Palliation of Bone Metastases
BACKGROUND AND INCIDENCE
Metastatic disease to the bone is a common cause of pain and other significant symptoms that are detrimental to quality of life. The exact incidence of bone metastases is difficult to determine, but estimates are that >100,000 people in the United States will develop osseous metastatic disease annually.1,2 The incidence of bone metastases varies significantly, depending on the primary site, with breast and prostate cancer accounting for up to 70% of patients with metastatic disease.3 Bone metastases may be found in up to 85% of patients dying from breast, prostate, or lung cancer. Other primary sites with a propensity for bone metastases include thyroid, melanoma, and kidney. On the other hand, gastrointestinal sites of primary malignancy give rise to bone metastasis in only 3% to 15% of patients with metastatic disease.4 Some hematologic malignancies, including myeloma and lymphoma, can also cause significant pain and bone destruction.
The ultimate prognosis for patients with bone metastases is poor, with median survival typically measured in months rather than years. Overall survival depends on the primary site and the presence or absence of visceral metastases. Patients with bone metastases from lung cancer have short median survival durations of 6 months. However, patients with bone metastases from breast or prostate primary sites may have significantly longer survival times. In patients with bone-only metastatic prostate or breast cancer, median survivals of 2 to 4 years have been reported.3,5,6 Whether the survival time is only a few months or extends to multiple years, these patients will often require active treatment because of pain, difficulty with ambulation and immobility, hypercalcemia, pathologic fractures, neurologic deficits, anxiety, depression, spinal cord or nerve root compression, fatigue, insomnia or sleep disturbances, and general deterioration of quality of life.4,7,8
The axial skeleton is the most common site of bone metastasis, with metastasis most frequently occurring in the spine, pelvis, and ribs. The lumbar spine is the most frequent site of bone metastasis.9–12 In the appendicular skeleton, the proximal femurs are the most common site of metastatic disease, and humeral lesions also occur frequently. The acral sites (feet and hands) are rarely involved. Certain skeletal sites are associated with specific areas of bone metastases. For example, scapular metastases are seen more frequently from renal primaries.13 Involvement of the skull is more common with breast primaries. The distal appendicular skeleton (tibia, fibula) and acral sites (especially the hands) are more common with lung primaries, and involvement of the toes is seen more commonly with genitourinary primaries (Fig. 94.1).
The most common symptom of bone metastases is slowly progressive, insidious pain that is fairly well localized. The pain may be worse at night. Pain from the femur or acetabulum may worsen with weight bearing or ambulation. In contrast, pain from the inferior ischium or sacrum may be worse with sitting but less bothersome with ambulation. Although the pain is frequently localized, pain may radiate to other areas. This is most frequently seen with pain in the lower back, pelvis, or hips that may radiate down the legs. Pain that radiates does not necessarily indicate nerve impingement because radicular pain can also be caused by spasm of muscles that originate or insert near the area of disease (e.g., pain in the hip radiating to the knee).
FIGURE 94.1. Radiograph of right foot of a patient with metastatic transitional cell carcinoma of the bladder. Note the destruction of the second metatarsal.
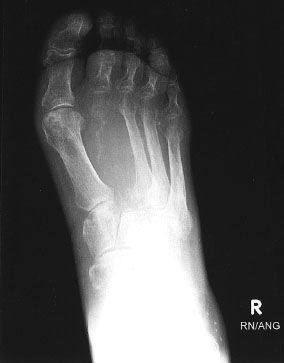
PATHOPHYSIOLOGY
There are primarily three types of cells within mature bone: osteocytes, osteoblasts, and osteoclasts. Osteoblasts originate from osteogenic cells, found in the periosteum or endosteum. The osteogenic cells differentiate into osteoblasts when there is a mechanical or chemical stimulus for remodeling or repair. The osteoblasts build bone by depositing collagen type I into the extracellular space. An inorganic complex of calcium and phosphate (hydroxyapatite) is laid down within this organic matrix to provide the strength and density of the bone. The osteoblasts then mature into osteocytes, which maintain the bone structure. Osteoclasts are multinucleated giant cells that originate from pluripotent hematopoietic bone marrow cells and are adherent to the bone surface.14 These cells create an acidophilic environment that causes dissolution of the hydroxyapatite crystals and proteolysis of the bone matrix.
The differentiation and activation of osteoclasts occurs because of the effects of a group of proteins that are related to tumor necrosis factor, including osteoprotegerin, receptor activator of nuclear factor-κB (RANK), and the RANK ligand (RANKL). Osteoblasts and stromal cells express RANKL, and activated T cells may also release RANKL. The RANKL binds to the RANK receptor on osteoclast precursors, which then induces the formation of mature osteoclasts. Osteoprotegerin is a decoy receptor for RANKL and inhibits the differentiation and activation of osteoclasts.14 The destruction of bone by osteolytic metastases is mediated by the osteoclasts, not by the tumor cells. However, the factors that activate the osteoclasts are likely produced by the tumor cells, including RANKL, interleukin-1, interleukin-6, and macrophage inflammatory protein 1α. The mechanisms for osteoblastic activation are not clearly delineated, but it appears that bone resorption occurs first even in osteoblastic metastases from prostate cancer.15
Normal bone is constantly being remodeled in a cycle lasting about 120 to 200 days (3 to 6 months). For the first 20 to 40 days of the cycle, the bone is resorbed by osteoclasts. The bone is then rebuilt by osteoblasts during the next 100 to 150 days.16
The structure of the bone changes during growth and development. All bones are immature, woven bone at the time of birth. Woven bone is more cellular, with no organized orientation to the collagen fibers of the bone. As the woven bone is absorbed, it is replaced by lamellar bone. The lamellar bone is organized circumferentially around neurovascular canals. This cylindrical structure provides much more strength than the haphazard orientation of woven bone. Most adult bone is lamellar.
The strength of bone is provided primarily by cortical bone, which is the dense, compact bone found in the diaphysis of long bones and along the exterior surfaces of cuboidal bones. Cortical bone comprises most of the mass of the skeletal system and provides most of the strength of the skeleton. Trabecular bone is the spongy, cancellous bone found in the center of cuboidal bones and in the center of the metaphysis and diaphysis of long bones. There is much more rapid remodeling of trabecular bone, with replacement of 25% of trabecular bone per year, compared with 3% of cortical bone.17
Long bones consist of the epiphysis, metaphysis, and diaphysis. The diaphysis is the long shaft of the bone. The epiphyses are at the ends of the bone. These are composed of hyaline cartilage initially, which becomes ossified during puberty. The metaphysis is the area between the epiphysis and diaphysis. The metaphysis is an area of rapidly growing trabecular bone.
Metastases to the bone most often occur in the red marrow, which is found in highest concentration in the axial skeleton. This most often occurs by hematogenous spread but may occur by direct extension as well. Involvement of adjacent bone by direct extension (e.g., mandibular involvement from an oral cavity cancer) does not necessarily imply that there is a higher likelihood of distant bone metastases, and its management is very different from that of bone metastases from hematogenous spread. The predilection of certain tumor sites to metastasize to bone may be related to local growth factors in the bone such as transforming growth factor-β, insulin-like growth factors I and II, fibroblastic growth factors, or platelet-derived growth factors, preferential adherence to endothelial surfaces in certain bones by cell adhesion molecules, or chemotactic attraction from bone cells by osteocalcin or type I collagen.4,14,18 The relatively high proportion of hematogenous metastasis to bone compared with other sites in the body cannot simply be explained by blood flow, which is >30 times greater in lung than in red bone marrow.19
Bone metastases are often described as either osteolytic or osteoblastic, but these are different representations of abnormalities in the normal bone-remodeling process. Breast and lung cancers more commonly cause osteolytic-appearing lesions, and lesions caused by prostate and thyroid cancers more often have an osteoblastic appearance. However, only myeloma is associated with purely osteolytic lesions.14 Most other tumors have a combination of osteolytic and osteoblastic components. Even in osteoblastic-appearing prostate cancer metastases, increased bone resorption does occur.
The mechanism of pain from bone metastases is not clearly understood. Possible mechanisms include mechanical instability, irritation of periosteal stretch receptors, tumor-directed osteoclast-mediated osteolysis, tumor cells themselves, or tumor-induced nerve injury, production of nerve growth factor, or stimulation of other cytokine receptors.2,3,20,21 Because the mechanisms of pain may be multifactorial, a combination of therapies may be superior to any one therapy alone.20
EVALUATION
The physical examination is an important step in evaluating a patient with bone metastases. The physical examination may help make decisions regarding appropriate subsequent imaging studies. Firm palpation will often elicit the specific area of pain, with “point tenderness” often pointing directly to the affected area in the bone. It is important to carefully evaluate the entire skeletal system with examination because intense pain at one site often masks subjective reports of pain at other sites. A careful physical examination may reveal hidden pain in other locations. A thorough neurologic examination is also important, especially in patients with spinal metastases, to carefully evaluate for the possibility of spinal cord, cauda equina, or nerve root compression.
For symptomatic patients with point tenderness, plain radiographs are typically the most appropriate first imaging study. Such radiographs are easy to obtain and inexpensive. The appearance of bone metastases on x-rays varies depending on the primary site and histology. Most bone metastases from lung cancer and breast cancer appear osteolytic, whereas most from prostate cancer appear osteoblastic (Fig. 94.2). However, nearly all bone metastases have components of both osteolytic and osteoblastic processes. The primary disadvantage of plain radiographs is that small lesions are rarely seen. Approximately 30% to 50% of the bone mineral content must be lost before the lesion will be apparent on x-rays.
Technetium-99m bone scintigraphy (nuclear medicine bone scan) is the best method for screening patients at risk for bone metastasis and is useful to evaluate the extent of metastatic disease in the bone. Bone scintigraphy is an indicator of osteoblastic activity. Because multiple myeloma is frequently purely osteolytic, bone scans are less useful for evaluating extent of disease in myeloma. Bone scintigraphy is not specific for metastatic disease, and positive findings must often be confirmed using other imaging studies. A confirmatory study is especially important in a weight-bearing bone such as the proximal femur. False-positive readings may be seen in areas of arthritis, trauma, or Paget’s disease. In addition, the osteoblastic activity in healing bone after treatment may give the appearance of progressive disease. False-negative readings may occur in fast-growing, highly aggressive tumors, especially if these are mainly osteolytic.
Computed tomography (CT) scans are more sensitive than plain radiographs and may be better able to localize the lesion within the bone. However, CT scans are more expensive, more time-consuming, and may not be useful as a screening tool for skeletal metastasis. The CT may be useful in defining the extent of cortical destruction and helping to assess the risk of a pathologic fracture.22 In addition, the CT scan may be used to guide needle biopsies to obtain a tissue diagnosis. CT scans have limited usefulness in detecting marrow involvement but are much better than plain radiographs at evaluating soft tissue extension of disease.
Magnetic resonance imaging (MRI) is better than plain radiography or nuclear medicine bone scintigraphy at assessing the involvement of trabecular bone (red marrow), especially in the vertebral bodies. The findings are typically best seen on T1 contrast-enhanced images and short-tau inversion recovery (STIR) images. Metastatic prostate cancer is visible as high-intensity lesions on the STIR images and is visible prior to its appearance on bone scintigraphy.23 In addition, MRI scans are useful in determining the involvement of neurovascular structures. MRI scans are not useful as a screening tool for bone metastases. However, MRI scans may be more sensitive than bone scintigraphy in the vertebral body region (Fig. 94.3). The sensitivity of MRI scanning has been reported as 91% to 100%, compared with 62% to 85% for bone scintigraphy.23,24 In addition, MRI images can help distinguish whether a vertebral body compression fracture is from malignancy or from osteoporosis.
FIGURE 94.2. Anteroposterior (A) and lateral (B) spine radiographs from a patient with metastatic prostate cancer. There are osteoblastic lesions seen in T12 and L1.
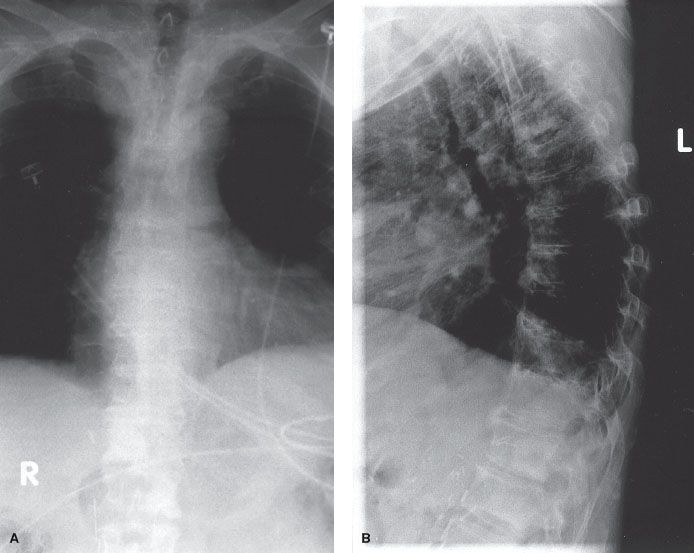
Positron emission tomography (PET) scanning evaluates areas of increased metabolic activity, most commonly using the 18-fluorodeoxyglucose (FDG) isotope. These scans are useful in detecting osteolytic bone metastases but are less sensitive for osteoblastic metastases. In addition, precise determination of the location of lesions is difficult with PET scans, but the use of simultaneous CT scans allows for much better localization of the abnormal FDG uptake.25 PET scans may be useful as a whole-body screening tool.25,26 Comparative studies have shown PET scans to be more sensitive than Tc-99m scintigraphy or whole-body MRI scans in detecting bone metastases.27,28 There may be limitations in the sensitivity of PET scanning in certain areas such as the skull, where the intense physiologic uptake from the adjacent brain parenchyma may obscure small skull metastases.
FIGURE 94.3. Nuclear medicine bone scan (A) and sagittal T1 magnetic resonance image (MRI) scan (B) from a woman with metastatic breast cancer. The bone scan shows the abnormality at L1, but the MR shows lesions at multiple levels, including the pedicles of T12, L3, and L4 (arrows).
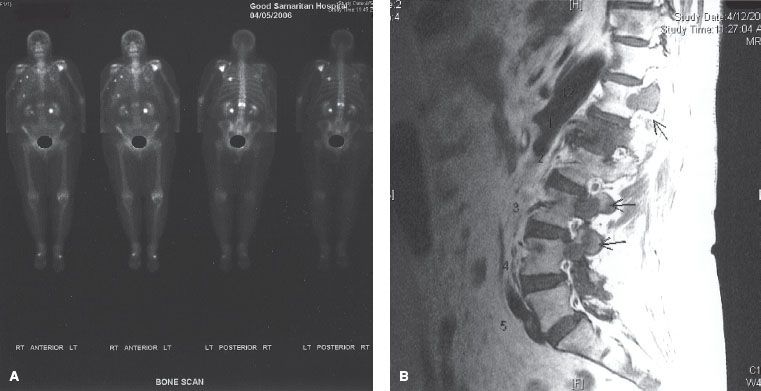
PAIN MANAGEMENT
The majority of patients with bone metastases will experience pain during their disease course, and pain control can significantly improve their quality of life. Pain management may be achieved either by debulking disease using cytotoxic therapy or by symptomatic control with pharmacologic interventions.
Despite increasing understanding about the effective treatment of pain, patients with pain from bone metastases frequently have inadequate pain management. Barriers to pain treatment include physician underestimation of the patient’s pain and reluctance by the patient to report pain.29 There is a significant discrepancy between the physician estimate of pain and the pain level reported by the patient.30 The use of a validated pain scale, such as the Brief Pain Inventory, gives the patient an opportunity to describe the severity of pain and the interference of pain with function in a manner that can be understood both by the patient and the physician.29 This also allows for comparisons of pain levels over time, to better assess the effectiveness of treatments.
Pain control can be achieved in the majority of patients using the World Health Organization analgesic ladder. Step I uses nonopioid analgesics such as acetaminophen or nonsteroidal anti-inflammatory drugs; step II uses weak opioids such as codeine; step III uses strong opioids such as morphine. These medications are increased as necessary until the patient is free of pain. Typically, the medications are given on a routine schedule (“by the clock”) rather than waiting until a certain level of pain is reached (“on demand”). Using this schedule, 70% to 76% of patients will have good pain relief.31,32 Adjuvant medications such as gabapentin, pregabalin, or amitriptyline may be added for neuropathic pain. Antianxiety or antidepressant medications may also be of benefit in selected patients.
The opioid-based pain medications frequently cause constipation and may cause nausea. Patients using opioid medications should routinely be administered a fiber medication with or without a stool softener to minimize constipation. Other side effects of the opioid analgesics may include sedation, mental status changes, and mood changes.
SURGICAL MANAGEMENT
Surgical management of bone metastases is performed primarily to prevent or treat pathologic fractures. The goals of surgical intervention are to prevent or relieve pain, improve motor function, and improve overall quality of life. Treatment techniques are simpler and more effective when the procedure is performed prophylactically for an impending fracture rather than after the occurrence of a pathologic fracture. The risk of pathologic fracture depends on multiple factors, including location and extent of the lesion; whether the lesion is osteolytic, osteoblastic, or mixed; and the primary cancer site.
Fractures of the weight-bearing bones are the most likely to cause significant functional deficits. The proximal femur may have a higher propensity for fracture than other sites, but some authors have suggested that the fracture risk in upper limbs is similar to the risk in the femur. Whether or not there is a difference in the risk of fracture, the peritrochanteric femur is the site most likely to cause serious morbidity, and therefore the threshold for prophylactic intervention should be relatively low. The femur accounts for 65% of pathologic fractures requiring surgical intervention.33 The humerus and vertebral bodies are also sites that require special attention because of the potential functional deficits from pathologic fractures.
The size of the bone metastasis is an important predictor of risk of fracture, especially with regard to the extent of cortical destruction. Various models have been used to predict the risk of pathologic fracture based on the size of the lesion. In series using plain radiographs, lesions ≥2.5 cm in the cortex of the femur were significantly more likely to fracture.34 The proportion of cortical destruction is important as well. The risk of pathologic fracture of the femur begins to significantly increase when there is destruction of >50% of the cortex; the risk of fracture is 80% when >75% of the cortex is destroyed.35 The location within the bone is important as well. An experimental model has shown that the greatest reduction in strength of the femur occurs with lesions in the inferior and medial aspect of the femoral neck, and posterior lesions have the least impact.36 The use of CT scans may offer more accurate assessment of the bone strength. Femoral lesions with axial cortical destruction >30 mm had a 23% risk of pathologic fracture, compared with 3% risk of fracture for cortical destruction ≤30 mm.37
A scoring system proposed by Mirels38 has a 12-point scale based on the location of the lesion, pain, extent of cortical destruction, and radiographic appearance (Table 94.1). The risk of fracture is 15% for a score of 8 and 33% for a score of 9. He proposed that prophylactic fixation is indicated for a score of ≥9.
The decision to proceed with surgery should be based on a number of factors, which include but are not limited to the estimated risk of pathologic fracture. For patients with a very limited life expectancy, surgery may not be indicated even if the risk of pathologic fracture is relatively high.33 Clinical prediction of survival may be more accurate than relying on specific parameters such as diagnosis (primary site), performance status, number of bone metastases, presence of visceral metastases, and hemoglobin level.39
Fractures of the femoral neck can be managed either by total hip arthroplasty (which replaces both the femoral head and acetabulum) or a proximal femoral endoprosthesis alone.33 Fractures of the intertrochanteric area may be managed by open reduction and internal fixation without the use of a prosthesis. This may allow for better long-term gait because of preservation of the hip flexor and adductor strength.33 Lytic disease that extends below the intertrochanteric area is treated with a long intramedullary rod that provides stability throughout the length of the femur (Fig. 94.4). If there is significant destruction of the greater trochanter and femoral neck or head in addition to subtrochanteric involvement, a prosthetic replacement would be more appropriate than a reconstruction nail.40 Fractures of the distal femur may be managed either with a plate and compression screw or with an intracondylar nail and screws augmented by intramedullary methylmethacrylate cement. The latter method may reduce the risk of late failure of the repair, especially in patients receiving postoperative radiation therapy.33
Repair of pathologic fractures of the humerus may be more problematic because of the small intramedullary canal and because of the proximity of the radial nerve, which may require extensive dissection.33 Pathologic fractures of the proximal humerus will frequently require prosthetic replacement.33 Fractures in the diaphysis may be repaired with a compression plate and screws. An alternative is to use a segmental diaphyseal replacement prosthetic device, which involves a prosthetic device than can be cemented into an allograft.40 Supracondylar fractures are difficult to manage because the shape of the bone is not conducive to a plate and screws; these lesions may require intramedullary rods inserted in a retrograde manner through both the medial and lateral condyles, supplemented by intramedullary cement.33
Most pathologic fractures of the pelvis do not require surgical intervention, except for those involving the acetabulum.33 Repair of acetabular fractures may involve the use of a total hip acetabular prosthesis, but more extensive lesions may require reconstruction that transfers the load-bearing stresses into more structurally intact bone in the iliac bone or sacroiliac joint.33
TABLE 94.1 MIRELS’ SCORING SYSTEM OF PREDICTION OF PATHOLOGIC FRACTURE RISK
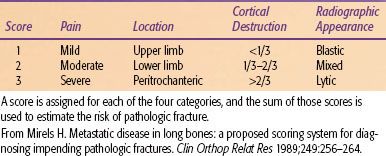
INTERVENTIONAL TECHNIQUES
Vertebroplasty is an effective method of palliating pain from vertebral body metastases, even in patients who have received prior radiotherapy.41 Most patients experience pain relief within 48 hours. The procedure involves percutaneous injection of methylmethacrylate under CT or fluoroscopic guidance. Retropulsion of bone, epidural tumor, or collapse of the bone to less than one-third of its original height are relative contraindications to percutaneous vertebroplasty because of the risk of extrusion of the cement into the spinal canal, potentially causing neurologic complications. In some patients with epidural tumor, percutaneous vertebroplasty can be performed safely and effectively with a relatively low risk of serious complications.42,43 However, the procedure should be considered with some caution; in a systematic review of 987 patients, there were 5 deaths and 19 other serious complications.44
Kyphoplasty involves percutaneous placement of a balloon-like device into a symptomatic spinal metastasis (most commonly into a fractured or compressed vertebral body).45 The balloon is then inflated to restore the height of the vertebral body, and methylmethacrylate is subsequently injected into this cavity. This procedure may provide significant relief of pain and improve overall functioning, especially in patients with mechanical instability of the vertebral body.46,47 Kyphoplasty may be a better option than vertebroplasty in patients with vertebral wall deficiency.46
An ablative procedure is frequently coupled with vertebroplasty or kyphoplasty.48 Radiofrequency ablation (RFA) may be used to ablate the tumor but is most effective for tumors that are osteolytic or mixed osteolytic and blastic. The RFA may not be as effective in tumors that are primarily sclerotic. Cryoablation may be used for larger lesions or those that are sclerotic. For both of these procedures, special attention to cord and nerve temperatures is required to minimize the risk of complications.
SYSTEMIC TREATMENT
The rationale for using systemic therapy in the management of bone metastasis is compelling. The pathophysiology of bone metastasis involves hematogenous dissemination, and most patients with bone metastasis suffer from multiple synchronous sites of disease. In theory, administering systemic cytotoxic therapy should deliver palliative benefit by simultaneously addressing all sites of bone metastasis. A localized therapy such as external-beam radiation may be most appropriate for palliation if symptoms are localized. On the other hand, systemic chemotherapy may offer palliative benefit if symptoms are diffuse or constitutional and disease is widespread.
Measurement of response to systemic therapy has generally been with the same criteria used for solid metastatic tumors: a measurable radiographic change. This works well for lung and liver metastasis but not as well for bone metastasis. For bone metastases, the definition of a complete response is complete disappearance of all lesions on radiographs for at least 4 weeks. This is unlikely to occur even if all tumor cells are eradicated. A partial response requires some recalcification of lytic lesions, which may not be evident for 6 months or more.49 PET scans may be more accurate at assessing response in a timely manner but are too expensive to be used as a routine follow-up evaluation for bone metastases. Markers of bone resorption may be a good way to detect response to therapy but are not clinically available at this time. Response to therapy for other modalities (i.e., radiotherapy, bisphosphonates) is measured in terms of pain relief and quality-of-life measures. Unfortunately, there is not much literature on accurate and reliable response criteria to palliative systemic therapy for bone metastasis. Most of the studies of chemotherapy for metastatic disease involve patients with visceral as well as osseous metastases. The responses in terms of pain are typically reported for all patients, not just those with bone metastases. There is more information regarding response to chemotherapy for patients with metastatic prostate cancer because this more frequently involves bone-only metastatic disease. The chemotherapy drugs are frequently given with bisphosphonates or corticosteroids, which may affect the response rates.
FIGURE 94.4. Anteroposterior radiograph (A) of a diaphyseal fracture of the femur in a patient with diffuse large-cell lymphoma. Intraoperative fluoroscopic views of the proximal (B), mid-shaft (C), and distal (D) femur after internal fixation with a trochanter fixation nail (TFN) nail.
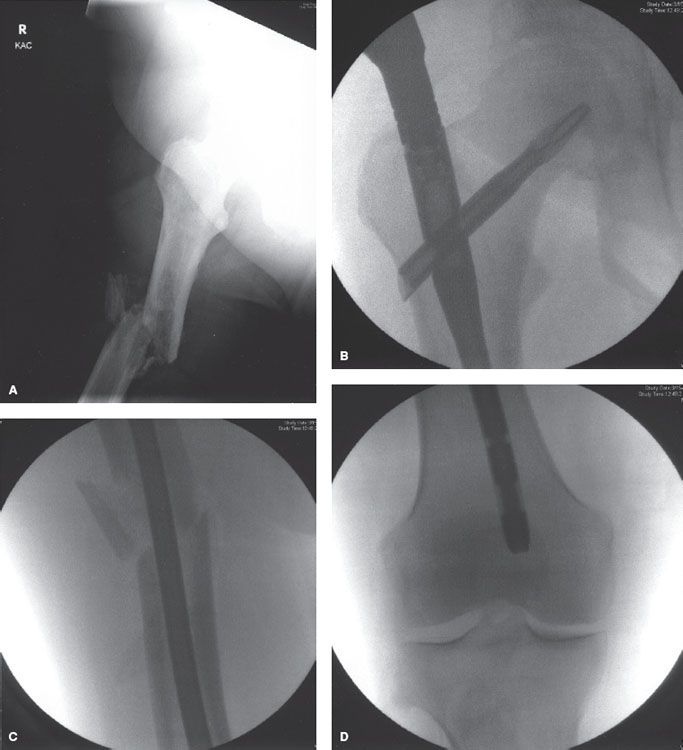
Tannock et al.50 recommended using more relevant endpoints of palliation. They performed a randomized trial of mitoxantrone plus prednisone versus prednisone alone for patients with hormone-refractory prostate cancer and pain. Improvement in pain was seen in 29% of patients receiving the chemotherapy, compared with 12% of those who received prednisone alone. In a subsequent study, they compared mitoxantrone and prednisone plus or minus clodronate for a similar group of patients. Most had mild pain at study entry (160 of 209; 77%), and the remainder had moderate pain scores. The patients with moderate pain who received chemotherapy and clodronate had a 58% response rate (≥2-point improvement in pain score) compared with 26% for those who received chemotherapy alone. The median duration of pain response was 6 months. The TAX327 phase III trial was conducted in 1,006 men with hormone-resistant metastatic prostate cancer and randomized patients to receive prednisone combined with either docetaxel or mitoxantrone. The docetaxel arm showed an improvement in overall survival, lower cancer-induced bone pain, and better objective tumor response.51
In patients with bone metastasis, there is seldom reason to combine chemotherapy with concurrent radiation therapy because of the potential for increased toxicity when both modalities are delivered concurrently.
A number of hormonal therapies are available in the management of metastatic prostate and breast cancer. In properly selected patients, hormonal therapy has the potential for providing excellent palliation of metastatic disease with limited morbidity. In 1984, the Medical Research Council started a prospective, randomized trial in which 938 patients who either had asymptomatic metastatic prostate cancer or were not medical candidates for definitive therapy were randomized to immediate versus delayed hormone ablation therapy. Hormone ablation was achieved using either a leutinizing hormone–releasing hormone agonist or by orchiectomy. This study showed that immediate versus delayed initiation of hormone ablation therapy in the subset of patients with metastatic prostate cancer helped to prevent serious complications from metastatic disease. Serious complications including pathologic fracture, spinal cord compression, development of extraskeletal metastases, and ureteral obstruction were twofold more frequent in patients whose hormonal therapy was deferred compared with patients who received immediate hormone ablation therapy.52
The bisphosphonates are pyrophosphate analogs that bind to calcium phosphate with high affinity and are potent agents affecting bone resorption.53 There is emerging evidence that the bisphosphonates also induce apoptosis in cancer cells.54 Clodronate is a first-generation, non–nitrogen-containing bisphosphonate. Clodronate has a high affinity for bone mineral and is subsequently taken up into activated osteoclasts during bone resorption, thereby ensuring high concentrations within osteoclasts.55 The nitrogen-containing bisphosphonates inhibit the key enzyme farnesyl diphosphonate synthase in the mevalonate pathway.56 This prevents the action of several additional enzymes required for bone resorption. The bisphosphonates include pamidronate, alendronate, ibandronate, risedronate, and zoledronic acid. Zoledronate is much more potent than the other bisphosphonates, in part because it also inhibits tumor cell adhesion to the extracellular matrix.
The initial studies of bisphosphonate usage were primarily in women with metastatic breast cancer. Pamidronate was evaluated in multiple randomized, prospective studies of women with osteolytic bone metastases from breast cancer. The Aredia Breast Cancer Study Group Protocols 18 and 19 enrolled women receiving either chemotherapy (P19) or hormonal therapy (P18). In the P18 study, 372 women were randomized to receive either placebo or pamidronate 90 mg as a 2-hour infusion every 4 weeks for 24 cycles.57 The primary endpoint was the prevention of “skeletal-related complications” (pathologic fractures, spinal cord compression, hypercalcemia, or the requirement for surgery or radiation therapy on bone). There were fewer skeletal-related complications in the pamidronate arm (475 events vs. 648 in the placebo group), but the benefit in reducing pathologic fractures and hypercalcemia was not significant until 18 to 24 months of treatment. The primary difference was that twice as many placebo patients required radiation therapy for palliation of pain, most often in the first 6 to 12 months. There was no difference in survival, although pain levels and serum markers of bone resorption were significantly lower in the group receiving pamidronate.
In the P19 study, women with stage IV breast cancer who were receiving chemotherapy and had lytic bone metastasis were given placebo or pamidronate for 12 monthly cycles.58 Patients in the pamidronate arm suffered fewer overall skeletal complications and had a greater median time to first skeletal complication when compared with patients in the placebo arm of the study. Women treated with pamidronate maintained better performance status and had less bone pain secondary to metastasis.
Rosen et al.59 evaluated 773 patients with bone metastases from solid tumors, including lung cancer, renal cell carcinoma, head and neck cancers, thyroid, and other primary sites (exclusive of breast and prostate cancer). They compared placebo with zoledronic acid in doses of either 4 or 8 mg given every 3 weeks for 9 months. The primary endpoint was proportion of patients developing a skeletal-related event (SRE), including pathologic fracture, spinal cord compression, or the need for surgery or radiation therapy. The proportion of patients developing an SRE was 44% in the placebo group compared with 38% in the 4-mg zoledronic acid group (p = .127) and 35% in the 8-mg group (p = .023). The time to development of an SRE was significantly longer with zoledronic acid compared with placebo (230 vs. 163 days; p = .017). The dose of the 8-mg group was reduced to 4 mg during the latter part of the study because of safety concerns, and thus 25% of patients in the 8-mg treatment arm actually received only 4 mg per dose. In addition, only 25% of patients received the full 9-month course of treatment because of death (27%), adverse events (21%), patient decision to discontinue (15%), or “insufficient efficacy” (7%). There was no improvement in two specific events—surgery to bone and spinal cord compression—in the patients receiving zoledronic acid compared with placebo. In addition, the need for analgesics gradually increased and functional capacity decreased from baseline to month 9 in all groups, with no differences between zoledronic acid and placebo.
The Medical Research Council PRO4 and PRO5 were two prospective, randomized trials conducted to evaluate sodium clodronate in men with prostate cancer. Long-term data showed that sodium clodronate improved overall survival in men with metastatic prostate cancer who were starting hormone therapy, but there was no improvement in overall survival for men with nonmetastatic disease.60
Complications of supportive therapy with bisphosphonates include osteoradionecrosis (particularly of the jaw) and renal insufficiency.61 The mechanism of bisphosphonate-induced osteoradionecrosis is not known. Risk factors include the intravenous use of pamidronate and zoledronic acid, duration of treatment of 36 months or longer, older age in patients with multiple myeloma, and need for periodontal procedures.62
Bisphosphonates are not metabolized by the body and are excreted by the kidneys. Bisphosphonate therapy can lead to renal toxicity. The incidence of renal toxicity is 9% to 15% in trials when 4 mg of zoledronic acid is delivered intravenously during 15 minutes.61 Moreover, the rate of renal toxicity depends on underlying renal disease, dose of bisphosphonate used, and duration of the infusion.
Another form of systemic therapy is the use of agents that target the RANK pathway. Denosumab is a human monoclonal antibody specific for the RANK ligand. The antibody binds to RANKL and thus inhibits the formation, activation, maturation, and survival of osteoclasts.63 In randomized, prospective comparisons of denosumab with zoledronic acid in patients with metastatic breast or prostate cancer, denosumab was superior to zoledronic acid in delaying or preventing the time to skeletal-related events.64,65
RADIATION THERAPY
Radiation therapy has been reported to be effective in palliating painful bone metastases, with partial pain relief seen in 80% to 90% of patients and complete pain relief in 50% of patients (Fig. 94.5). These data are primarily from studies using physician evaluation of pain. When patient evaluation of pain is used, pain improvement is seen in 60% to 80% of patients and complete pain relief is seen in 15% to 40% of patients.66 The response to treatment depends on a large number of factors, including sex, primary site and histology, performance status, type of lesion (osteolytic vs. osteoblastic), location of the metastases, weight-bearing vs. non–weight-bearing site, extent of disease, number of painful sites, marital status, and level of pain prior to treatment. The effectiveness of the treatment also depends on the goal: palliation of pain, prevention of pathologic fracture, avoidance of future treatments, or local control of the disease. The doses required and volumes treated may be quite different for each of these goals. In addition to pain relief, other symptoms may be relieved by radiotherapy. Patients who have improvement in pain after radiotherapy may also have improvement in emotional functioning, decreased insomnia and decreased constipation, and overall improvement in quality-of-life scores.67 Radiation therapy should be an integral part of palliative treatment for bone metastases for treatment of pain and prevention of other symptoms.68
FIGURE 94.5. Axial computed tomography scans from a patient with metastatic breast cancer. Images through the frontal skull (A) and thoracic spine (B) show large, destructive, osseous lesions. Images 1 year later (C, D), after palliative external-beam radiation, show significant healing of the bone.
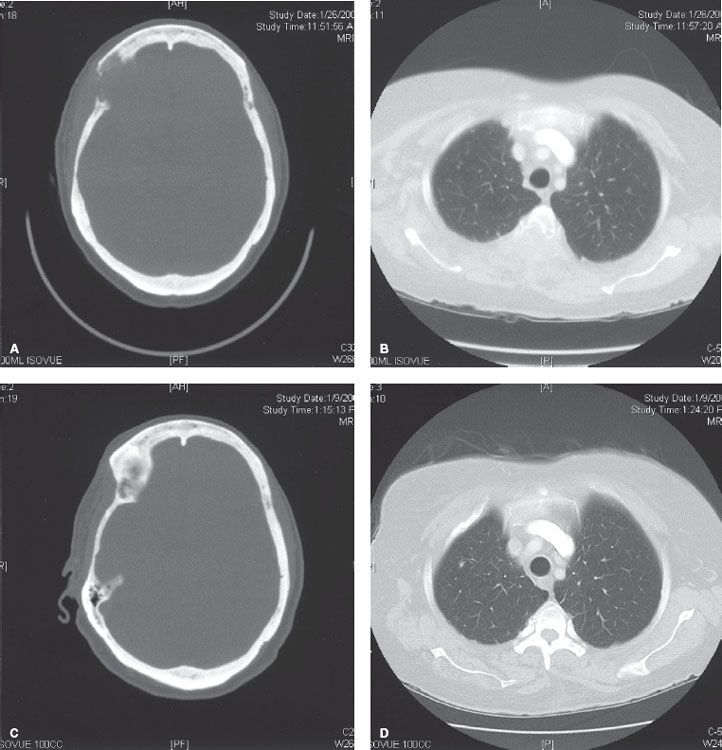
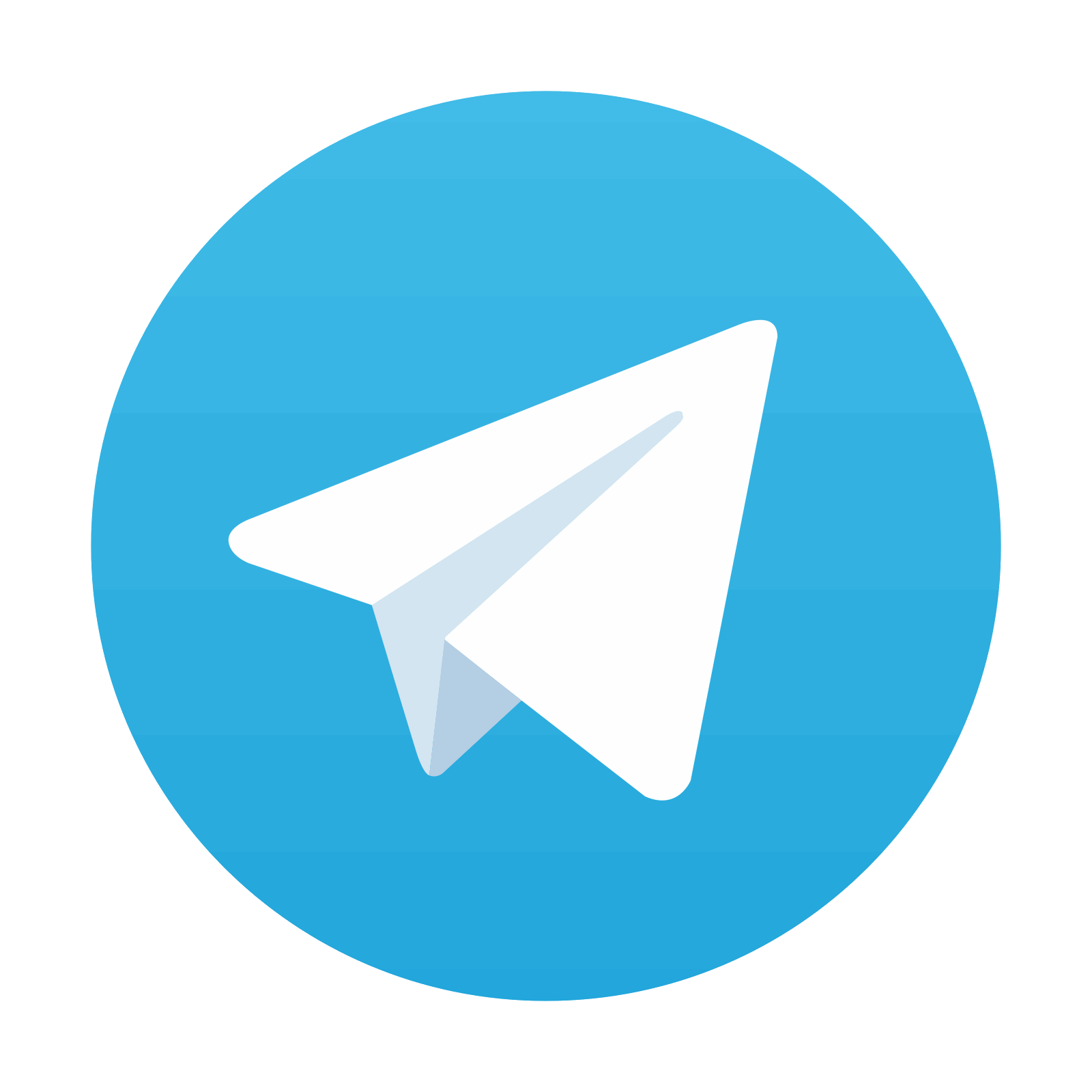