Table 6.1 Selected cytokines of innate and adaptive immunity | |||||||||||||||||||||||||||||||||||||||||||||||||||||||||||||||||||||
---|---|---|---|---|---|---|---|---|---|---|---|---|---|---|---|---|---|---|---|---|---|---|---|---|---|---|---|---|---|---|---|---|---|---|---|---|---|---|---|---|---|---|---|---|---|---|---|---|---|---|---|---|---|---|---|---|---|---|---|---|---|---|---|---|---|---|---|---|---|
|
are present in most healthy cells. A lack of class I MHC, often termed “missing self,” will result in NK cell activation due to a lack of inhibitory signals.
Table 6.2 Selected chemokines | ||||||||||||||||||||||||||||||||||||||||||||||||||||||||||||||||||||||||||||||||||||||||||||||||
---|---|---|---|---|---|---|---|---|---|---|---|---|---|---|---|---|---|---|---|---|---|---|---|---|---|---|---|---|---|---|---|---|---|---|---|---|---|---|---|---|---|---|---|---|---|---|---|---|---|---|---|---|---|---|---|---|---|---|---|---|---|---|---|---|---|---|---|---|---|---|---|---|---|---|---|---|---|---|---|---|---|---|---|---|---|---|---|---|---|---|---|---|---|---|---|---|
|
adapt to different types of microbial infections, and memory. The majority of lymphocytes are located within lymphoid tissues and epithelial barrier tissue such as skin and gut. There is constant trafficking of between these tissues and the circulation, where they number 1-3 × 103/mm3. In blood smears, resting lymphocytes appear as relatively homogeneous round cells with a high nuclear:cytoplasmic ratio and a diameter of approximately 7 µM. This uniform morphologic appearance does not reflect the existence of multiple lymphocyte subsets.
Table 6.3 Types of lymphocytes | ||||||||||||||||||||||||||||||||||||||||||||||||||||||||||||||||||||||
---|---|---|---|---|---|---|---|---|---|---|---|---|---|---|---|---|---|---|---|---|---|---|---|---|---|---|---|---|---|---|---|---|---|---|---|---|---|---|---|---|---|---|---|---|---|---|---|---|---|---|---|---|---|---|---|---|---|---|---|---|---|---|---|---|---|---|---|---|---|---|
|
lymphocytes, which are relatively short-lived cells that actively contribute to elimination of antigen. After most microbial antigen-specific B- and T-cell responses wane, a population of longlived progeny of the expanded lymphocyte clones persists as memory cells, which mediate rapid and specialized responses to subsequent exposures to the microbial antigens. Memory lymphocytes can survive for many years, and probably undergo a low level of antigen-independent proliferation to maintain their numbers.20,21,22 In vitro assays for recall responses to antigens after infection or immunization indicate that most memory T cells express the CD45RO surface protein, while naïve T cells, such as found in neonates, are mostly CD45RA+. Memory T cells also express the IL-7 receptor. Effector T cells are usually localized within lymphoid organs or in infected tissues, and only rarely are they numerous in the blood, such as during acute EBV infections when they appear as lymphoblasts. Specific details of T-and B-cell antigen recognition, subsets, and effector function will be discussed later in this chapter.
antigens, which occurs in the white pulp.36 T cells in the white pulp are located in sheaths around small branches of the splenic artery, and B-cell follicles are arranged adjacent to the T-cell zones. FRC conduits like those in lymph nodes traverse the follicles. The anatomic arrangement of B cells and T cells in the splenic white pulp optimizes antibody responses.
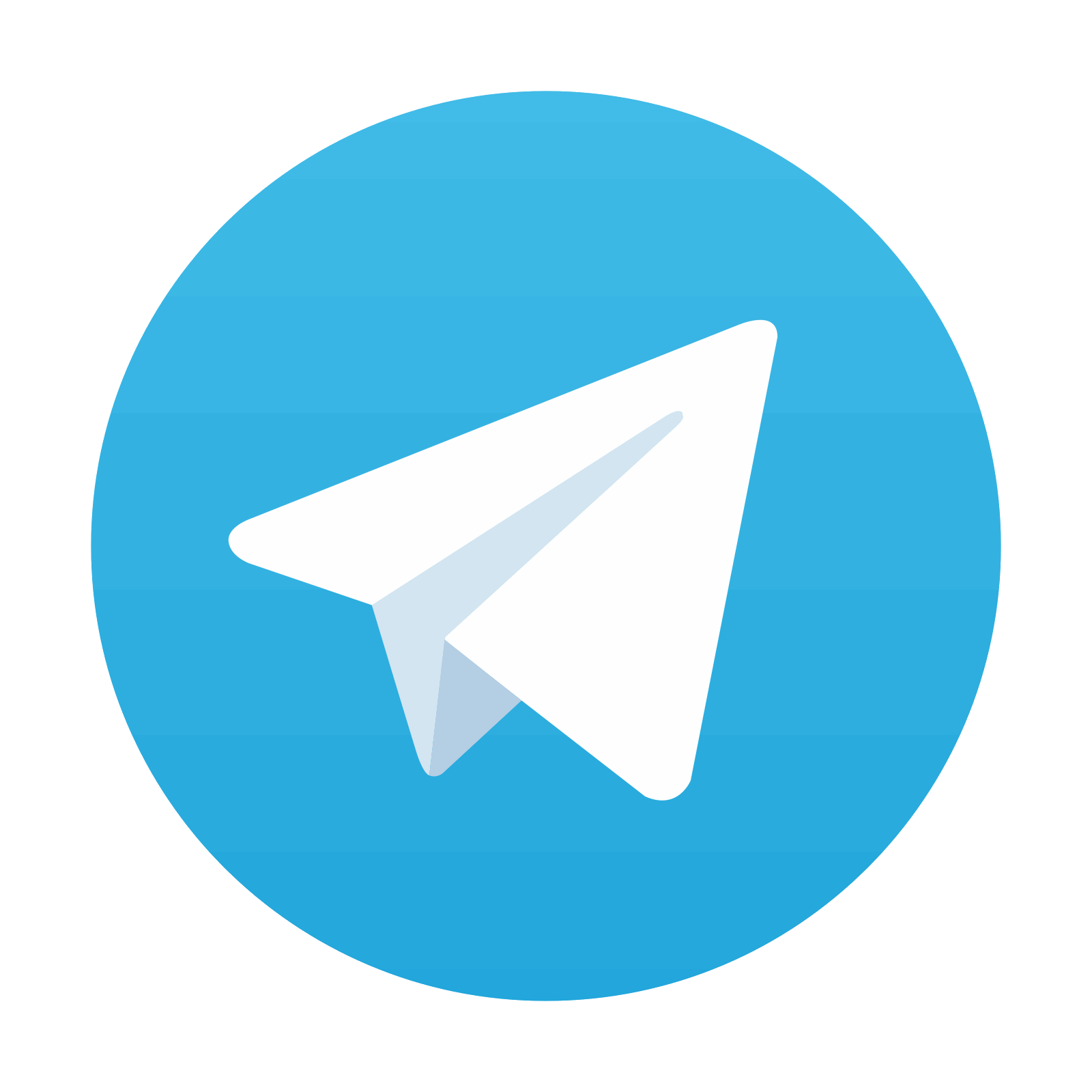
Stay updated, free articles. Join our Telegram channel

Full access? Get Clinical Tree
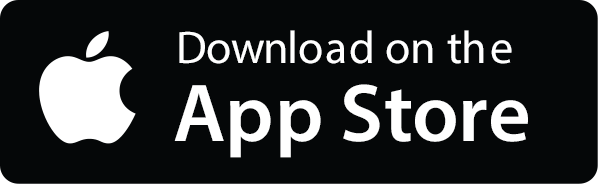
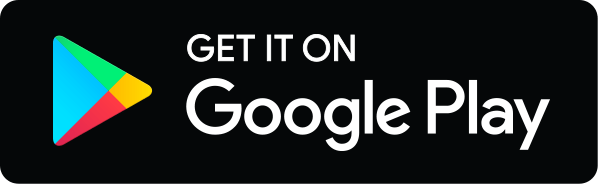