Other Nontraditional Risk Factors for Cardiovascular Disease
Simeon Margolis
It is relatively easy to decide how aggressively to pursue preventive measures in people at low risk (no risk factors) and in those with multiple risk factors or known cardiovascular disease (CVD). Decisions are more difficult in the large number of individuals considered at “intermediate risk” because they have only one traditional risk factor.
Despite the lack of clear evidence for its support, a frequently heard statement is that the traditional risk factors account for only 50% of those who go on to develop coronary heart disease. However, a publication in 2003 cast considerable doubt on this assertion. Greenland et al. found at least one major risk factor (hypercholesterolemia, hypertension, cigarette smoking, and diabetes) in 87% to 100% of those who suffered a fatal or nonfatal myocardial infarction (MI) (1). Although such findings reemphasize the importance of these conventional risk factors, their high frequency in the general population makes it difficult to predict the likelihood that an individual with one or more of these risk factors will develop clinically significant coronary disease.
One approach to this question is the Framingham Risk Score (FRS), which incorporates age, total cholesterol (TC), high-density lipoprotein cholesterol (HDL-C), systolic blood pressure, treatment for hypertension, and cigarette smoking to estimate the 10-year risk of MI and coronary death. Risk status is defined as follows: high = >20% 10-year risk; intermediate = 10% to 20% 10-year risk; and low = <10% 10-year risk.
Weaknesses of the FRS include the failure to include family history, excessive dependence on age, and inappropriately low risk attributed to women and younger people with hypercholesterolemia. Moreover, relatively few physicians use the FRS to make treatment decisions.
Much effort has been directed toward identifying other “nontraditional” factors that will help to predict which individuals have a greater likelihood of developing CVD. The latest National Cholesterol Education Program (NCEP) Adult Treatment Panel III (ATP III) guidelines listed a number of “emerging” risk factors (Table 17.1) that might help to make treatment decisions in people who are at “intermediate risk” and even guide management of those with several risk factors. Ridker et al. formulated the Reynolds Risk Score (RRS) by adding to the FRS one of these emerging risk factors, C-reactive protein (CRP), and a history of premature coronary events in a parent (2). Although the RRS does a better job than the FRS in predicting coronary events among women at intermediate risk, both risk scores have serious weaknesses. They do not include deaths or strokes resulting from cerebrovascular disease. An even greater problem is that both scores only predict what will happen in the next 10 years rather than throughout the lifetime of a man or woman. A 50-year-old man or woman, for example, is likely to live far more than 10 years. A more apt choice would be lifetime risk, the risk of dying of CVD versus the risk of dying of other diseases throughout their remaining lifespan. A 2003 report from Framingham showed that the cholesterol level at ages 40, 50, 60, 70, and 80 predicted the lifetime risk of CVD for both men and women (3).
The c-statistic, or area under the receiver operating characteristic (ROC) curve (AUC), is one of the methods often used to assess the additional prognostic value of novel risk factors. However, it may be ill-advised to rely solely on the c-statistic to evaluate how useful they are as risk predictors because “a biomarker with an odds ratio of 3 may have little effect on the c-statistic” (4).
This chapter will not consider some of the emerging risk factors suggested by the NCEP: insulin resistance (Chapter 10), CRP (Chapter 15), Lp(a) (Chapter 18), or coronary calcium score (Chapter 6), which are covered elsewhere in this textbook. After a brief discussion of the prothrombotic factors mentioned in the NCEP guidelines (Table 17.2), the chapter will
cover other factors that appear to have a causal relationship with atherosclerosis, or are associated with a greater likelihood of CVD. The final section will consider several disorders that are associated with an increased risk of CVD (Table 17.3).
cover other factors that appear to have a causal relationship with atherosclerosis, or are associated with a greater likelihood of CVD. The final section will consider several disorders that are associated with an increased risk of CVD (Table 17.3).
TABLE 17.1 EMERGING RISK FACTORS | ||||||||||||||||||
---|---|---|---|---|---|---|---|---|---|---|---|---|---|---|---|---|---|---|
|
TABLE 17.2 PROTHROMBOTIC FACTORS | ||||||||
---|---|---|---|---|---|---|---|---|
|
TABLE 17.3 DISORDERS ASSOCIATED WITH INCREASED CORONARY HEART DISEASE | |||||||||||||||||||||||||||||||||
---|---|---|---|---|---|---|---|---|---|---|---|---|---|---|---|---|---|---|---|---|---|---|---|---|---|---|---|---|---|---|---|---|---|
|
THEORETICAL CONSIDERATIONS
Prothrombotic Factors
Although this textbook deals primarily with the entry and removal of lipids from atherosclerotic plaques, clot formation and lysis are critical for the arterial occlusions that trigger MIs and strokes. Coagulation factors, von Willebrand factor (vWF), and platelet aggregation are required for the formation of blood clots. Clot lysis depends on a balance between tissue-plasminogen activator (t-PA) and plasminogen activator inhibitor-1 (PAI-1).
A number of studies have reported a relationship between CVD and abnormal levels of one or more of these factors. In the Northwick Park Heart Study, high levels of factor VII activity and plasma fibrinogen were associated with a higher risk of coronary events (5). A later European study found that elevated blood levels of fibrinogen and antigens for vWF and t-PA were independently associated with an increased incidence of MI and sudden death in patients with angina (5). The Atherosclerosis Risk in Communities (ARIC) study also found that the incidence of new CVD was greater in those who had higher baseline levels of fibrinogen, even after adjustment for other risk factors (5). In a 1998 meta-analysis, elevated fibrinogen levels were associated with a higher risk of CVD (5), as well as risk of peripheral arterial disease. Elevated fibrinogen levels may be related to cigarette smoking.
Many studies have shown that abnormalities in the formation and lysis of blood clots play a particularly prominent role in the development of cardiovascular complications in people with insulin resistance and diabetes. High levels of PAI-1 suppress fibrinolysis in normoglycemic subjects with insulin resistance and in patients with type 2 diabetes (6); and high PAI-1 levels in plasma preceded a first acute MI in both men and women (7) and were a risk factor for recurrent MI (8). Among subjects with normal glucose tolerance in the Framingham Offspring Study, hyperinsulinemia was associated with higher levels of fibrinogen (in women but not men) and antigen levels for PAI-1, t-PA, vWF, and factor VII (6). However, levels of PAI-1 and t-PA antigens, but not the other factors, were higher in subjects with glucose intolerance compared with those whohad normal glucose tolerance. In type 2 diabetes, higher levels of PAI-1 are possibly related to its greater release from adipose tissue in obese individuals. A 2007 review on diabetes as a prothrombotic condition concluded “The combination of increased circulating coagulation zymogens, inhibition of fibrinolysis, changes in fibrin structure/function and alterations in platelet reactivity creates a thrombotic risk clustering which underpins the development of cardiovascular disease” (6).
Among apparently healthy men in the Physicians’ Health Study, elevated plasma levels of D-dimer, the primary degradation product of cross-linked fibrin, were associated with increased risks of future MI, but did not appear to be an independent predictor after adjustment for other risk factors (9). A 1999 report found that plasma levels of D-dimer, but not PAI-1, were associated with a significantly increased risk of MI and coronary death in the Cardiovascular Health Study cohort of 5,201 men and women who were 65 years of age or older (9). In another study of 5,661 men who were monitored for 16 years in the British Regional Heart Study, accompanied by a meta-analysis, those with the highest plasma levels of D-dimer had a significantly increased risk of CVD (9).
Blood levels of lipids and lipoproteins may influence fibrinolysis. In a Japanese study, blood levels of PAI-1 and factors VII and X were positively associated with fasting triglycerides in healthy control subjects as well as in those with CVD (10). Both clinical and laboratory findings indicate that hypertriglyceridemia associated with insulin resistance increases the synthesis and secretion of PAI-1 (11). In one study very low density lipoprotein (VLDL) induced the transcription of the promoter for human PAI-1 in endothelial cells (12). And some, but not all, studies have shown a rise in PAI-1 levels during postprandial hypertriglyceridemia (13). An increase in levels of factor VII and PAI-1 in the postprandial state may potentially promote clot formation and hinder fibrinolysis (13).
Lp(a) particles that contain small apo(a) isoforms with the highest affinity for fibrin can compete with the binding of plasminogen and slow or prevent its cleavage by t-PA to plasmin (14) (see also Chapter 18). Thus, high plasma concentrations of some types of Lp(a) may exert antifibrinolytic activity.
Despite these associations of hemostatic and fibrinolytic factors with the risk for CVD, their measurement does not add significantly to that of more established risk factors in the prediction of coronary events.
Biochemical Factors
Homocysteine
Homocysteine is an intermediate formed during the transfer of methyl groups from methionine (Fig. 17.1). Methionine and ATP are combined by the enzyme methionine adenosyltransferase to form S-adenosylmethionine, which transfers methyl groups to a number of intermediates. The other product of these methyl-transfer reactions is S-adenosylhomocysteine, which is cleaved by a hydrolytic enzyme to form homocysteine and water. Homocysteine can be converted back to methionine by the addition of a methyl group in a reaction that requires vitamin B12 and folic acid (Fig. 17.1). Alternatively, homocysteine
can be combined with serine by cystathionine β-synthase (CBS), an enzyme that contains pyridoxine (vitamin B6), to form cystathionine. Cystathionine is cleaved to produce α-ketobutyrate and cysteine. Thus, the breakdown of homocysteine requires three vitamins—folic acid, B12, and pyridoxine.
can be combined with serine by cystathionine β-synthase (CBS), an enzyme that contains pyridoxine (vitamin B6), to form cystathionine. Cystathionine is cleaved to produce α-ketobutyrate and cysteine. Thus, the breakdown of homocysteine requires three vitamins—folic acid, B12, and pyridoxine.
![]() FIGURE 17.1 Homocysteine metabolism in man. The large arrows indicate enzyme reactions that are regulated by S-adenosyl-L-methionine (SAM); closed arrow indicates inhibition; open arrow indicates activation. Enzymes: N-5-methyltetrahydrofolate, homocysteine methyltransferase, methylenetetrahydrofolate reductase, homocysteine methyltransferase, choline dehydrogenase, cystathionine-β-synthase, ρ-cystathionase. THF, tetrahydrofolate; PLP, pyridoxal-5′-phosphate. (Reproduced from Selhub (19) with permission.) |
Homocystinuria, a disorder characterized by extremely high levels of this amino acid in the blood and urine, results from an inherited defect in CBS, one of the enzymes involved in the metabolism of homocysteine (Fig. 17.1). Affected individuals have marked thromboses in veins and arteries, as well as advanced atherosclerosis, and often die at an early age of a heart attack or stroke. Suggested mechanisms for the atherothrombotic effects of homocysteine include endothelial cell damage, and inflammatory and prothrombotic effects.
Small amounts of homocysteine are normally found in the blood. In 1969 Kilmer C. McCully published a paper suggesting that more subtle elevations in homocysteine levels might accelerate the development of atherosclerosis. Interest in homocysteine then waned for about 20 years. Many epidemiologic studies have subsequently shown that even modest elevations in blood levels of homocysteine are associated with atherosclerotic complications. In 1995 Motulsky et al. reviewed a spate of studies, beginning in the mid 1980s, which clearly showed that small elevations in homocysteine levels are associated with an increased risk of heart attacks, stroke, and peripheral vascular disease (15). They also suggested the probable benefit of increasing folic acid intake. Blood levels of homocysteine also strongly predicted the risk of mortality in patients with known CVD (16). In several studies, for example, the Women’s Health Study, which compared various markers, CRP was a more effective predictor of subsequent vascular events than homocysteine.
Elevated levels of homocysteine, present in more than 80% of patients with end-stage renal disease, are an independent risk factor for atherosclerosis in such patients (17). Homocysteine levels also tend to be elevated in smokers and in patients with hypothyroidism, pernicious anemia, and psoriasis. Fenofibrate, bezofibrate, and hydrochlorothiazide raise blood levels of homocysteine (18). Homocysteine levels are normal or low in diabetic patients who do not have renal disease (16).
A nutritional study in 1993 showed an association between elevated levels of homocysteine and low blood levels and dietary intakes of folate and pyridoxine (19), and an earlier study had reported that dietary supplements of folate lower blood levels of homocysteine (19). These findings led many
physicians to measure homocysteine levels and to recommend that their patients take supplements of folic acid, and often pyridoxine and B12. A meta-analysis of results from four trials involving 14,000 participants, however, showed no beneficial effects of B-vitamin supplements on CVD (20). By contrast, folate supplements reduced vascular events in homozygotes with homocystinuria.
physicians to measure homocysteine levels and to recommend that their patients take supplements of folic acid, and often pyridoxine and B12. A meta-analysis of results from four trials involving 14,000 participants, however, showed no beneficial effects of B-vitamin supplements on CVD (20). By contrast, folate supplements reduced vascular events in homozygotes with homocystinuria.
Even though modest reductions in homocysteine do not appear to decrease CVD events, should we screen patients for homocysteine levels? The following recommendations from three different groups were accessed online in May 2006 (21). The International Task Force for Prevention of Coronary Heart Disease stated that there is insufficient evidence to recommend measuring homocysteine levels in the general population. They point out that homocysteine may not be an independent risk factor (it correlates, e.g., with renal function and smoking) and the recommended cutoff values for elevated homocysteine vary from 12 to 18 µmol/L. The American Heart Association and the Centers for Disease Control and Prevention (CDC) jointly said that screening for homocysteine levels may be useful in patients with a personal or family history of CVD but who lack the well-established risk factors. According to the National Guideline Clearinghouse, there is insufficient evidence to recommend for or against screening homocysteine levels in the general population or even in high-risk populations, but screening the latter group may identify individuals who might benefit from aggressive risk factor modification.
![]() FIGURE 17.2 Postulated role of lipoprotein-associated phospholipase A2 (Lp-PLA2) in atherogenesis. Most Lp-PLA2 in blood is transported on low-density lipoprotein (LDL) and enters the vascular wall with it (upper left). Lp-PLA2 then hydrolyzes oxidized LDL (lower left and center) to produce two inflammatory products, lysophosphatidylcholine (LysoPC) and oxidized fatty acids. LysoPC enhances atherogenesis in a number of ways: it attracts monocytes to enter the arterial wall; impairs the function of endothelial cells; and causes cell death by disrupting plasma membranes and by producing apoptosis in smooth muscle cells and macrophages (center and right). Macrophages secrete large amounts of the enzyme, and its release from macrophages is thought to be a direct response to inflammation. (Reproduced from Zalewski A, Macphee C (22) with permission.) (see color insert.) |
I can see no reason to screen for homocysteine in either high- or low-risk patients, but homocysteine levels may help guide treatment in those at intermediate risk since homocysteine remains a risk factor for CVD, even if lowering it has not been shown to be beneficial.
Lipoprotein-associated Phospholipase A2 (Lp-PLA2)
Pathophysiology of Lp-PLA2. Also referred to as platelet activating factor acetylhydrolase (PAF-AH), Lp-PLA2 is a member of the phospholipase A2 superfamily, enzymes that remove fatty acids from the 2-position of phospholipids. Lp-PLA2 is a polypeptide of 441 amino acids with an estimated molecular weight of about 50,000 based on its DNA structure. A role for Lp-PLA2 in atherogenesis is based on its hydrolysis of oxidized lipids in low-density lipoprotein (LDL) to produce two inflammatory products, lysophosphatidylcholine (LysoPC) and oxidized fatty acids (Fig. 17.2) (22). LysoPC enhances
atherogenesis in a number of ways: it attracts monocytes to enter the arterial wall; impairs the function of endothelial cells; and causes cell death by disrupting plasma membranes and by producing apoptosis in smooth muscle cells and macrophages (Fig. 17.2) (22,23). Moreover, staining for Lp-PLA2 was absent or minimal in early plaques but strongly expressed in the necrotic core and surrounding macrophages of vulnerable and ruptured plaques in coronary arteries (24).
atherogenesis in a number of ways: it attracts monocytes to enter the arterial wall; impairs the function of endothelial cells; and causes cell death by disrupting plasma membranes and by producing apoptosis in smooth muscle cells and macrophages (Fig. 17.2) (22,23). Moreover, staining for Lp-PLA2 was absent or minimal in early plaques but strongly expressed in the necrotic core and surrounding macrophages of vulnerable and ruptured plaques in coronary arteries (24).
Although the enzyme is present within cells of most tissues, as well as in plasma, of particular interest is the Lp-PLA2 in macrophages (Fig. 17.2). Macrophages secrete large amounts of the enzyme, and its release from macrophages is thought to be a direct response to inflammation (22,23). In plasma 83% of Lp-PLA2 is bound to LDL, 11% to HDL, and 6% to VLDL.
Lp-PLA2 as a Risk Factor for CVD. Several large studies have identified the macrophage-derived enzyme, Lp-PLA2, as an independent predictor of coronary events (25). Data from participants in the West of Scotland Coronary Prevention Study (WOSCOPS), the Monitoring Trends and Determinants in Cardiovascular Diseases (MONICA) study in south Germany, and the Rotterdam Study all demonstrated a significant association between Lp-PLA2 and CVD.
WOSCOPS evaluated the effect of pravastatin in 6,595 hypercholesterolemic men who had no prior coronary event (25). The risk of a coronary event was almost twice as high in men in the highest quintile of blood Lp-PLA2 when compared to those in the lowest quintile. While men in the highest quintile for CRP levels also had a twofold greater risk of subsequent events, this relationship was greatly attenuated in other quintiles when other risk factors were included in multivariate analyses. By contrast, the continued highly significant relationship between Lp-PLA2 and the risk of CVD for all quintiles in multivariate analysis demonstrated that Lp-PLA2 was an independent marker of CVD. In this study, the levels of CRP did not correlate with those of Lp-PLA2.
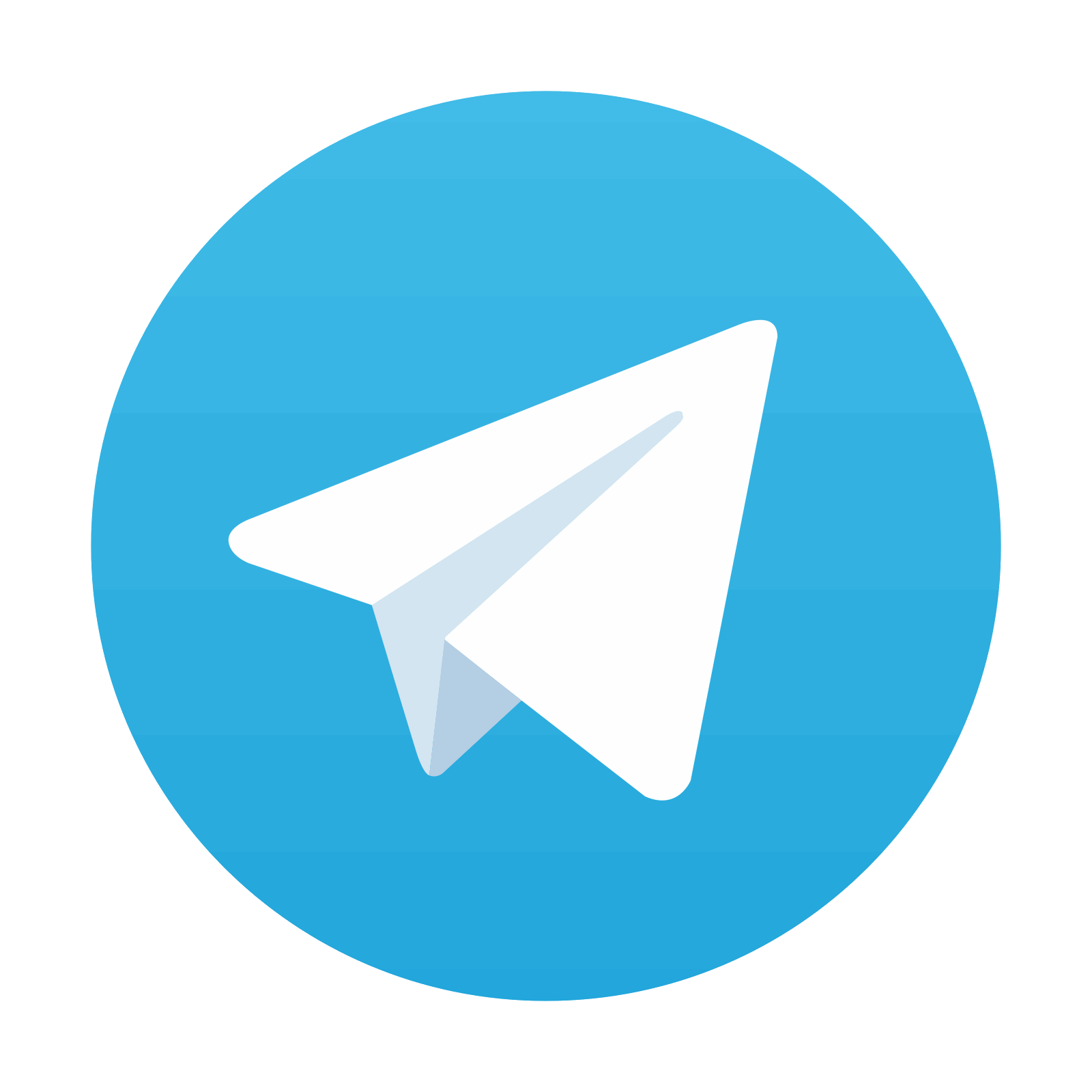
Stay updated, free articles. Join our Telegram channel

Full access? Get Clinical Tree
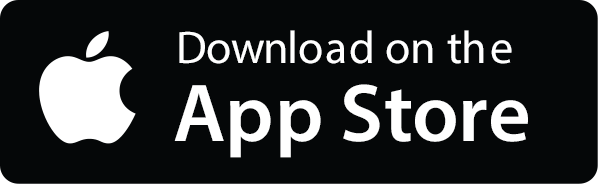
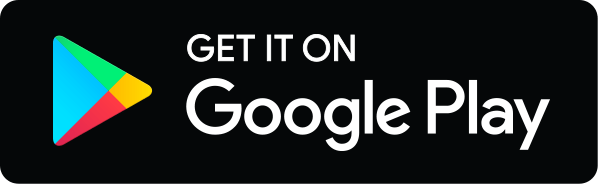
