Overview of Basic Coagulation and Fibrinolysis
Gilbert C.White II
Victor J. Marder
Sam Schulman
William C. Aird
Joel S. Bennett
The development of a fibrin clot occurs after a series of enzymatic reactions that lead to the generation of thrombin. These reactions were originally described as an enzyme cascade1 or waterfall,2 a model that remains conceptually useful today (see FIGURE 8.1A). Notions of this process have evolved over the past 45 years as proteins have been purified, their function characterized, and additional factors identified. For example, factors VIII and V, which were originally depicted as enzymes in the waterfall and cascade hypotheses, were identified as nonenzymatic proteins that function as catalysts to increase the rate of activation of factor X and prothrombin, respectively. More importantly for the clinician, the idea evolved that clotting was initiated by either contact activation via a so-called intrinsic pathway (termed because all of the components were contained in blood) or activation by tissue factor via an extrinsic pathway (termed because the initiator, tissue factor, was considered extrinsic to blood). This separation into two pathways, measured by different in vitro clotting assays, was useful for the diagnosis of bleeding disorders and helped tie the biology closer with diagnostic testing (FIGURE 8.1 B).
Over the past decade or two, concepts regarding the initiation of coagulation have continued to develop, leading to further evolution of the models for coagulation. Tissue factor and factor VIIa are now considered to be the major initiators of coagulation in vivo,3,4,5 not the contact activation system, which is thought to be a key link between coagulation and inflammation. Accordingly, humoral blood coagulation is believed to initiate in vivo through a cell-based system (FIGURE 8.1C) that occurs when tissue factor, a membrane-associated lipoprotein that is present in most cells, is released upon cell damage or is secreted by cells such as monocytes and platelets.6,7,8 Tissue factor forms a complex with and activates factor VII, which in turns activates factor X leading to the generation of small amounts of thrombin. This is called the “initiation phase” of coagulation. The next phase is an “amplification phase” in which the thrombin that is generated is then able to activate factor IX which activates more factor X, leading to more thrombin. The various proteins involved in coagulation, their genes, their function, and their enzymology are described in the chapters that comprise this section of the text. In this introduction, we highlight some of the features of the coagulation process. The reader is also referred to Chapters 3, 4, and 5 in the initial section of the text for background reading.
I EVOLUTION OF COAGULATION PROTEINS
Just as this text is written at a point in time, the coagulation system as we describe it in this book is at a point in time. The human coagulation system has evolved over hundreds of millions of years to become the complex system it is today.9 In lower-order organisms, thrombosis was not an issue—hemostasis was key; breeches in the vascular system had to be plugged as quickly as possible or exsanguination occurred. As reviewed in Chapter 2 of this text, the first coagulation systems were very simple, consisting of a fibrinogen, a thrombin molecule, and an activator. As organisms grew in complexity, the coagulation system evolved through domain duplication and insertion and through protein duplication. Factor VIII is a good example, a protein that has evolved over the past 450 million years to become what it is today (see FIGURE 8.2). Factor VIII is composed of three A domain repeats, a B domain, and two C domain repeats. The A domains are involved in protein-protein interactions, the B domain is removed during activation and may play a role in protein synthesis, and the C domains are involved in phospholipid binding. The A domains have their origin in blue-green algae. Over millennia, the A domain in algae duplicated and then triplicated, each A domain repeat evolving independently, to eventually form a ceruloplasmin precursor protein containing three A domain repeats, each with about 30% identity to each other. At the same time, a primordial C domain that was present in dictyostelium duplicated, and each C domain evolved independently. Somewhere in evolution, the ceruloplasmin precursor protein with its triplicated A domains and the duplicated C domains came together to form an A3-C2 precursor protein. Different evolutionarily unrelated B domains were inserted into the A3-C2 protein in the same position, between the A2 and the A3 domains, one B domain for factor VIII, and one B domain for factor V. The FVIII protein evolved to interact very specifically with factors IX and X in the factor X activation or “tenase” complex. The FV protein evolved to interact very specifically with factors X and II in the prothrombin-activating or “prothrombinase” complex. Presumably, these coagulation factors continue to evolve. The structure and even the function of factors VIII and V hundreds of thousands of years in the future is unknown, but evolutionary pressure will drive the process!
In a similar manner, factors VII, IX, X, and protein C, the vitamin K-dependent coagulation factors, characterized now by their serine-histidine-aspartic acid charge relay catalytic domain, the duplicated epidermal growth factor domains which are involved in protein-protein interactions, and the membrane-binding, amino-terminal ®-carboxyglutamic acidcontaining domain, evolved through domain duplication and structural evolution. Prothrombin differs from the other vitamin K-dependent proteins by having two kringle domains in place of the EGF (epidermal growth factor) domains; protein Z differs by lacking the histidine and serine residues necessary
for catalysis in the catalytic domain. Because some of these genes are on separate chromosomes, there is speculation9 that the duplication of the vitamin K-dependent factors came about through two rounds of total genome duplication that occurred in early vertebrate evolution some 500 million years ago.10 Each vitamin K-dependent protein now plays a unique role in the coagulation process. Their evolution as separate clotting factors with separate functions adds to the complexity of the system, its control, and its specificity.
for catalysis in the catalytic domain. Because some of these genes are on separate chromosomes, there is speculation9 that the duplication of the vitamin K-dependent factors came about through two rounds of total genome duplication that occurred in early vertebrate evolution some 500 million years ago.10 Each vitamin K-dependent protein now plays a unique role in the coagulation process. Their evolution as separate clotting factors with separate functions adds to the complexity of the system, its control, and its specificity.
![]() FIGURE 8.1 The human coagulation cascade through the years. A: The original coagulation cascade or waterfall postulated by MacFarlane1 and by Ratnoff and Davie.2 The development of a fibrin clot is seen as a series of sequential enzymatic reactions, starting with factor XII and ending with the conversion of fibrinogen to fibrin. B: Modified coagulation cascade used in the 1970s through the 1990s. This modified cascade began to separate the coagulation process into an intrinsic and extrinsic system, each assessed separately by a PT and a PTT. It also reflected the understanding that factors VIII and V were enzymatic cofactors not proteolytically active enzymes. C: Current concepts of coagulation, which indicate the cell-based nature of coagulation. |
I COAGULATION TESTING
Early on, clotting assays that were sensitive to components of the coagulation system were developed and were used to define the enzymatic pathways leading to clot formation. The two most important of these, the prothrombin time (PT) developed by Quick11 and the partial thromboplastin time (PTT) developed by Langdell et al.12 and later modified by Rapaport,13 continue to be used by coagulation laboratories throughout the world, The PT measures the factors in the extrinsic pathway (FIGURE 8.1 B) and is sensitive to plasma levels of factors VII, X, V, prothrombin, and fibrinogen. The PTT, which measures the intrinsic pathway (FIGURE 8.1B), is sensitive to plasma levels of the contact factors, factors IX, VIII, X, V, prothrombin, and fibrinogen. The factors common to both pathways, factors X, V, prothrombin, and fibrinogen, constitute what is called the final common pathway. It is important to emphasize that this arbitrary division into extrinsic, intrinsic, and final common pathways does not represent what occurs in vivo and is used simply to understand and interpret the clotting assays that are used to measure coagulation (see Chapter 50). These divisions into arbitrary pathways also do not reflect important connections that exist between the various pathways. An example is the ability of tissue factor-factor VIIa complex to activate both factor
IX in the intrinsic pathway as well as factor X in the common pathway. Nevertheless, the tests are useful in screening for various deficiencies in the coagulation system and as a basis for assays for the functional measurement of clotting factor activities. Tests that measure fibrinolytic activity are also available. The euglobulin clot lysis time is sensitive to proteins leading to and regulating clot lysis.
IX in the intrinsic pathway as well as factor X in the common pathway. Nevertheless, the tests are useful in screening for various deficiencies in the coagulation system and as a basis for assays for the functional measurement of clotting factor activities. Tests that measure fibrinolytic activity are also available. The euglobulin clot lysis time is sensitive to proteins leading to and regulating clot lysis.
I THE ROLE OF SURFACES IN COAGULATION
Functionally, the relationship between clotting and surfaces is striking.14,15,16,17 For a process that is typically termed humoral, most of the coagulation reactions take place on biologic surfaces (see FIGURE 8.3). For example, the assembly of the prothrombinase complex, where factors Xa and thrombin-activated factor V form a complex with prothrombin, occurs on acidic phospholipids on the surface of cells. The presence of a phospholipid surface increases the rate of activation of prothrombin by several orders of magnitude (see Chapter 4). It also localizes the reaction to the site of injury and may protect the reaction from inhibitors. Likewise, the assembly of the tenase complex, where factors IXa and thrombin-activated factor VIII form a complex with factor X, occurs on acidic phospholipids. These reactions on acidic phospholipids are mediated in part by ρ-carboxyglutamic acid (Gla) residues present in the amino-terminal Gla domain of the vitamin K-dependent coagulation factors.18,19 The ®-carboxylation of glutamic acid occurs posttranslationally and confers an extra negative charge on glutamic acid that is thought to mediate calcium binding, which in turn induces a change in conformation that enables the protein to interact with acidic phospholipids on cellular surfaces.20,21 FIGURE 8.4 shows the insertion of a Gla domain into a phospholipid surface.21 The activation of protein C by thrombin is another reaction that occurs on a cellular surface, in this case the surface of the endothelial cell, by virtue of thrombomodulin, an endothelial-specific integral membrane protein that functions as a cofactor in the reaction, along with protein S.22,23,24 Both protein C and protein S are vitamin K-dependent and thus interact with the membrane in the same way as factors II, VII, IX, and X. Activated protein C (APC) can thereafter interact with the endothelial protein C receptor (EPCR) to mediate the cellular effects of APC, another reaction that occurs on the cell surface by virtue of the membrane integration of EPCR.25,26 The contact activation complex in which prekallikrein and high molecular weight kininogen form a complex with factor XII is also assembled on a surface, in this case, negatively charged surfaces like glass and kaolin, materials used to initiate coagulation in vitro via the intrinsic pathway.27,28,29,30 In vivo, these negatively charged surfaces might be bacteria or other inflammatory generated surfaces. Thus, the assembly of cofactor, enzyme, and substrate on surfaces is a recurring theme in blood coagulation, resulting in optimal efficiency and rate of the molecular reactions, especially as a phospholipid or cell membrane provides the surface for efficient positioning of interacting enzyme complexes with proenzyme substrates.
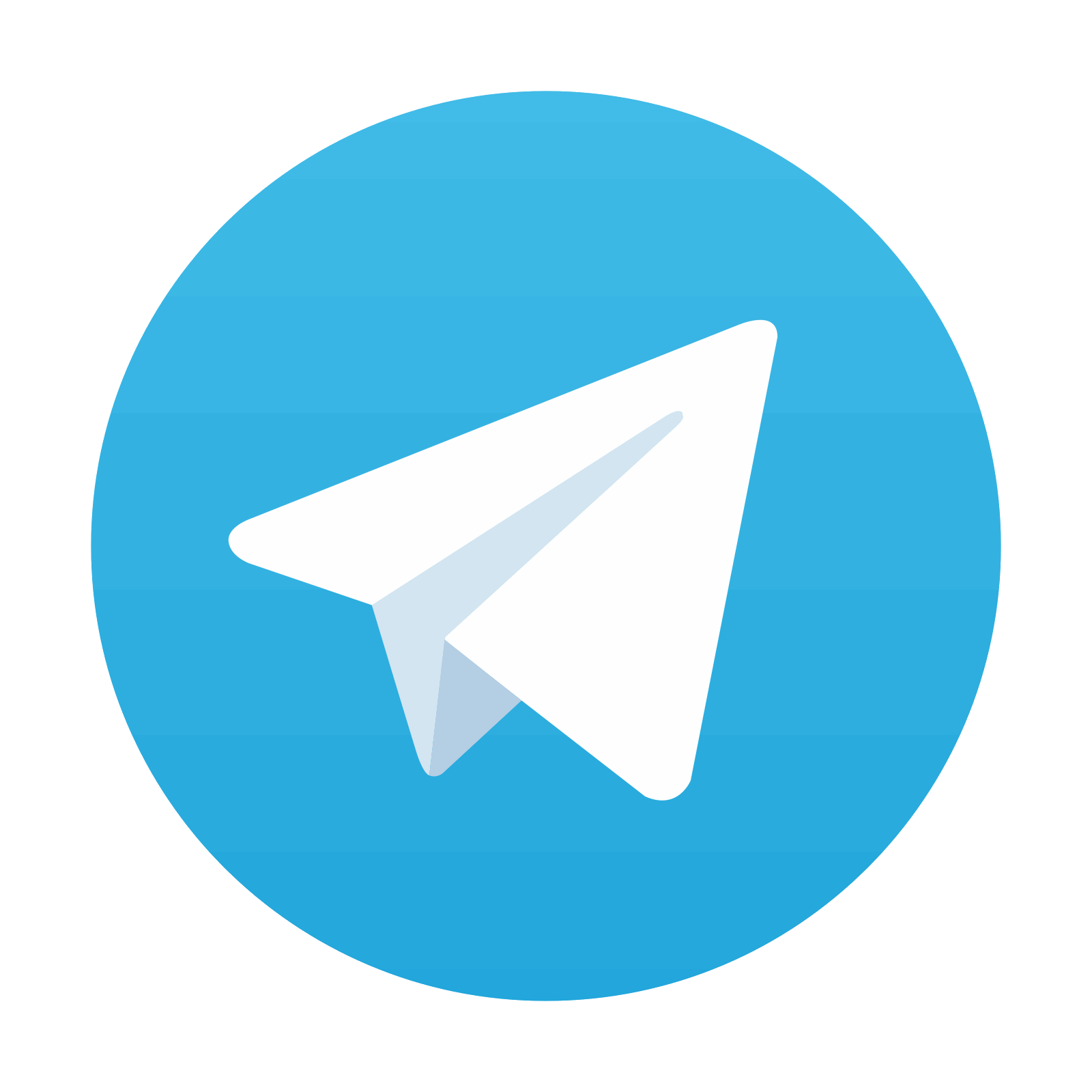
Stay updated, free articles. Join our Telegram channel

Full access? Get Clinical Tree
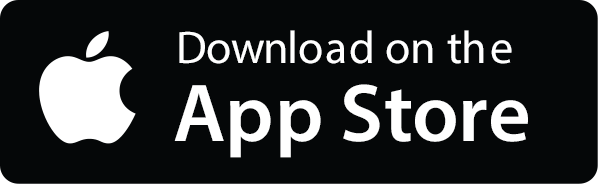
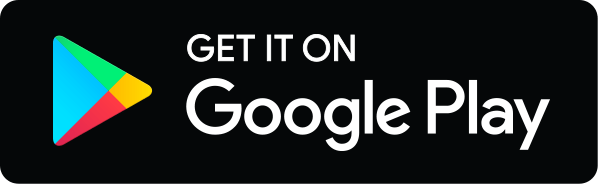