Genes related to the lipid homeostasis
Fabs
The polymorphisms have been studied in several metabolic phenotypes like obesity, metabolic syndrome, hypertriglyceridemia, and insulin sensitivity [6]
ELOVL6
Related with insulin resistance [5]
ApoE
Genes related to the energy metabolism
UPC
Uncoupling proteins have been considered predictive genes for obesity, T2D, and IR [5]
ADRB
The polymorphisms were associated with serum insulin levels and IR [9]
Genes encoding hormones and hormone receptors
AMP1, ADIPOR1, ADIPOR2
Polymorphisms are strongly associated with metabolic syndrome, obesity, and IR [10]
TSHR
Related with IR in T2D [5]
SHBG
Polymorphisms in genes encoding sex hormones may be effective on the development of IR [5]
LEP, LEPR
Leptin and its receptors are predictive genes for IR [5]
RPB4
Retinol-binding protein 4 is an adipokine that potentially contributes to systemic IR [11]
RETN
Resistin has been examined as a predictive gene for obesity, T2D, and IR [5]
Genes related to the renin-angiotensin system (RAS)
The RA system plays a pivotal role in the regulation of insulin sensitivity [5]
Genes related to the inflammation
TNFα
Increased levels of TNFα have been shown to increase the risk of IR [12]
IL-6
Several studies have tried to understand when the earliest metabolic changes start to arise during life, and some “critical periods” have been identified: prenatal life, early postnatal period, and puberty [15]. There is a physiologic insulin resistance during puberty: a reduction of 25–50 % in insulin sensitivity with recovery at complete pubertal development has been demonstrated [16]. Hannon et al. have confirmed these data, showing a reduced glucose oxidation and an enhanced lipolysis. So, pubertal transition may be a major risk factor in both obese subjects and in those with a “thrifty phenotype” [17]. A 9-year longitudinal study has suggested that IR may start in mid-childhood, 2 years before puberty [18]. A relevant fat mass deposition during this age, high plasma IGF-1 levels, and adrenarche-induced peripheral insulin resistance could contribute to an earlier onset [19]. The causal association between nutrition and the onset of insulin resistance, type 2 diabetes (T2D), obesity, and metabolic syndrome during adulthood has been clearly demonstrated [20–23]. The metabolic impairment seems to begin during fetal life; intrauterine environment and fetal feeding modify metabolism programming later in life: high-fetal glucocorticoids caused by intrauterine growth restriction (IUGR) or exposure to stressors (such as famine) may play a critical role [24–26]. This predisposition to metabolic syndrome continues during early postnatal life, in toddlers, and during childhood and adolescence (the abovementioned “thrifty phenotype”) [27, 28].
For this reason, it is becoming of primary relevance to understand the mechanisms that induce insulin resistance in children and adolescents because of the increasing prevalence of obesity, type 2 diabetes (T2D), and metabolic syndrome in these periods of life [29]. The increasing emergency of obesity and T2D during childhood and adolescence and the rapid development of an impaired glucose metabolism explain how these diseases are becoming very notable emergency in public health [30]. The most relevant dietary risk factors for insulin resistance are summarized in Table 9.2.
Table 9.2
Dietary habits as risk factors of insulin resistance
Prenatal period | Undernutrition |
Low-protein intake | |
Low vitamin B12 and folate intake | |
Overnutrition | |
High-fat diet | |
High-glycemic index (GI) | |
Infancy | Short breastfed duration |
High-protein formula fed | |
Postweaning high-fat/high-sugar diet | |
Childhood and adolescence | Higher total sugar intake |
Sugar-sweetened beverages and other liquid source of sugar | |
Increased intake of high-energy foods and sweet snacks | |
High-GI and high-fat breakfast | |
Saturated fatty acid (SFA)-enriched diet | |
Breakfast skipping |
9.2 Nutrition During Pregnancy: The Role of Lactation
Maternal nutrition during pregnancy has been a central topic of debate in recent years. Maternal diet has a direct effect on fetal growth and development: the quality and quantity of maternal nutrition influence the supply to the fetus of the required nutrients, exerting lifelong effects on morbidity and mortality risk. Furthermore, maternal feeding influences the hormonal pathways that control fetal and maternal metabolism (“metabolic programming”) [31]. Both overnutrition and undernutrition have been analyzed in animal and human models as risk factors for metabolism impairment that involves not only early childhood but also adolescence and adulthood.
An abnormal supply of nutrients with diet induces physiologic changes in metabolic pathways: when the fetus is exposed to a restricted maternal diet, an appropriate caloric supply must be ensured to the brain for its growth and development. However, this causes a reduction of energy supply for other non-vital organs through a peripheral insulin resistance, increasing the chance of fetal survival and the amounts of glucose to the brain [32, 33]. By contrast, maternal overnutrition is associated with a higher risk of obesity, metabolic syndrome, and cardiovascular diseases in the offspring, above all if there is a relevant maternal weight gain or BMI during pregnancy or higher neonatal weight (large for gestational age – LGA) and higher birth centile before weaning [34–36].
A low-fat diet during pregnancy and lactation prevents an irreversible and inheritable form of T2D in the offspring of obese mice with T2D [37, 38]. Instead, a high-fat diet could impair the function and the vascularization of pancreatic islets [39, 40], resulting in glucose intolerance and insulin resistance. Furthermore, the high-fat diet seems to stimulate β-cell replication so that β-cell mass increases [41]. Free fatty acids (FFAs) regulate glucose-induced insulin release by a pathway that involves a receptor for FFAs combined with G proteins. Moreover, a higher fat mass deposition in offspring leads to hepatic steatosis [42, 43].
The role of protein intake in the development of the impairment in glucose metabolism, insulin resistance, and later compensatory hyperinsulinemia has been largely considered: in the rat model of low-protein diet, several tissues are involved, such as the liver, the skeletal muscle, and the adipocytes [20]. The metabolic effects of a protein-restricted diet include an impaired insulin secretion, a higher gluconeogenesis, and a lower FFA β-oxidation when a common western diet is started [44]. However, dysmetabolic and cardiovascular risks in an offspring have been also associated with a high protein intake during pregnancy: a high-protein diet together with a low-carbohydrate intake has noxious consequences (high blood pressure and adrenal cortisol secretion) in stressful situations [45]. Proteins from animal sources, especially meat products, eaten during pregnancy may increase the risk of overweight and obesity in offspring in both sexes [46].
Glucose is the most used energy source for cells to guarantee the normal execution of physiologic processes. It is the main fuel of fetal tissues and it supports the growth and the development since the earlier phases of intrauterine life [47]. An important index that has been largely studied for its probable association with insulin resistance onset or prevention is glycemic index (GI): it measures how a carbohydrate-containing food raises blood glucose when compared to a reference food (either glucose or white bread) [48]. A maternal diet with a low GI during pregnancy reduces the physiologic maternal insulin resistance and glucose tolerance impairment, and it limits maternal weight gain. However, there are conflicting data about the effects of GI on fetus and newborn size: some authors have not found any difference in the incidence of LGA infants [49, 50]. By contrast, Tzanetakou et al. showed a limitation of the risk of LGA newborn when exposed to maternal low-GI diet [51]. At the same time, there are evidences of an increased incidence of infants born small for gestational age (SGA) after an intrauterine exposure to a maternal low-GI diet. This could be linked to lower circulating levels of blood glucose and thus less fuel for fetal growth and development [52]. Moreover, a maternal diet with low carbohydrates eases recurrent episodes of hypoglycemia: they seem to limit beta-cell maturation and replication, with a reduction of Langerhans islet volume and vascularization; this mechanism could predispose to IR and diabetes mellitus [53]. A few studies have correlated high maternal GI diets to the risk of LGA infants, and they have demonstrated a higher prevalence of LGA offspring [35]. The GI could modify leptin synthesis that controls appetite and eating patterns. So, GI could play a pivotal role in this process of metabolic programming since fetal life until adulthood [54]. Glycemic load (GL) is another metabolic index that has been largely evaluated for its feasible association with insulin resistance and the markers of metabolic syndrome. GL is a practical way to apply the GI and it is calculated from GI and the number of net carbohydrates in a given serving. So it gives an indication of how much that serving of food is likely to increase the glycemia. To date, not any correlation has been demonstrated with insulin secretion and infant size [52, 55].
Eventually, several studies have evaluated the role of vitamins in a fetus and later risk of disease onset [56]. Micronutrients are essential for antioxidant and anti-inflammatory processes, and pancreatic β-cells are particularly sensitive to oxidative stress [57, 58]. For example, the Pune Maternal Nutrition Study demonstrated that high maternal circulating levels of homocysteine predict the risk of SGA infant, while low vitamin B12 and high folate levels are associated with higher HOMA-IR and fat mass [59]. The deficit of vitamin B12 and the resulting homocysteine accumulation could lead to a restricted protein synthesis [60], an inhibition of beta-oxidation of FFAs [61], and a modulation of DNA methylation [62].
The dietary habits after birth play a critical role in the risk of excessive weight gain and the later risk of obesity, IR, metabolic syndrome, and cardiovascular diseases. Newborns are fed with a wide variety of choices, from breastfeeding to artificial formulas that have shown different and conflicting effects on health. Breastfeeding is suited to the nutritional needs and the rapid growth of the infant. Moreover, breast milk contains micro- and macronutrients that play important functions, such as protection against infections; reduction of the risk of obesity, diabetes, and eczema; and support of the neurodevelopment [63]. Lactation duration has also been largely analyzed. Breastfeeding has been recommended for at least the first 6 months of life, but it could be prolonged until the second year along with complementary foods [64]. However, there are conflicting data: while Veena et al. have shown lower HOMA-IR values and a reduced incidence of glucose intolerance after 6 months of breast milk [65], other studies have demonstrated a constant relevant risk of cardiac and metabolic diseases and IR even after an exclusive long-lasting breastfeeding [66]. Breastfed infants are leaner and have a lower risk of hypertension, obesity, IR, and dyslipidemia later in life when compared to formula-fed infants, due to a restrained postnatal weight gain and catch-up growth [46, 67–70]. Despite a lot of observational studies, there are only few experimental studies about the effects of breastfeeding on later health outcomes; hence, the data should be interpreted with caution. The protein intake during lactation has been widely argued. The “early protein hypothesis” postulates that a high protein intake during infancy and early childhood results in a metabolic programming of later obesity as a result of adverse hormonal responses [71]. A correlation between an elevated protein intake during 24 months and the risk of overweight and obesity has been demonstrated: a 24-month clinical trial of EU Childhood Obesity Programme has shown higher weight-for-length ratio and BMI in high-protein formula-fed children compared to breastfed or low-protein formula-fed infants [72]. Postnatal high-protein diet facilitates fat mass deposition and a weight gain of 10–40 % during adulthood [73]. Insulin and IGF-1 synthesis could ease adipocyte growth and differentiation during early childhood, predisposing to obesity [74]. The so-called cafeteria diet (high-fat, high-sugar diet) would lead to similar outcomes [34], while the opposite effects have been ascribed to a low-protein formula [75].
9.3 Epigenetics: What Is Its Role?
In the recent past, the fetal development has been also analyzed from a different point of view: the link between the maternal nutrition and epigenetics. Epigenetics includes the changes in gene expression without alterations of DNA sequences transmitted through mitosis or meiosis. Individual epigenetics could be modified throughout life by environmental factors that interferes with DNA methylation, histone acetylation/methylation, mRNA expression, or chromatin looping [76]. So, wrong nutritional and environmental stimuli during fetal development could lead to a permanent loss of imprinting. IGF-2 gene is one of the most important loci analyzed for its labile methylation and impaired imprinting in both human and animal models [77]. Fetal and neonatal nutrition influences food intake and energy balance later in life, and leptin seems to be involved in the energy homeostasis. Epigenetic also seems to involve leptin gene expression. Leptin could facilitate the development of the hypothalamic nuclei that regulate nutrition and dietary behavior, with effects on neuronal and adipocyte plasticity [78]. There are two sources of leptin during pregnancy: placental leptin that mediates placental growth and nutrient transport [79] and fetal leptin that is secreted into the amniotic fluid. It induces insulin release and IGF-1/GH pathway activation that influences fetal growth and development, catabolic reactions, and adipose mass distribution [80]. Leptin acts on adipose cells and other tissues since they contain abundant leptin receptors [81]. Leptin enhances metabolic rate and facilitates muscular insulin sensitivity, but it impairs insulin binding to the receptors of adipose tissue (diabetogenic trait) [82]. Obesity heightens this pathway [83]. The presence of leptin receptors on pancreatic β-cells suggests that leptin may influence insulin secretion in obese and insulin-resistant subjects [84]. In summary, leptin establishes a link between peripheral tissue-derived information and the central nervous system network [82].
9.4 Nutrition in Children and Adolescents
The risk of obesity, metabolic syndrome, impaired glucose metabolism, T2D, and cardiovascular diseases lasts during childhood and adolescence, and eating behaviors play a crucial role [85]. A proper diet and a healthy lifestyle should be proposed since first years of life: the introduction of a great variety of foods provides for the caloric requirement of the toddler. From the very earliest age, children’s experiences with food influence both preferences and intake [86]. The school age is a time in which the parents can experience some problems with the feeding of their children. The children will spend more time away from home, and the supervision of their daily diet will become more difficult. On the other hand, the nutritional requirements of the child will change with the increase in physical and intellectual activities. The parents have to inculcate the good habits of feeding and give to them a varied diet [87].
An emerging problem is the meal skipping and the need of adequate inter-meal intervals. A good nutrition begins with the first food of the day: the breakfast is the first ingestion after the longest fasting period of the day, especially for children that sleep more hours than the adults. Hence, it is important not to eliminate the breakfast from the normal diet. Breakfast supplies micro- and macronutrients and an adequate daily energy contribution to favor a proper growth. In a randomized crossover trial, three different groups (low-GI breakfast; low-GI breakfast with added sucrose; high-GI breakfast) were compared, and it was highlighted that low-GI breakfasts with or without added sucrose caused a reduced lunch intake and a relevant satiety compared to daily high-GI breakfasts. So, low-glycemic index may have a role in preventing obesity and impaired glucose metabolism [88]. Unfortunately, breakfast skipping has been increasing in western countries. Recent studies have reported an association between this behavior and the risk of overweight or obesity, IR, and T2D since childhood [89–94], because breakfast skipping seems to increase the frequency of a snacking behavior for hunger. The HELENA study underlined that there are differences for breakfast habits between sexes and between north and south Europe. Moreover, a significant difference was seen in BMI, skinfold thickness, and HOMA-IR among breakfast consumers, occasional consumers, or skippers [95]. Donin et al. in the Child Heart and Health Study in England (CHASE) have highlighted a higher risk of T2D and cardiovascular diseases (increased fasting insulin levels and IR, glycemia, HbA1c, and blood urate) among breakfast skippers when compared to children having breakfast daily (74 %). Furthermore, breakfast skippers had a lower total energy intake because their diet was poor in carbohydrates but rich in monosaturated fats and highly caloric foods (“scrap foods”) [94]. Mediterranean diet has been considered a well-balanced model since it has demonstrated a clear efficacy in the prevention of IR, obesity, cardiovascular diseases, and metabolic impairment. It is a balanced diet that supplies an adequate amount of complex carbohydrates, polyunsaturated fatty acids, proteins, fibers, and micronutrients [96]. Pérez-Jiménez et al. demonstrated that a diet rich in complex carbohydrates and unsaturated fatty acids (Mediterranean diet) in healthy children and adolescents may improve insulin sensitivity and glucose metabolism, reducing blood glucose and increasing peripheral insulin sensitivity [97]. The Mediterranean diet would improve the BMI, glucose, and lipid profile in children and adolescents with obesity and metabolic syndrome components: it decreases BMI and fat mass, glycemia and dyslipidemia, and the consumption of saturated fatty acids [98].
On the other hand, a high sugar intake has been associated with hyperglycemia, hyperinsulinemia, and IR. This diet is common in western countries, due to a common consumption of sugar-sweetened beverages (SSBs) and energy-dense foods. Vyncke et al. have developed a diet quality index for adolescents (DQI-A) to evaluate three main aspects: dietary quality, dietary diversity, and dietary equilibrium. They have demonstrated that DQI-A was positively related to the intake of fruits and vegetables, while there was an inverse relationship with high-energy and low-nutritious foods [85]. Higher values of HOMA-IR and an impaired glucose homeostasis have been evidenced in those children and adolescents that consumed SSBs several times per week [99, 100]. Similarly, Latinos are at an increased risk of weight gain after a high sugar intake: Davis et al. found a reduced β-cell function and insulin secretion in children consuming foods rich in sugar, such as SSBs [101].
By contrast, vegetable and fruit consumption has been highly recommended [102], because they provide vitamins and elements that have vital functions in a developing organism. However, there are limited data about their effects on adolescent metabolism. An increased insulin sensitivity and a reduced visceral adiposity have been found in overweight vegetable consumers as compared to nonconsumers in a cross-sectional study by Cook et al. [103]. Moreover, the consumption of whole foods has demonstrated beneficial effects on insulin sensitivity and BMI, particularly for the heaviest children and adolescents. The higher intake of whole grain has been also associated with healthier dietary habits, including greater intakes of fruit and vegetables, fibers, iron, zinc, calcium, folate, and vitamins [104]. The greatest limit to the healthy effects of this dietary regimen is the low compliance among western adolescents.
Conclusions
The crucial role of insulin resistance as risk factor for later metabolic syndrome, T2D, and cardiovascular diseases has been clearly demonstrated. In the recent past, a greater attention has been focused on the effects of nutrition on longtime health since intrauterine life. The age at higher risk for future insulin resistance and metabolic impairment is still controversial. Nevertheless, the phases of life more clearly associated with IR remain prenatal period, infancy, and puberty (the so-called critical periods). The prevention of insulin resistance must contemplate an adequate distribution of macro- and micronutrients, a diet rich in fibers and vegetables but poor in saturated fats and simple sugars. The role of GI in the prevention of IR has been also enhanced. Overall, the results of the aforementioned epidemiological and experimental studies suggest the need of intervention studies: the purpose is the prevention of IR since the earliest period of life through a correct quality of diet and dietary habits.
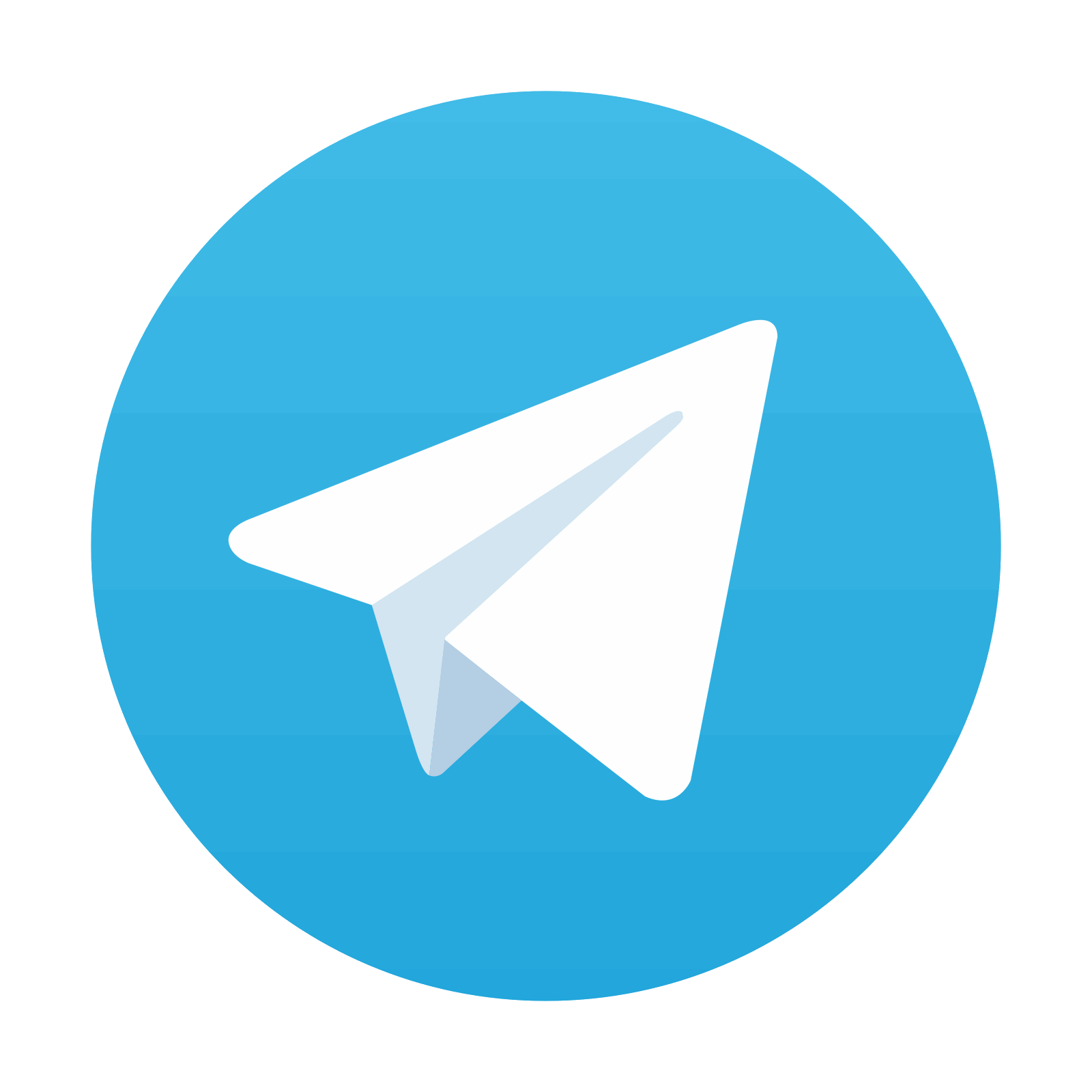
Stay updated, free articles. Join our Telegram channel

Full access? Get Clinical Tree
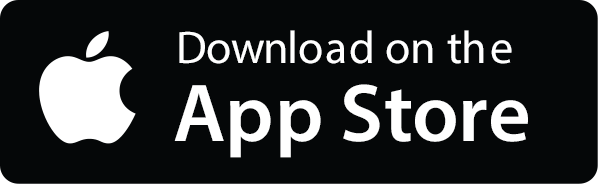
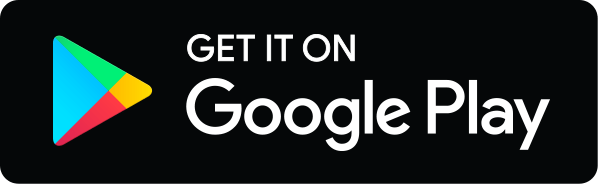