% to 70%) and 28 days (50% to 81%). The study concludes that 4 mg per day of dexamethasone (with a taper over 4 weeks) is the preferable regimen. One should be cautious, however, in interpreting the results of this study. Patients in the 4 mg per day arm had to have the medication be reinstituted at a higher rate than the patients in the 8 or 16 mg per day arms. Furthermore, the arm with the greatest improvement in the KPS was the 16 mg per day arm when this was tapered over 4 weeks, compared with any of the other arms. It can be argued that higher KPS improvement arose from the maximal anti-inflammatory effects of the initial higher doses, with the 4-week taper minimizing the late toxicity associated with corticosteroids.
A reasonable corticosteroid regimen in patients with brain metastases is a 10 mg intravenous (IV) or oral bolus, followed by a 4 to 6 mg every 6 to 8 hours of dexamethasone equivalent dose (with a concurrent proton-pump inhibitor [PPI]), before this is tapered in a clinically cautious manner. In asymptomatic patients with little peritumoral edema or mass effect, initial corticosteroids may be reserved until the first sign of neurologic symptoms.
Whole-Brain Radiotherapy
WBRT continues to be the standard of care in patients with brain metastasis. In general, WBRT should be given soon after the diagnosis of brain metastasis. There has never been any evidence to suggest that delaying systemic chemotherapy for WBRT compromises overall survival, especially when one considers that progression in the brain frequently leads directly to the death of the patient.
There is still no agreement on the dose and fractionation schedule for WBRT, despite numerous studies designed to determine the optimal delivery. Table 93.3 summarizes selected randomized fractionation studies.8–12 Typically, the radiographic and clinical response rates range from 50% to 75%. Most of these studies have been negative, and the overall survival has not improved appreciably over the past 25 to 30 years. A total of 30 Gy in 10 fractions continues to be the standard for a vast majority of patients. In chemotherapy refractory RPA class III patients, a shorter fractionation scheme (e.g., 20 Gy in 5 fractions) should be considered. However, short fractionation schemes should be avoided in chemotherapy-naive patients with brain metastasis as the presenting event in the cancer diagnosis. The natural disease course of such patients can be frequently unpredictable, so they may live sufficiently long enough to experience late radiation toxicity posed by such short fractionation schedules.13
TABLE 93.3 SELECTED RANDOMIZED TRIALS EXAMINING VARIOUS FRACTIONATION SCHEDULES FOR BRAIN METASTASIS
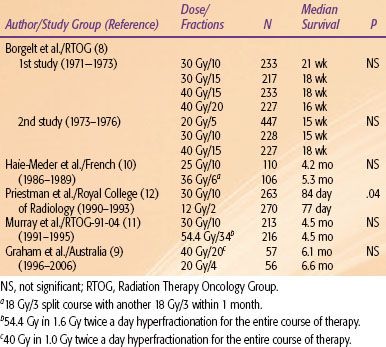
TABLE 93.4 RANDOMIZED TRIALS OF SURGICAL RESECTION OF SINGLE BRAIN METASTASIS
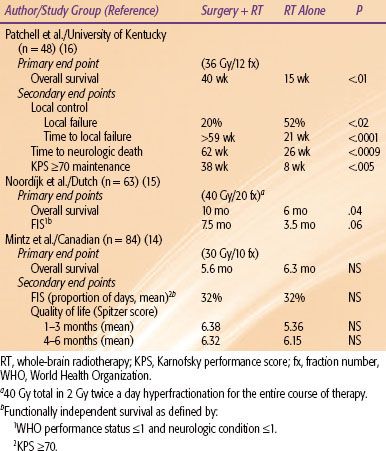
Surgical Resection
Surgical resection can provide immediate relief of the tumor mass effect (Fig. 93.1). On the other hand, radiation typically takes several days to work. Radiobiologically, 30 Gy in 10 fractions to a solid tumor (excluding radiosensitive tumors) is not adequate to achieve long-term tumor control. This issue is especially germane because historically up to one-half of all patients died from neurologic causes after being treated with WBRT alone.
There have now been three phase III trials testing the hypothesis that surgical resection to single brain metastasis is potentially beneficial. All three trials were on patients with a single lesion, which is defined as the presence of only one lesion in the brain regardless of the extracranial disease status, while a solitary lesion is defined as the presence of the CNS metastasis as the only site of the metastatic disease burden. Table 93.4 summarizes the three trials.14–16 The studies by Patchell et al.16 (KPS ≥70) and Noordijk et al.15 (World Health Organization grade ≤2) included better performance status patients compared with the Mintz et al.14 study (KPS ≥50), which mainly contributed to the differences in the survival outcomes between the studies. The results of these studies suggest that surgical resection should be reserved for lesions causing life-threatening complications or for those patients with good performance status (i.e., KPS ≥70).
Radiosurgery Boost Trials
Radiosurgery provides a substitute or alternative to conventional surgery. The three randomized trials of surgical resection were performed before the widespread availability of stereotactic radiosurgery (SRS). Although no randomized trials have been performed comparing surgery with SRS, SRS appears to provide similar local control rates (in the order of 80% to 90% only when combined with WBRT). Unless the tumor causes significant edema and mass effect, with consequent hydrocephalus or herniation requiring urgent surgical intervention, SRS can serve as a noninvasive alternative. Frequently, a patient may not be a craniotomy candidate because of tumor location in eloquent areas or existing medical contraindications. Although two of the three conventional surgery trials have shown a survival benefit in single brain metastasis, there have been no randomized trials addressing multiple lesions, and the retrospective data available are contradictory. For SRS there have been three randomized trials assessing the efficacy of SRS in the treatment of multiple metastases (Table 93.5).
The first randomized trial was reported by Kondziolka et al.17 from the University of Pittsburgh. This small study was stopped early at a planned interim analysis of 60% patient accrual because the authors reported having found a large difference in the primary end point of local control in favor of SRS (92% vs. 0%; P = .0016). Unfortunately, the study used nonstandard end points to measure recurrence, defining it as any increase in the lesion size on MRI. Furthermore, no attempt was made to control for steroid use, radiation changes, or other factors that might produce small fluctuations in the lesion size on MRI. Therefore, this study is difficult to interpret.
Chougule et al.18 from Brown University reported the second trial, although this is published only in abstract form. This trial had three treatment arms and randomized patients to treatment with SRS alone with Gamma Knife (Elekta Corp, Stockholm), SRS plus WBRT, or WBRT alone. This trial suffers from several methodological problems. Although the authors conclude that the survival times among the treatment arms were similar and that patients treated with SRS experienced superior local control and fewer brain metastases, no probability values are given. Furthermore, 51 of the patients had surgical resection for at least one symptomatic brain metastasis prior to entry into the study, and no attempt was made to stratify for previous surgery. The inclusion of the surgically resected patients effectively made this a six-arm trial and, therefore, the size of this trial was not large enough to support a meaningful analysis. Finally, the radiation doses used in the SRS arms cannot be considered conventional because the peripheral dose was not individualized based on the tumor size or volume.
In the third study, RTOG-95-08, the primary end point was overall survival, which was not statistically different between the WBRT plus SRS and WBRT alone arms (6.5 months and 5.7 months, respectively; P = .1356), although the SRS boost improved the survival in the subgroup (planned analysis) of patients with single metastasis.19 For secondary end points, the local control and performance measures, were higher in the SRS boost arm, but this did not translate into a lower death rate from neurologic progression. Multiple, unplanned subgroup analyses were made, and an overall survival benefit with the SRS boost was found in several subgroups that included patients with RPA class 1, tumor size ≥2 cm, and non–small cell lung cancer or metastatic squamous histology from any site. Unfortunately, these subset analyses were not planned or prespecified, and the probability values needed for significance should have been adjusted for inflation of the type I error. When this was done, none of these subgroup analyses showed a positive benefit for SRS.20 On the other hand, this trial did demonstrate that SRS is associated with lower edema and corticosteroid use, countering a commonly held notion that SRS actually increases the edema risk. However, regarding the major end points for multiple metastases, this study should be considered a negative trial.
Although SRS boost is indicated (from RTOG-95-08 and from the extrapolation of surgical resection data) in patients with a single metastasis, it is difficult to justify its routine use in patients with multiple metastases in light of the equivocal phase III SRS boost trials.
FIGURE 93.1. A: Six months after definitive therapy for stage IIIB non–small cell lung carcinoma (NSCLC), a patient presented with progressively worsening headache, nausea, vomiting, and coordination difficulties. T1-gadolinium-enhanced axial magnetic resonance imaging (MRI) demonstrates a large, necrotic right cerebellar mass. B: T2-axial MRI reveals large area of vasogenic edema, with resultant mass effect causing fourth ventricular compression and hydrocephalus (not shown). C: Patient was taken immediately for a craniotomy and a gross total resection was achieved. Pathology revealed metastatic NSCLC. Postoperative T1-gadolinium-enhanced axial MRI reveals no residual tumor, decompression of the mass effect, and re-expansion of the fourth ventricle.
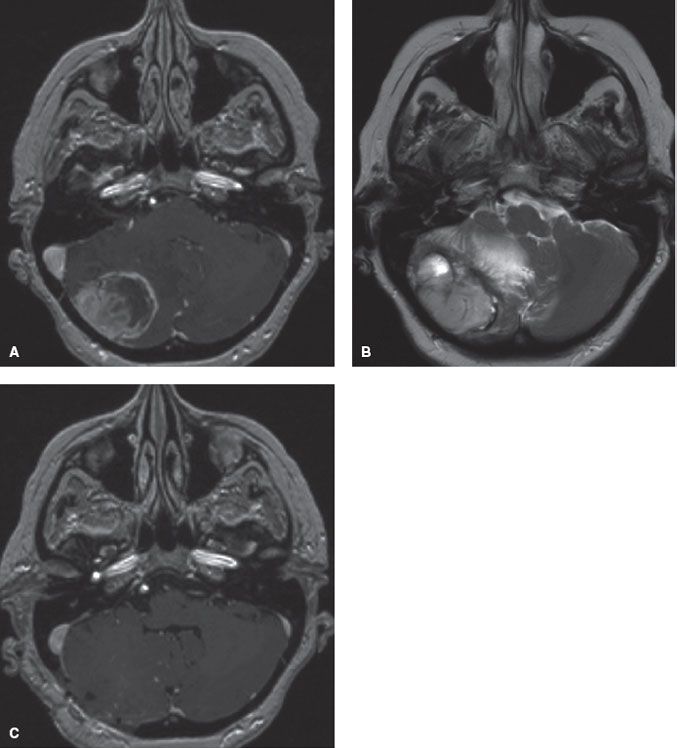
Postoperative or Postradiosurgery Whole-Brain Radiotherapy
A controversy in the treatment of brain metastasis is the routine use of postoperative or post-SRS WBRT. In a multi-institutional retrospective SRS study, Sneed et al.21 argue for the omission of upfront WBRT because this does not compromise overall survival. Unfortunately, only an overall survival analysis was performed, and no local control or retreatment data were given. In an earlier study by Sneed et al.22 on the University of California–San Francisco SRS experience, patients who were initially treated with SRS alone without WBRT experienced worse freedom from new brain metastasis and overall brain freedom from progression despite the imbalance of the prognostic factors that favored the SRS alone group, although the overall survival was not different. Because of the equivalency of overall survival, many have advocated withholding upfront WBRT. They often use repeat SRS for the failures, which can be very expensive. Furthermore, brain failure can lead to unacceptable consequences. Many incorrectly assume that with close follow-up, recurrences can be diagnosed early before symptoms. For example, Regine et al.23 reported on 36 patients with planned observation after initial SRS alone. Even with close follow-up with examinations and high-resolution MRIs, 47% of patients experienced brain failure, with 71% and 59% experiencing symptomatic relapse and neurologic deficits, respectively.
TABLE 93.5 RANDOMIZED TRIALS OF STEREOTACTIC RADIOSURGERY BOOST IN BRAIN METASTASES
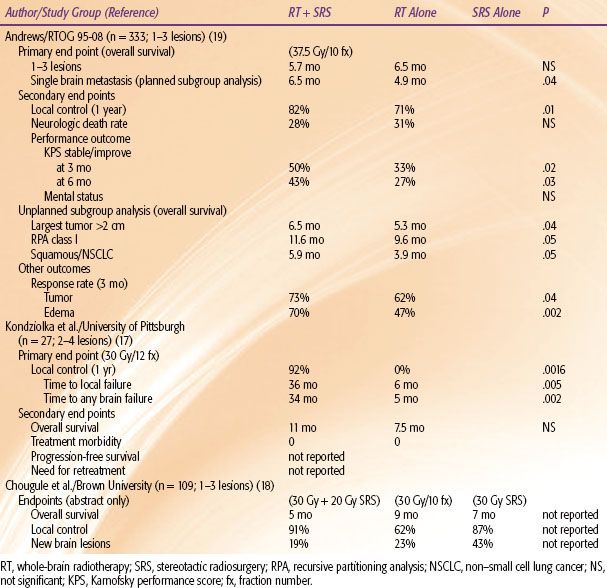
The omission of upfront WBRT may have even more serious consequences for patients with more radioresistant tumors such as renal-cell carcinoma (RCC). The SRS dose given is typically limited by tumor size and volume, not by whether the patient received additional dose with WBRT. Therefore, a patient treated with WBRT plus SRS receives much higher tumor dose than SRS alone. It is then not a surprise that the Eastern Cooperative Oncology Group protocol E6397 demonstrates very disappointing results.24 In this phase II trial that evaluated SRS alone in radioresistant tumors (RCC, melanoma, sarcoma), Manon et al.24 reported a 6-month total brain failure rate of 48.3%. The authors correctly conclude that routine avoidance of WBRT should be approached judiciously.
Fortunately, there have been five phase III trials that have assessed the use of postoperative or postradiosurgery WBRT (Table 93.6). Patchell et al.25 demonstrated that surgical resection without WBRT led to a failure rate at the original site and the entire brain of 46% and 70%, respectively. More importantly, 44% of the patients in the surgery alone arm died as a result of neurologic sequelae from brain failure. The results of this study have been frequently misinterpreted in the literature. Some have justified the withholding of upfront WBRT based on the fact that this study demonstrated equivalent survivals. In fact this study was designed with brain tumor recurrence rate as the primary end point and not overall survival. To show an overall survival difference, this trial needed to enroll over 2,000 patients. This study met its primary end point and confirmed the importance of postoperative WBRT in preventing brain failure and death from neurologic causes.
In the Japanese Radiation Oncology Study Group JROSG-99-1 phase III trial of one to four lesions, the SRS-only arm experienced worse 1-year total brain recurrence rate (P <.001) and more frequently required salvage therapy (P <.001).26 Furthermore, the average time until Mini-Mental State Examination (MMSE) deterioration was significantly longer for the combined arm (16.5 vs. 7.6 months; P = .05).27 The main drawback of this study was the designation of overall survival as the primary end point. There is very little evidence that adjuvant WBRT after surgery is likely to improve overall survival. However, this study did demonstrate the importance of WBRT in decreasing brain failure, corroborating the findings of the Patchell et al.25 study.
Muacevic et al.28 randomized single brain metastasis patients (KPS ≥70, size ≤3 cm, stable systemic disease) to SRS alone versus resection plus WBRT. Those randomized to SRS alone experienced worse local (P = .06) and distant (P = .04) recurrences, but there were no differences in neurologic death rates or overall survival.
The results of EORTC-22952-26001 have been reported with the primary end point of evaluating duration of functional independence.29 In this study, patients with one to three brain metastases underwent local therapy with either surgery or SRS and were then randomized to the addition of WBRT versus observation. WBRT did not improve duration of functional independence or overall survival but was associated with a decreased 2-year local and distant brain relapse rate of roughly 30%, resulting in a 16% decrease in neurologic death. Although there was no difference in duration of functional independence between the two arms, the authors conclude that this is likely due to a variety of factors, including the subjective grading of the performance status, the routine MRI imaging rendering the majority of recurrences as asymptomatic, and the potential impact of systemic agents on performance status.
Recently, Chang et al.30 reported on a series of 58 patients with one to three brain metastases randomized to WBRT with or without SRS. The primary end point of the study was neurocognitive function, which was assessed using the Hopkins Verbal Learning Test-Revised at 4 months after therapy. They found that patients receiving combined therapy were more likely to have a decline in learning and memory function at 4 months compared with patients who did not receive WBRT. The median survival was 15.2 months for the SRS alone group and 5.7 months for the WBRT/SRS group (P = .003). However, the local (67% vs. 45%; P = .012) and distant (100% vs. 73%; P = .02) brain control were worse in the SRS alone group compared with the WBRT/SRS group. There are multiple criticisms of this study worth noting.31 First, because the primary end point was neurocognitive function, the authors did not stratify by baseline neurocognitive function or other factors known to impact neurocognition. Second, the authors chose a single test at a single time point. Ideally, a whole battery of tests should be performed at multiple time points to adequately assess the trend of something as complex as neurocognition. Most importantly, the combined arm inexplicably had a shorter survival, contrary to the four previously mentioned trials that demonstrated equivalent survivals. The median survival of 5.7 months was within 2 months of the primary end point mark, which classically falls within the time point of progressively worsening cognition seen in terminally ill patients.32,33 The superior survival in spite of inferior local and distant brain control is unprecedented and can possibly be explained by an improper randomization, which is possible in a small study.
In summary, four of the five phase III local with or without WBRT trials unequivocally show a meaningful benefit of WBRT in terms of preventing neurologic deaths or brain failure. It is difficult to ignore the level I evidence provided by these phase III trials. Adjuvant WBRT, therefore, should be considered the standard of care after local therapy with surgical resection or SRS.
TABLE 93.6 RANDOMIZED TRIALS OF POSTOPERATIVE/POSTRADIOSURGERY WHOLE-BRAIN RADIOTHERAPY
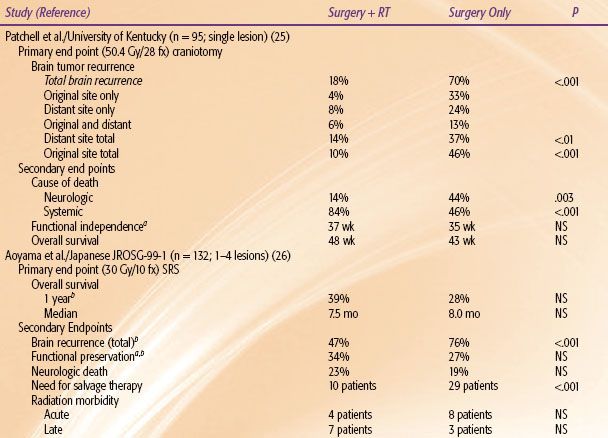
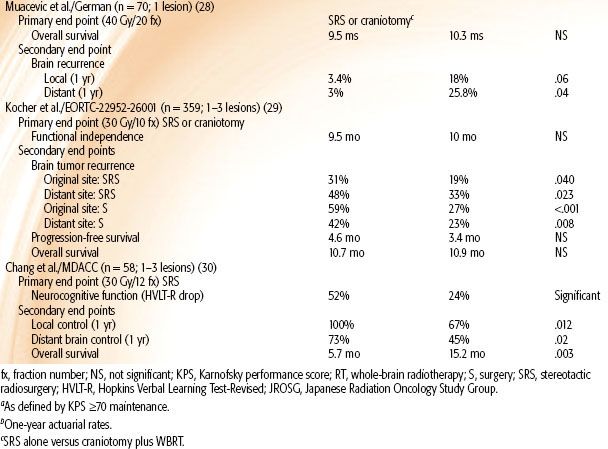
TABLE 93.7 SELECTED RANDOMIZED TRIALS OF RADIOSENSITIZERS IN BRAIN METASTASIS
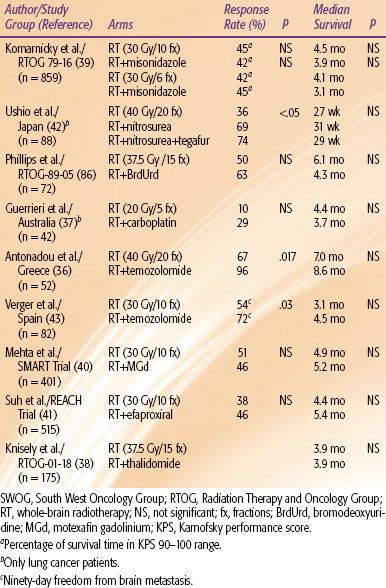
Repeat Whole-Brain Radiotherapy
Occasionally, patients will fail in the brain with multiple lesions after initial WBRT. Repeat WBRT should strongly be considered. Wong et al.34 reported on a series of 86 patients who underwent repeat WBRT. The median dose for the first course was 30 Gy, while the median dose for the second course was 20 Gy. A total of 70% experienced neurologic improvement, with 27% experiencing complete neurologic resolution while 43% had partial improvement after repeat WBRT. A retreatment dose of >20 Gy was associated with a significantly longer survival. Only one patient experienced dementia thought to be caused by radiation.
Son et al.35 similarly reported on a series of 17 patients who underwent whole brain reirradiation. The median RT dose for the first course of treatment was 35 Gy and for the second course 21.6 Gy. The median survival time for all patients after retreatment was 5.2 months. In patients with stable extracranial disease, the median survival time after retreatment was 19.8 months compared with 2.5 months in patients with progressive extracranial disease. Eighty percent of patients experienced improved symptomatology, but acute mild to moderate adverse reactions were seen in 70.5% of patients.
Repeat WBRT is relatively safe because a vast majority of patients have limited survival with recurrent or progressive brain metastases after initial WBRT. A minimum of 20 Gy in 1.8 to 2 Gy fractions should be given.
Concurrent Radiosensitizers
Although a majority of patients with brain metastases ultimately succumb to systemic progression, a significant percentage will die from neurologic progression. Multiple randomized trials of concomitant radiosensitizers have been performed in an attempt to optimize brain control (Table 93.7).25,36–43 No trial has demonstrated a survival advantage, although a few have demonstrated an increased response rate. The two trials with temozolomide show promise. Temozolomide is an oral alkylating agent with excellent CNS penetration. However, the findings of these two relatively small trials need to be confirmed in a larger trial. Concurrent temozolomide with WBRT should be considered in a patient with bulky brain metastases burden who is unlikely to become a SRS candidate. Otherwise, a patient should be treated with a concomitant radiosensitizer only on a prospective trial.
Causes of Neurocognitive Decline in Brain Tumor Patients
Historically, brain radiation has been frequently cited as the major cause of neurocognitive decline in cancer patients. One of the most misinterpreted studies on this subject is the Memorial Sloan-Kettering Cancer Center experience reported by DeAngelis et al.13 An 11% risk of radiation-induced dementia is reported in patients undergoing WBRT for brain metastasis. The 11% figure is very misleading. Of the 47 patients who survived 1 year after WBRT, 5 patients (11%) developed severe dementia. When these five patients were examined, all were treated in a fashion that would significantly increase the risk of late radiation toxicity (i.e., large daily fractions and concurrent radiosensitizer). Three patients received 5 Gy and 6 Gy daily fractions, while a fourth patient received 6 Gy fractions with concurrent Adriamycin. Only one patient received what is considered a standard radiation fractionation scheme (i.e., 30 Gy in 10 fractions), but this patient received a concurrent radiosensitizer (lonidamine). No patient who received the standard 30 Gy in 10 fractions WBRT alone experienced dementia.
The accuracy of the 11% dementia rate is further questioned by the nature of the statistical interpretation utilized. Although the study included 232 patients in the initial analysis, it only examined the 47 patients who survived at least 1 year. The principles of conditional probability dictate that the 11% risk is accurate only if a patient survives 1 year, which is significantly longer than most reported series. Therefore, a radiation-induced dementia risk of 2% (5 of 232) would reflect the true probability ab initio for patients presenting with brain metastasis. Indeed, in a separate study of a larger cohort, DeAngelis et al.44 estimated the risk of radiation-induced dementia to be 1.9% to 5.2% for all patients presenting with brain metastasis. In the authors’ opinion, this risk of dementia is not high enough to warrant withholding quality-of-life–prolonging WBRT.
Many have argued that the increased local control with adjuvant WBRT does not translate into a survival benefit, and that performing repeat SRS or deferring WBRT for recurrences is a reasonable approach. However, WBRT may actually improve neurocognition in a significant number of patients, and brain recurrence or progression is associated with a decrease in neurocognitive function.45 In a neurocognitive analysis of RTOG-91-04, Regine et al.46 demonstrated that approximately one-third of patients treated with WBRT experienced improvement in their MMSE; most importantly, those who had uncontrolled brain metastases had an average decrement of 6 points on the MMSE.
The DeAngelis et al.13 experience, as well as other studies that employed MMSE, did not utilize sophisticated neurocognitive testing. It is possible that subtle neurocognitive dysfunction may indeed result from WBRT. Recent studies that have used sophisticated neurocognitive testing clearly demonstrated that the brain tumor (presence, recurrence, and progression) has the greatest effect on neurocognitive decline. In the large phase III motexafin gadolinium study, the neurocognitive battery examined memory recall, memory recognition, memory delayed recall, verbal fluency, pegboard hand coordinate, and executive function.47 This study demonstrated that 21.0% to 65.1% of patients had impaired functioning at baseline before treatment with WBRT. Furthermore, patients who progressed in the brain after treatment experienced significantly worse scores in all of these individual tests.
There are now strong data that other factors, such as anticonvulsants, benzodiazepines, opioids, chemotherapy, craniotomy, and, most importantly, the brain tumor contribute significantly to the neurocognitive decline of patients with brain tumor.48–52
Anticonvulsants
Patients frequently present to the radiation oncologist already started on prophylactic anticonvulsants. This represents one of the most preventable causes of neurocognitive decline in brain tumor patients. Anticonvulsants are clearly known to impact negatively on quality of life and neurocognition. In a study of 156 patients with low-grade glioma (85% experiencing a seizure), Klein et al.50 correlated seizure burden with quality of life and neurocognitive function. This study convincingly demonstrates the significant correlation between the increase in the number anticonvulsants (even with lack of seizures) with the decrease in quality of life and neurocognitive function.
Based on four negative randomized trials, the American Academy of Neurology recommends that prophylactic anticonvulsants not be initiated in newly diagnosed brain tumor patients who have not experienced a seizure.53 It is safe to taper a patient off of anticonvulsants provided the patient has not experienced a seizure.
SPINAL CORD COMPRESSION
In the United States, more than 20,000 cases of metastatic spinal cord compression (MSCC) are diagnosed annually, and it is estimated to develop in approximately 5% to 14% of all cancer patients.54,55 MSCC is a devastating complication of cancer. It is considered a true medical emergency, and immediate intervention is required. Even with aggressive therapy, results can often be unsatisfactory. Although most patients with MSCC have limited survival, up to one-third will survive beyond 1 year.56 Therefore, aggressive therapy should always be considered to preserve or improve the quality of life.
Pathophysiology
MSCC develops primarily in one of three ways: (a) continued growth and expansion of vertebral bone metastasis into the epidural space; (b) neural foramina extension by a paraspinal mass; or (c) destruction of vertebral cortical bone, causing vertebral body collapse with displacement of bony fragments into the epidural space. Although complex, the most significant damage caused by MSCC appears to be vascular in nature. Epidural tumor extension causes epidural venous plexus compression, which leads to edema of the spinal cord. This increase in vascular permeability and edema cause increased pressure on the small arterioles. Capillary blood flow diminishes as the disease progresses, leading to white matter ischemia. Prolonged ischemia eventually results in white matter infarction and permanent cord damage.57
Clinical Presentation, Diagnosis, and Prognosis
The vast majority of patients with MSCC have a cancer diagnosis history. In a review by Fuller et al.,58 of more than 1,000 patients with MSCC, the most common tumor types are breast cancer (29%), lung cancer (17%), and prostate cancer (14%). This reflects the high natural incidence of these tumors. New-onset back pain in cancer patients needs to be taken seriously and worked up. Even without a prior cancer diagnosis, MSCC should be suspected in anyone who presents with progressively worsening back pain, incontinence, or paraplegia, especially in the high-risk population such as longtime smokers. The most common level of the MSCC involvement is in the thoracic spine (59% to 78%), followed by lumbar (16% to 33%) and cervical spine (4% to 15%), while multiple levels are involved in up to half of the patients.58–60 Back pain is the most common presenting symptom (88% to 96%), followed by weakness (76% to 86%), sensory deficits (51% to 80%), and autonomic dysfunction (40% to 64%).59,61–65
MRI is the standard modality for spine imaging. It has a very high sensitivity (93%), specificity (97%), and accuracy (95%) in diagnosing MSCC.66,67 Because patients can have synchronous, multifocal MSCC, an MRI of the entire spine with and without contrast should be promptly performed in anyone suspected of having MSCC.68 High-resolution CT scan or CT myelogram of the spine should be performed for those with contraindications to MRI.
Prognostic factors predicting survival are generally similar to patients with brain metastasis, as discussed above. In terms of predicting ambulatory outcome, one of the most important factors is the rapidity of symptom onset. Other important prognostic factors include radiosensitive histology (e.g., multiple myeloma, germ cell tumors, small cell carcinoma) and pretherapy ambulatory function. In a prospective study of 98 patients with MSCC reported by Rades et al.,69 the single strongest predictor for ambulatory status after therapy on multivariate analysis was time to development of motor deficits before radiation (P <.001) from the start of any symptoms. This cohort was separated into three groups according to the time to motor deficits before radiation therapy: 1 to 7 days (group A), 8 to 14 days (group B), and >14 days (group C). The ambulatory rates after therapy for groups A, B, and C were 35%, 55%, and 86% (P <.001), respectively. The symptom improvement rates for groups A, B, and C were 10%, 29%, and 86% (P = .026), respectively. The other factor significant on the multivariate analysis for posttherapy ambulatory status was favorable histology (P = .005), and there was a trend regarding pretherapy ambulatory status (P = .076). Acute, rapid deterioration is predictive of irreversible spinal cord infarction. Only 10% of the patients in group A had symptom improvement; therefore, prompt diagnosis and treatment of MSCC are essential.
Corticosteroids
Corticosteroids must be started as soon as possible in anyone suspected of having MSCC, even before radiographic diagnosis, because this can be rapidly discontinued with a negative diagnosis. Corticosteroids effectively decrease cord edema and serve as an effective bridge to definitive treatment. Although multiple retrospective studies have demonstrated their clinical efficacy, Sorensen et al.70 reported the only randomized controlled study (n = 57) on the utility of high-dose corticosteroids before definitive radiotherapy in MSCC from solid tumors. The treatment arm consisted of 96 mg of IV bolus of dexamethasone followed by 96 mg oral per day for 3 days and a 10-day taper versus no corticosteroid therapy. This study demonstrated 3-month and 6-month ambulatory rates of 81% versus 63% and 59% versus 33% (P <.05), respectively, in favor of high-dose dexamethasone.
The optimal maintenance dose of corticosteroids is unknown. Vecht et al.71 reported the only randomized study (n = 37) comparing corticosteroid doses in patients with MSCC, but this study only evaluated the IV loading dose. It compared IV loading doses of 10 mg versus 100 mg, followed in both arms by the same oral regimen of 16 mg per day. Both arms demonstrated significant reductions in pain from baseline (P <.001); however, there was no difference between the two arms with respect to pain reduction, ambulation, or bladder function.
Very high doses of corticosteroids are associated with significant side effects. The Sorensen et al.70 phase III study reported an 11% incidence of serious side effects for patients in the treatment arm, while Heimdal et al.72 reported a 14.3% incidence of serious gastrointestinal (GI) side effects in 28 consecutive patients treated with 96 mg of IV dexamethasone per day. The toxicities in the Heimdal et al.72 report included one fatal ulcer hemorrhage, one rectal bleeding, and two bowel perforations. Subsequently, the dexamethasone dose was decreased to 16 mg per day for the next 38 consecutive patients, and there were no incidences of serious side effects (P <.05). Most importantly, the ambulatory rates were not different between the two dexamethasone doses.
Based on these data, a loading disc of 10 mg of IV dexamethasone followed by a maintenance dose of 4 to 6 mg every 6 to 8 hours should be sufficient before being tapered. Patients can be safely switched to a oral regimen after 24 to 48 hours because there is good oral bioavailability of corticosteroids. Furthermore, patients should be started on a PPI for GI prophylaxis. Although here has been no randomized trial utilizing PPIs in patients receiving corticosteroids, there have been multiple phase III studies demonstrating the protective effects of PPIs against peptic ulcers in patients receiving chronic nonsteroidal anti-inflammatory drugs (NSAIDs).73 NSAIDs and corticosteroids both cause GI mucosal injury by decreasing mucosal-protective prostaglandin levels. Therefore, it is not an unreasonable extrapolation to assume that PPIs provide a similar mucosal protective effect with corticosteroids, especially considering that the morbidity of GI toxicity can be life-threatening. Chronic use of oral antacids should be avoided because the short half-life of these agents requires a large amount to be ingested per day. Increased popularity of over-the-counter antacids as a means of calcium supplementation has been recognized as an important cause of the recent increase in milk-alkali syndrome and resultant hypercalcemia.74,75
Surgery
Radiation for nonradiosensitive tumors typically takes several days to have an effect and does not stabilize the spine, while surgery allows for immediate cord decompression and provides an opportunity to stabilize the spine intraoperatively. At some institutions, surgery was used infrequently because several retrospective studies and one small randomized study showed no benefit to surgery plus radiation over radiation alone. Young et al.76 randomized 29 patients with MSCC to decompressive laminectomy followed by radiation versus radiation alone. Although this trial showed no benefit to surgery in terms of pain relief, ambulation, or sphincter function, it is difficult to draw any conclusion because of the small sample size. All of these studies used posterior laminectomy in conjunction with radiotherapy; however, most of the lesions in MSCC involve the anterior portion of the vertebral body.54 Therefore, a laminectomy does not effectively relieve the compression and may actually worsen the stability of the spine.
Recently, several authors have advocated the use of direct surgical decompression, tumor debulking, and spinal stabilization via instrumentation to improve on the results from radiation alone. Patchell et al.77 reported the first phase III randomized trial testing the efficacy of direct decompressive surgery in patients with MSCC (Table 93.8). The study compared radiation alone (standard 30 Gy in 10 fractions) versus decompressive and stabilization surgery within 24 hours of diagnosis followed by the same radiotherapy (within 2 weeks of surgery). The trial was terminated early when early-stopping rules were met regarding the primary end point of ambulation after treatment. This trial definitively demonstrated an advantage to surgery for every end point at statistically significant levels. For nonambulatory patients, the combined treatment patients had a significantly higher chance of regaining the ability to walk after therapy. Maintenance of continence, maintenance of American Spinal Cord Injury (ASIA) and Frankel scores (measures of spinal function after injury), median overall survival, and median mean daily dexamethasone and morphine equivalent doses all favored the surgery arm.
If operable, patients should undergo surgical decompression and stabilization followed by radiotherapy. Even for radiosensitive tumors, surgery can often stabilize the spine. Therefore, all patients with MSCC should be evaluated by a surgeon. Effective multidisciplinary teamwork is critical to the rapid evaluation and management of patients with MSCC.
TABLE 93.8 KEY FINDINGS OF A PHASE III STUDY (77) OF PATIENTS WITH METASTATIC SPINAL CORD COMPRESSION
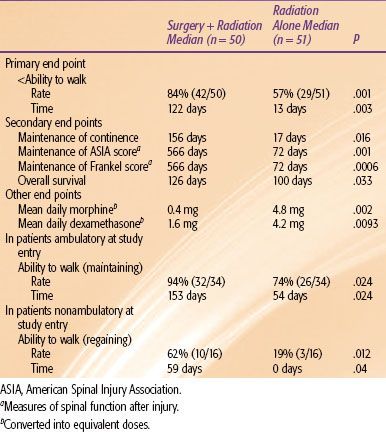
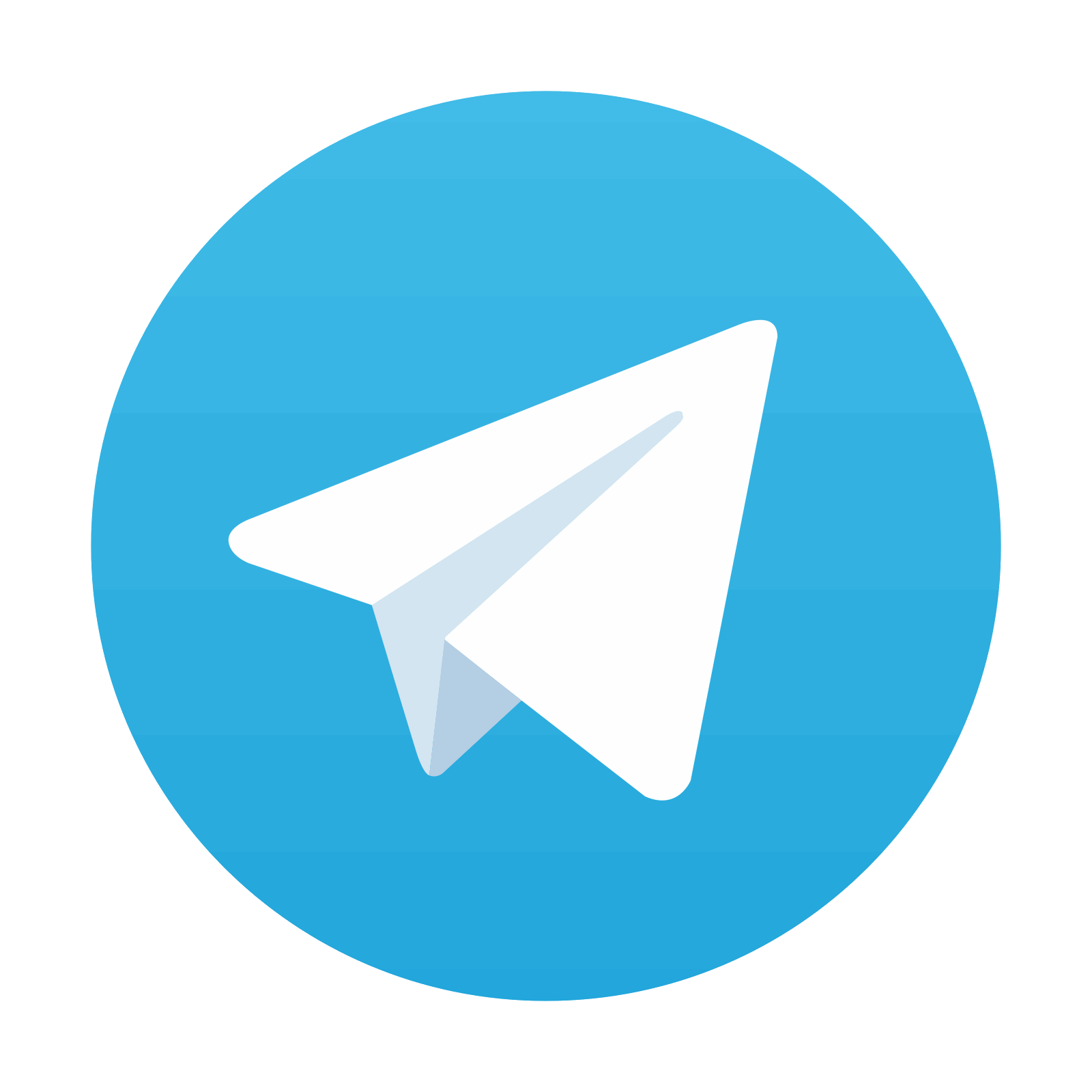