Osteosarcoma and Other Primary Tumors of Bone
Primary malignant tumors of bone are rare neoplasms accounting for <0.2% of all cancers. In 2010, an estimated 2,650 new cases and 1,460 related deaths were expected.1 Osteosarcoma, chondrosarcoma, and Ewing sarcoma are the most common, comprising 35%, 30%, and 16% of cases, respectively. Other rare entities include malignant fibrous histiocytoma, fibrosarcoma, and chordoma. Ewing sarcoma is discussed in detail in Chapter 88. Osteosarcoma and the other malignant bone tumors will be discussed here.
OSTEOSARCOMA
Epidemiology
Osteosarcoma is a rare primary malignant tumor of bone, accounting for approximately 750 to 900 new cases in the United States annually. Despite its rarity, osteosarcoma is the fifth-most-common malignancy and the most common malignant bone tumor in children and adolescents.2,3 It has a bimodal age distribution, with peak incidence in early adolescence and another smaller peak in adults older than 65 years of age.4 In childhood, osteosarcoma typically occurs sporadically, whereas in adulthood it is more commonly associated with sarcomatous degeneration of Paget’s disease or other benign bone lesions. There is a slight male predilection, with a ratio of 1.2:1.2
Pathogenesis and Risk Factors
The etiology of osteosarcoma is unknown, but there is a suggestion of a relationship with rapid bone growth. The peak incidence of osteosarcoma occurs during the adolescent growth spurt; this peak is earlier in girls corresponding to their earlier bone development. The most frequent sites of involvement correspond to the areas of greatest increase in bone length—the metaphysis of the distal femur, proximal tibia, and proximal humerus. It has been suggested that an aberration in the natural process of bone growth leads to osteosarcoma, but the specific etiology has not been elucidated. Unlike other pediatric tumors, no characteristic translocation or genetic abnormality has been defined for osteosarcoma.
Several risk factors have been associated with osteosarcoma. Development of osteosarcoma after radiation therapy exposure in childhood has been reported. The mean latency period is generally >10 years.5–7 Similarly, chemotherapy, especially alkylating agents, has been implicated with secondary osteosarcoma.7 Benign bone lesions, particularly Paget’s disease, have also been associated with osteosarcoma. Paget’s disease of bone is a focal skeletal disorder characterized by accelerated bone turnover. Sarcomatous transformation is usually seen in long-standing Paget’s and occurs in only 0.7% to 1% of cases.8 Other benign bone lesions have also been associated with risk of osteosarcoma, including chronic osteomyelitis, multiple hereditary exostoses, fibrous dysplasia, osteochondromas, enchondromas, sites of bone infarcts, and sites of metallic implants.9 Several genetic conditions have been linked to an increase risk of osteosarcoma. Retinoblastoma is associated with an increased risk of secondary tumors, more than half of which are soft-tissue sarcomas and osteosarcomas.10 Li–Fraumeni syndrome is associated with a spectrum of malignancies, including breast, adrenocortical, brain, leukemia, and sarcomas, including osteosarcoma. Li–Fraumeni syndrome involves a germline inactivation of p53, a key cell cycle regulatory gene.11 Rothmund–Thomson, Bloom, and Werner syndromes have also been associated with osteosarcoma.12
Clinical Presentation
Most patients present with localized pain in the affected bone. Pain is usually of several months duration and may wax and wane. There may be associated soft-tissue swelling or a palpable mass. Some patients present with pathologic fracture. Osteosarcoma has a predilection for involvement of the metaphysis of long bones. The most common site of involvement is the knee (distal femur or proximal tibia), followed by the proximal humerus, mid and proximal femur, and then other bones.13 Although most patients have micrometastatic disease at the time of presentation, only 10% to 20% of patients present with clinical evident macrometastases. The lung is the most common site of metastatic involvement, followed by bone.14
Diagnostic Evaluation
Plain x-ray of the affected bone classically demonstrates destruction of the normal trabecular bone with lytic and/or sclerotic lesions, osteoid formation under the periosteum (Codman’s triangle), and variable ossification of the associated soft tissue mass (Fig. 82.1). Magnetic resonance imaging (MRI) of the affected bone is essential to fully delineate the extent of the lesion, evaluate any soft-tissue component, and evaluate for involvement of joint, nerves, and vasculature (Fig. 82.2). The entire affected bone should be imaged to evaluate for the presence of skip lesions. Skip metastases are well recognized in osteosarcoma but occur infrequently, with <5% incidence.15,16 Systemic staging should include a computed tomography (CT) scan of the chest and radionuclide bone scan to evaluate for pulmonary and bone metastases, respectively. Positron emission tomography (PET) scan can be used as an alternative for systemic staging but may have less sensitivity than CT and bone scan.17,18 PET scan has also been used to assess response to preoperative chemotherapy.19
Biopsy of the tumor should be performed to confirm the diagnosis and to differentiate from other bone lesions. Similar to soft-tissue sarcomas, the biopsy should be performed at a center with expertise in bone tumors and should be carried out by or in conjunction with the orthopedic surgeon who will be performing future definitive surgery in order to not jeopardize subsequent treatment, particularly a limb-preserving procedure.
FIGURE 82.1. A: Plain radiograph of a distal femur osteosarcoma showing a lytic region and Codman’s triangle in the medial distal femur. B: Magnetic resonance image scan of the same lesion.
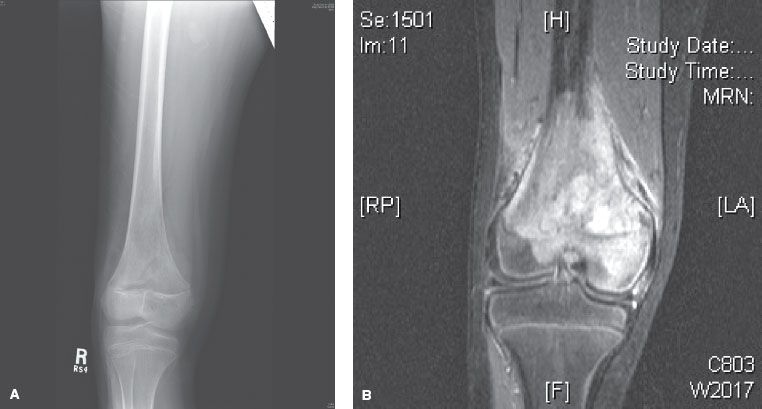
FIGURE 82.2. Plain radiograph of a sclerotic pelvic osteosarcoma.
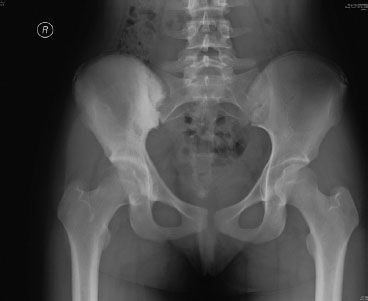
TABLE 82.1 STAGING OF OSTEOSARCOMA
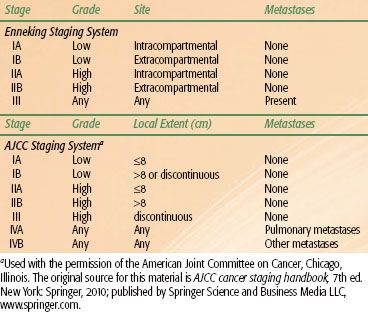
Staging Systems
There are two staging systems commonly used for osteosarcoma (Table 82.1). The Musculoskeletal Tumor Society (MSTS) staging system is a surgical staging system stratifying tumors by grade and subdividing by local extent.20 The American Joint Committee on Cancer system is less often used.21
Pathology
Osteosarcoma is characterized by the presence of malignant sarcomatous stroma with associated osteoid (immature bone) production.22 Osteosarcoma is believed to arise from mesenchymal stem cells with the capacity to have fibrous tissue, cartilage, and bone differentiation. Thus, osteosarcoma shares many features with chondrosarcomas and fibrosarcomas. However, only osteosarcoma produces woven bone matrix, a key element for diagnosis.
Osteosarcoma is classified into two main categories: conventional (intramedullary) and surface.23 The conventional type accounts for 90% of osteosarcomas and is associated with the typical presentation in adolescence. The majority of conventional osteosarcomas are high-grade tumors. Conventional osteosarcoma is further subdivided into osteoblastic, chondroblastic, fibroblastic, and mixed subtypes. Other, less common histologic variants of conventional osteosarcoma include small cell, telangiectatic, malignant fibrous histiocytoma, and multifocal. Multifocal osteosarcoma typically carries a worse prognosis.
Surface osteosarcoma is subdivided into parosteal, periosteal, and high-grade surface.24,25 Parosteal variant is considered to be a low-grade tumor with a low metastatic potential. Periosteal variant is intermediate in grade, with an intermediate rate of developing metastases, between parosteal and conventional osteosarcoma, about 20%. A rare variant, extraosseous osteosarcoma, arises in soft tissues and is generally associated with prior radiation exposure.26
Treatment
Chemotherapy plays a critical role in the management of most patients with osteosarcoma. Although only 10% to 20% of patients present with overt metastatic disease, the vast majority of patients harbor subclinical metastatic disease at the time of presentation. Before effective chemotherapy, 80% to 90% of patients subsequently developed distant metastases and died of their disease despite achieving local disease control.27 The typical treatment sequence for intermediate- and high-grade osteosarcoma is neoadjuvant chemotherapy, followed by surgery with a limb-sparing procedure if possible, and then followed by further adjuvant chemotherapy. With this approach, 60% to 70% of patients without overt metastases at diagnosis are expected to be long-term survivors.28 Those with isolated lung metastases have an overall survival of 35% to 40%, whereas those with more extensive metastatic disease at diagnosis have <20% likelihood of long-term survival. For the less common low-grade tumors such as parosteal osteosarcoma, treatment with surgery alone is appropriate because the risk of developing metastases is low. These patients have an 80% to 90% likelihood of long-term survival.29
Surgery
The mainstay of surgical management is the complete en bloc resection of tumor. The extent and functional implications of surgery have dramatically evolved over time, with an emphasis on more conservative, limb-sparing resections with maintenance of function rather than amputation. Neoadjuvant chemotherapy has played an important role in this evolution.
For extremity lesions, limb preservation is preferred and can be accomplished in the majority of cases. Retrospective studies have shown equivalent results of limb-sparing surgery and amputation as long as adequate margins can be achieved.30–32 Contraindications to limb-sparing surgery include nerve or vascular encasement, presence of large, biopsy-related hematoma, and pathologic fracture. Some data suggest that pathologic fracture does not increase the risk of local recurrence after limb-sparing surgery as previously believed.33,34 Reconstructive options include use of allografts, endoprostheses, and occasionally rotationplasty.
Axial tumors, although much less common, pose a particular challenge because achieving complete surgical resection can be difficult. As a result, these lesions have a worse prognosis compared to extremity tumors. Pelvic tumors typically require a hemipelvectomy for en bloc resection. Some patients can undergo resection of the hemipelvis with preservation of the extremity (internal hemipelvectomy). This has a better functional outcome compared to an external hemipelvectomy, also referred to as a hindquarter amputation. Adjuvant radiation has been used to improve outcomes in patients with incomplete resections of pelvic tumors. Spinal tumors are also particularly difficult to resect with negative margins. Typically, an en bloc resection with vertebrectomy is performed, combined with mechanical stabilization. Postoperative radiation therapy can be used when negative margins cannot be obtained, particularly when there is microscopic dural involvement.
Chemotherapy
In the absence of chemotherapy, 80% to 90% of patients will subsequently develop distant metastases.27 Chemotherapy thus plays an important role for all patients with intermediate- and high-grade tumors. Level I evidence for the benefit of chemotherapy was established by two randomized trials in the 1980s. Eilber et al.35 reported on 59 patients with nonmetastatic osteosarcoma randomized to surgery followed by observation versus adjuvant chemotherapy. Disease-free survival at 2 years was 55% with chemotherapy and 20% with observation (p < .01). Overall survival was also superior at 2 years: 80% versus 48% with and without chemotherapy, respectively (p < .01). Link et al.36 reported similar results in a group of 36 patients with nonmetastatic, high-grade osteosarcoma randomized to observation versus adjuvant chemotherapy after primary surgery. Disease-free survival at 2 years was 66% with chemotherapy and 17% with observation (p < .001).
The concept of neoadjuvant chemotherapy arose in conjunction with evolving surgical techniques striving for limb-preserving procedures and improved functional outcomes. This led to a randomized clinical trial by the Pediatric Oncology Group (POG).37 POG 8651 randomized patients with nonmetastatic, high-grade osteosarcoma to neoadjuvant chemotherapy followed by surgery or surgery followed by the same chemotherapy. The 5-year relapse-free survival was not statistically different between the two groups (65% vs. 61%, respectively), nor was the rate of limb salvage (55% vs. 50%, respectively). Although this trial did not show improved outcomes with neoadjuvant chemotherapy, it did show equivalence and established a benchmark for comparison with future trials. Neoadjuvant chemotherapy is favored by most centers, with the belief that the likelihood of limb-sparing surgery and, ultimately, functional outcome can be improved with this approach. Furthermore, the response to neoadjuvant chemotherapy has been shown to be prognostic.38 This allows for the stratification of patients for more intensive postoperative treatment.
The optimal choice of chemotherapy and administration schedule remains a subject of active research. The Memorial Sloan-Kettering Cancer Center T10 regimen is frequently used for nonprotocol patients and consists of high-dose methotrexate, doxorubicin, bleomycin, cyclophosphamide, and actinomycin D.39 Patients are being accrued to EURAMOS I (AOST 0331), an international collaborative group trial sponsored by European and American Osteosarcoma Study Group, as well as by other groups, including the Children’s Oncology Group.40 This trial is evaluating the benefit of additional chemotherapy after preoperative and postoperative chemotherapy consisting of methotrexate, doxorubicin, and cisplatin. Patients with a poor response to preoperative chemotherapy are randomized to the addition of ifosfamide and etoposide, whereas those with a good response to preoperative chemotherapy are randomized to the addition of interferon.
Radiation
Historically, radiation has been used for the treatment of osteosarcoma; however, high local failure rates with the use of radiation alone, improved surgical techniques, allowing for limb preservation, and effective use of chemotherapy limits the use of radiation therapy for osteosarcoma today. With a combined approach of chemotherapy and surgery with negative margins, local control rates of 90% to 98% have been reported.30–32,41
Patients who have tumor resection with inadequate or positive margins or who have unresectable tumors, however, have high rates of local recurrence. The Cooperative Osteosarcoma Study Group (COSS) performed a multivariate analysis of 1,702 patients and found that poor response to neoadjuvant chemotherapy and incomplete surgical resection predicted negatively for overall survival.42 Picci et al.43 also reported that local recurrence was higher for limb-salvage surgery if wide, negative margins were not achieved. Furthermore, high recurrence rates have been reported in locations where complete surgery is usually not possible, including a recurrence rate of 70% in the pelvis, 68% in the spine, and 50% in the skull regions.44–46 Radiation can potentially improve local control in these patients. Therefore, indications for integration of radiation therapy with other treatment modalities currently include incompletely resected tumors with positive margins and unresectable tumors or for palliation of symptoms.
Radiation Therapy Techniques
As with other sarcomas, proper patient position at the time of simulation and treatment is essential to achieve optimal tumor coverage and normal-tissue sparing. Customized immobilization devices may need to be constructed to achieve optimal positioning that is reproducible on a daily basis. Three-dimensional treatment planning with the aid of presurgical and postsurgical imaging is used to define gross tumor volumes and areas of subclinical disease. Typically, a 2-cm margin is used for axial tumors, which can be extended to 4 to 5 cm for extremity tumors. These margins can be restricted at natural tissue and fascial boundaries. The radiation technique used, either three-dimensional conformal or intensity-modulated radiation therapy, should be tailored to the individual patient. Dose to uninvolved organs should be minimized to prevent late organ dysfunction, as should the integral dose to minimize risk of secondary malignancy.
A prescription dose of 60 Gy in 2-Gy fractions is typically used for microscopically involved margins, whereas 66 Gy is used for macroscopic residual disease and 70 Gy is used for inoperable tumors. Chemotherapy should not be interrupted to deliver local radiation therapy. Radiation can be given concurrently but is usually delivered after chemotherapy due to increased acute toxicity with concurrent administration.
Intraoperative radiation therapy has been used to deliver dose directly to close or involved surgical margins.47,48 Proton particle therapy has been used in an attempt to escalate radiation dose, particularly in unresectable tumors.49,50 Radionuclide therapy with rhenium,51 strontium,52 and samarium53 has been used for palliation of extensive bone metastases with good effects.
Results of Radiation Therapy
In the prechemotherapy era, Cade54 pioneered a technique of radiotherapy with delayed amputation in patients who did not develop distant metastases. The primary tumor was controlled in some patients who refused amputation. However, the overall results were poor, with most patients dying of metastatic disease. The incorporation of chemotherapy with optimal surgery has resulted in significantly improved outcomes, obviating the need for radiation therapy for most patients. Dincbas et al.55 evaluated the addition of preoperative radiation therapy to chemotherapy followed by limb-sparing surgery. They reported on a series of 46 patients, most of whom received 35 Gy in 10 fractions. Local control and overall survival rates at 5 years were 97.5% and 48.4%, respectively. Although the results were excellent, it is not clear whether preoperative radiation therapy improved outcomes, given that the rate of local control with chemotherapy follow by surgery in the absence of adjuvant radiation is high, as previously summarized.
For patients who have incomplete tumor resection or unresectable tumors, the risk of local recurrence/progression is high. These patients, therefore, can potentially benefit from radiation therapy. Machak et al.56 reported on a series of 187 patients with nonmetastatic osteosarcoma treated with induction chemotherapy. Of these, 31 patients refused surgery and were treated with radiation to a mean dose of 60 Gy. Local control was related to response to induction chemotherapy. There were no local recurrences in 11 patients who had a good response to chemotherapy. However, local progression-free survival was 31% at 3 years and 0% at 5 years for nonresponders. Schwarz et al.57 reported on an analysis of 100 patients treated with radiation therapy in the COSS registry. Local control and overall survival for the whole group were 30% and 36%, respectively, at 5 years. Local control was significantly better when surgery was combined with radiation compared to radiation alone: 48% vs. 22%, respectively (p = .002). Local control was also higher for primary tumors compared with recurrent tumors: 40% vs. 17%, respectively. DeLaney et al.50 reported on the Massachusetts General Hospital (MGH) experience. Forty-one patients with osteosarcoma underwent radiation for close or positive margins or for unresectable disease. Anatomic sites included 17 skull, 8 extremity, 8 spine, 7 pelvis, and 1 trunk. Patients received a median dose of 66 Gy (10–80 Gy), with about half of patients receiving a portion of their treatment with protons. The overall local control rate was 68% at 5 years. Local control was similar between patients who underwent a gross total resection or subtotal resection but was significantly better than for those who underwent biopsy only: 78% versus 78% versus 40% at 5 years, respectively (p < .01).
Overall, it appears that radiation is most effective when the tumor burden is small, with the best results achieved in patients who have a good response to chemotherapy or are able to undergo gross total or subtotal tumor resection. Total dose may also be an important factor. Gaitán-Yanguas58 showed a dose–response relationship for osteosarcoma, with no lesions controlled at doses ≤30 Gy and all lesions controlled at doses of >90 Gy. The best clinical results were reported in the MGH series.50 A median dose of 66 Gy was used, and half of the patients received proton therapy as part of their treatment. The unique dose–depth properties of proton radiation therapy allows for dose escalation while maintaining normal-tissue sparing. This approach may explain the improved outcomes reported. However, this study did fail to show a dose–response relationship.
Whole-Lung Irradiation
Whole-lung irradiation has been shown to be beneficial in several other pediatric tumors with a propensity for lung metastases. This led to the rationale that this treatment may improve outcomes in osteosarcoma, which has a high propensity for metastases and for which the lung is the most common site of spread. Two initial small randomized trials in the prechemotherapy era showed a trend for improved disease-free and overall survival for whole-lung irradiation.59,60 This led to the EORTC-20781/SIOP-03 phase III trial, which randomized 240 patients to three arms: chemotherapy, whole-lung irradiation, or both.61 The whole-lung dose was 20 Gy. The 4-year disease-free survival and overall survival were 43% and 24%, respectively, with no difference between the arms. Therefore, with the recognition of the other advantages of systemic therapy, whole-lung irradiation has fallen out of favor.62
TABLE 82.2 SELECTED STUDIES OF BASE-OF-SKULL CHORDOMA AND CHONDROSARCOMA
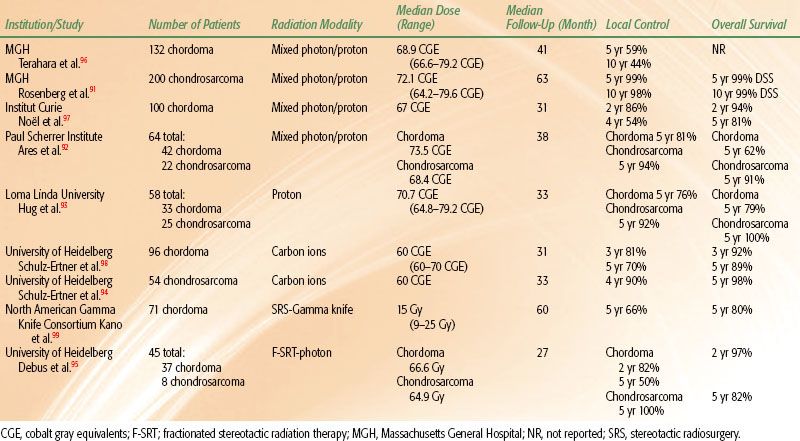
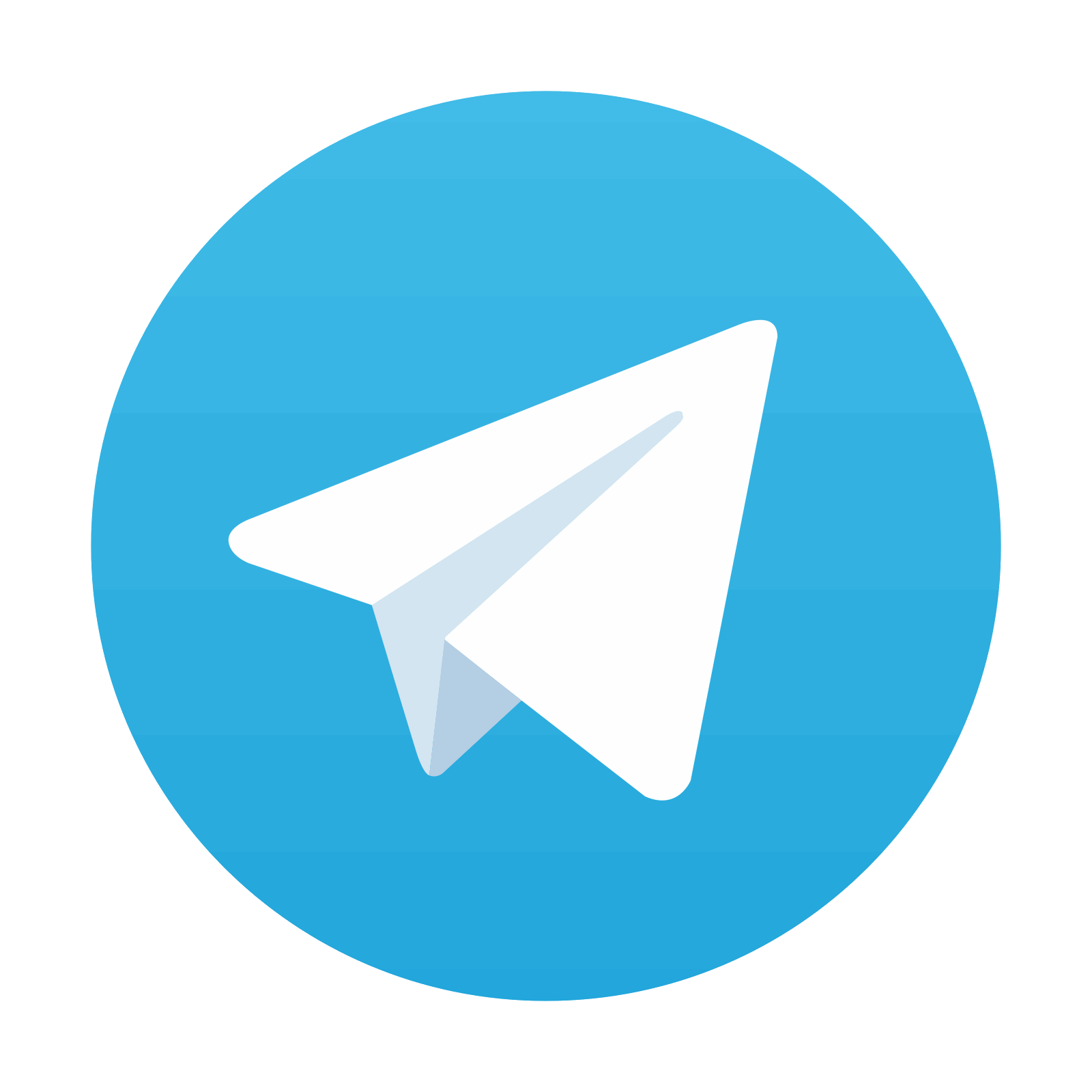