Findings observable on dilated ophthalmoscopy
No retinopathy
Mild nonproliferative diabetic retinopathy (NPDR)
Microaneurysms only
Moderate NPDR
More than just microaneurysms but less than severe nonproliferative diabetic retinopathy
Severe NPDR
Any of the following: more than 20 intraretinal hemorrhages in each of four quadrants, definite venous beading in two or more quadrants, prominent intraretinal microvascular abnormalities in one or more quadrants, and no signs of proliferative retinopathy
Proliferative diabetic retinopathy
One or more of the following: neovascularization, vitreous/preretinal hemorrhage
Clinically significant macular edema
Retinal thickening within 500 μm from center of macula, hard exudates within 500 μm from center of macula with adjacent retinal thickening, retinal thickening of more than one optic disc area within one optic disc diameter from center of macula
According to the ETDRS, nonproliferative DR has been categorized as mild, moderate, severe, and very severe [7].
6.1.2 Natural History
Chronic hyperglycemia appears to be the most important factor in promoting functional changes in the retinal vasculature.
Early histological changes of DR, which occur before any clinical evidence of the complication, consist of pericyte loss and basement membrane thickening. Pericytes are contractile cells in charge of blood flow regulation; they are also involved in the control of proliferation and repair of endothelial cells inside the retinal vascular system. Pericyte loss is associated to the formation of retinal microaneurysms [6, 8].
The development of microaneurysms represents the earliest clinical sign of DR and consists in the development of small dilations of terminal arterioles and/or of small venules caused by proliferation and outpouching of the capillary endothelium in areas of intramural pericyte loss. These signs are present in nearly all the patients who have had type 1 diabetes for at least 20 years and in nearly 80 % of those with type 2 diabetes of the same duration [6].
Small hemorrhages as a result of ruptured microaneurysms and leaking capillaries, deposits of lipid (hard exudates), accumulation of axoplasmic debris from microinfarction of the nerve fiber layer (cotton-wool spots) venous dilation, and, finally, beading and development of intraretinal microvascular abnormalities (IRMA) will appear subsequently [6].
Mild to moderate nonproliferative DR is also referred as background DR, while severe nonproliferative DR is also referred as pre-proliferative DR.
Years of ischemia of the inner retinal layers due to the closing of segments of the retinal capillary system lead, as a response, to the production of vessel-stimulating growth factors such as: vascular endothelial growth factor (VEGF), platelet-derived growth factor (PDGF-B), placental growth factor (PlGF), and stromal cell-derived growth factor (SDF-1) along with angiopoietin 2 (Angpt2). As a consequence a strong stimulus to the development of neovascularization will take place [9].
Proliferative DR is characterized by the appearance of new, fragile, and fenestrated retinal blood vessels penetrating into the inner limiting membrane and, in some cases, also entering the vitreous. These neovessels bleed easily giving rise to fibrovascular scar tissue, retinal tears, and tractional retinal detachment with subsequent blindness [6].
Diabetic macular edema is instead characterized by retinal thickening due to the accumulation of fluid behind the macula (the area of greatest visual acuity of the retina) secondary to microaneurysm formation, breakdown of the blood-retinal barrier, increased vascular permeability, and leakage of fluid and exudates [6, 8]. Diabetic macular edema is the principal cause of vision loss in patients with nonproliferative DR; the incidence of this complication over a 10-year period has been estimated to be around 20.1 % in patients affected by type 1 diabetes [10].
6.1.3 Therapeutic Approaches
As of today, with exception of tight glycemic, blood pressure, and lipidic control, there are no interventions that can prevent or delay the development of DR. There is not even a specific treatment for pre-proliferating forms. Once DR is diagnosed, the patient is followed a long time to assess the progression of the complication, and only in case of proliferative DR or diabetic macular edema, when a serious impairment of vision becomes manifest, specific treatments are applied [4].
Laser photocoagulation is the mainstay of ophthalmic therapy for vision-threatening proliferative DR. This approach consists in placing microscopic laser burns over the entire retina, sparing the central macula, to stop progression and promote regression of retinal neovessels. Despite its remarkable efficacy in the prevention of visual loss, the destructive nature of laser might be associated, in some cases, with ocular side effects as poor response to treatment, pain, nyctalopia, loss of peripheral vision, uveal effusions, worsening of macular edema, and difficulty to treat eyes with vitreous hemorrhage, and in some cases multiple treatments may become necessary [11].
Alternative therapies consist in the intravitreal injection of anti-inflammatory (corticosteroids) and antiangiogenesis (anti-VEGF) agents. Several clinical trials have demonstrated the usefulness of intravitreal anti-VEGF agents to treat both diabetic macular edema and proliferative DR, but serious side effects like cataract, elevation of intraocular pressure, recurrence of neovascularization, tolerance, and increased rate of vascular events (myocardial infarction and stroke) may however accompany this therapeutic approach [12].
Based on these considerations, the importance of understanding the mechanisms involved in the development of diabetic retinopathy becomes clear.
A number of interconnected biochemical mechanisms associated with hyperglycemia have been implicated in the pathogenesis of DR. These include oxidative stress, polyol and hexosamine pathway activation, advanced glycation end product formation, and activation of protein kinase C isoforms. However, the direct link between hyperglycemia per se and visible vascular lesions remains undemonstrated. This explains why a number of agents that have been developed to treat DR through the modulation of the above-described biochemical pathways have not shown beneficial effects or only minor efficacy in subsequent clinical trial [13].
6.1.4 Endothelial Progenitor Cells and DR
During the last decade, a subpopulation of bone marrow-derived cells (endothelial progenitor cells or EPCs) has been described in the peripheral blood. EPCs are able to differentiate in vitro into endothelial cells and can be incorporated in vivo into sites of active angiogenesis. EPCs play an important role in tissue regeneration by promoting the repair of blood vessels and by taking part in the reperfusion of ischemic areas [14].
In response to hypoxia, EPCs move from the bone marrow into the circulating blood to finally localize into the ischemic tissues. The mechanisms by which EPCs are able to migrate and home in specific areas are complex and not yet completely understood [14]. The concept that EPCs, by traveling through the peripheral circulation on their way to the vessel wall, could become (readily accessible) biomarkers of vascular events or vascular status is definitely intriguing. The implementation of these biomarkers could indeed change the therapeutic approach to vascular pathologies with a long subclinical phase.
The knowledge of EPCs is still evolving, and it has become clear that they consist of heterogeneous subpopulations of cells characterized by the expression of different antigens. Currently, circulating EPCs are identified either by flow cytometry on the basis of the combined expression of surface antigens characteristic of hematopoietic stem/progenitor cells (CD34) and of endothelial cells (vascular endothelial growth factor receptor 2 [VEGFR-2]) or on the basis of clonogenicity (i.e., the capability to form colonies in vitro) [15, 16].
On this basis it was shown that at least two types of EPCs can form colonies in vitro. One type, named endothelial colony-forming cells or late outgrowth cells, gives rise to colonies within 7–21 days of plating. The cells of the colonies have the typical morphology and molecular specifications of endothelial cells and can be transplanted to form chimeric blood vessels in vivo. This type of EPCs is positive for a range of endothelial cell markers (including VEGFR-2, VE-cadherin (CD144), CD31, CD105, CD146, and Tie2) and negative for hematopoietic cell markers (such as CD45, CD14, CD133, and CD115) and demonstrates positivity to CD34 and CD117 [17, 18].
A second type of EPCs, in the circulation, has become known as colony-forming units (CFU)-Hill cells or early outgrowth endothelial cells. These cells form colonies within 5–7 days; the cells are round, or spindle shaped at the periphery of the colonies, and are positive for both endothelial markers and hematopoietic (CD45) and monocytic (CD14) lineage markers. These cells do not integrate into the blood vessels but exert proangiogenic effects through paracrine mechanisms [17, 18].
To date the most extensive evaluation of EPCs as informers of vascular status has been done in atherosclerotic vascular disease where a decreased number of circulating CD34 + VEGFR-2+ and of CFU-Hill cells was found to be associated with a larger burden of cardiovascular risk factors [19, 20]. Recent studies have also demonstrated that EPCs are involved also in the pathology and the progression of DR. Accordingly, EPCs were shown to be recruited in the ischemic retina and incorporated into new vessels in animal models of proliferative DR. In parallel, an increased number of circulating CD34+ mononuclear cells in patients with proliferative DR were also reported [21, 22].
In a recent study, performed by our group, which included three groups of type 1 diabetic patients: (a) with long-standing (>23 years) diabetes and no retinopathy, (b) short-standing (<7 years) diabetes and no retinopathy, and (c) diabetes of <20 years standing with nonproliferative DR, both late outgrowth EPCs and CFU-Hills cells were evaluated. As a result, no differences were found in the number of circulating EPCs (CD45-/CD34+/VEGFR-2+) between the different groups of diabetic patients and a control group composed by age- and gender-matched healthy volunteers. However, the number of colonies formed in the Hill assay (when EPCs were grown in vitro) was increased in patients with nonproliferative DR (group c) when compared to patients with long-standing diabetes and no retinopathy (group a) indicating that higher colony counts were associated with the presence of DR. This hypothesis is supported also by another study showing that patients with established proliferative DR are characterized by an increased CFU-Hills assay activity [23].
Moreover in CFU-Hills cells, a reduction of the expression of a number of integrins, uPA (urokinase plasminogen activator), and CXCR4 (a factor involved in the EPCs homing inside the retina) was found. These evidences suggest in case of DR the reduced expression of the homing receptors and integrins deprives the cells of adhesive and migratory functionality and, thus, limits interactions with the vascular wall, a phenomenon that may have consequences facilitating the progression of DR itself [23].
6.2 Diabetic Nephropathy
6.2.1 Background
Diabetic nephropathy (DN) eventually affects about one third of patients affected by type 1 diabetes mellitus. Among the long-term complications of diabetes, DN retains the highest human, social, and economical costs. DN represents a leading cause of end-stage renal failure and the strongest predictor of premature death and cardiovascular disease in type 1 diabetes [24]. The risk of developing this complication is not linear with time, reaches a peak between 10 and 20 years of diabetes, and then declines, thus suggesting that only a subgroup of patients affected by type 1 diabetes may be susceptible to renal complications [25]. Recent clinical evidences suggest that, despite the continuous improvement of clinical protocols aimed to control glucose metabolism and blood pressure, the risk of developing end-stage renal disease as a consequence of DN has not significantly changed during the last 20 years [26]. Altogether these findings suggest that the cellular/molecular mechanisms leading to the development of DN are probably not yet fully understood and that further studies are needed to identify in advance patients predisposed to develop DN (at stages that precede the appearance of microalbuminuria) and, in parallel, to develop new drugs able to prevent the onset of DN rather than to modulate its progression.
6.2.2 The Role of Essential Hypertension
That genetic factors may play a role in the development of DN is suggested by the clustering of this complication in families [27]. Furthermore, arterial blood pressure can be higher in parents of patients affected by type 1 diabetes and proteinuria than in parents of normoalbuminuric patients matched for age and duration of diabetes, suggesting that an inherited predisposition to essential hypertension may be related to the susceptibility to DN in patients affected by type 1 diabetes [28, 29].
This hypothesis was further supported by two independent studies [30, 31] showing an elevated activity of Na–Li countertransport in red blood cells of type 1 diabetic patients with DN. Elevated activity of Na–Li countertransport is presently considered the most consistent intermediate phenotype of essential hypertension [32, 33], and genetic epidemiology studies have suggested that the activity of this membrane transport can be explained by inheritance more than environmental factors, when a major gene and polygenic effects are taken into account [34, 35]. Subsequently, further evidences have confirmed that an increased activity of Na–Li countertransport predicts the development of both essential hypertension [36, 37] and DN [38] and cannot be therefore considered a mere consequence of the development of these human diseases. Studies aimed to identify the genetic component of essential hypertension and DN are presently underway; on this regard the recent demonstration that Na–Li countertransport is actually mediated by the first isoform of Na–H exchange [39], a sodium transport present in virtually all living cells where it is in charge of controlling the rate of proliferation, might potentially lead to the identification of polymorphisms of Na–H exchange associated with the development of essential hypertension and/or DN.
6.2.3 The Role of C-Peptide
Although the natural history of DN is supposed to invariably end with a global glomerular sclerosis and consequent renal failure [40], recent studies demonstrated that long-lasting pancreas transplantation may reverse the glomerular lesions that characterize DN in man [41].
That long-term normoglycemia might by itself be responsible for the regression of lesions of DN is presently under debate [42]. Although the Diabetes Control and Complications Trial (DCCT) demonstrated that a reduction of the risk of developing microalbuminuria and a slower progression of existing nephropathy were associated to the improved HbA1c levels consequent to intensive insulin treatment, no clinical regression of established DN could be associated to this procedure [1, 43].
A major difference between intensive insulin treatment and pancreas transplantation is that the latter not only normalizes plasmatic insulin levels but also restores the physiologic concentration of C-peptide, the product, along with insulin, of the cleavage of proinsulin [44, 45].
Although for long time considered to have no biologic function, C-peptide is now considered as an active peptide hormone, independent of insulin with a specific G protein-coupled membrane receptor [46, 47]. Recent evidences suggest that C-peptide might have a protective role in the development of diabetic complications [48–50] both in animals and in humans. In line with this hypothesis, we have demonstrated the existence of a decreased C-peptide/creatinine ratio in plasma of type 1 diabetic patients with DN when compared to normoalbuminuric patients of similar age and duration of diabetes [51].
How C-peptide can possibly exert its beneficial effect is presently unknown. In animal models the Na–K pump activity is decreased by hyperglycemia, and C-peptide reverses this dysfunction [48]. Activation of Na–K pump by C-peptide was also demonstrated in man [52], but whether the protection of C-peptide from DN is mediated by this mechanism is still unclear, as the activity of the Na–K pump seems not to be decreased in DN [30].
Altogether a huge number of studies in the field did not allow to solve the problem whether C-peptide actually protects from the development of diabetic complications. The recent finding that preservation of C-peptide secretion has beneficial outcomes in type 1 diabetic patients adds to the ongoing dispute [53]. Clinical studies aimed in verifying the effect of the reintegration of physiologic levels of C-peptide on the development/regression of diabetic complications appear to be mandatory in order to clarify the issue.
6.2.4 Podocytes and Podocyturia
The progression of diabetic nephropathy is characterized by a gradual decrease of the number of podocytes (the arborized, postmitotic, and terminally differentiated cells in charge of the selective permeability of glomerular filtration barrier) residing inside the renal glomerulus [54–56]. This phenomenon is paralleled by an increasing loss of these same cells with urine (so-called podocyturia) [57, 58].
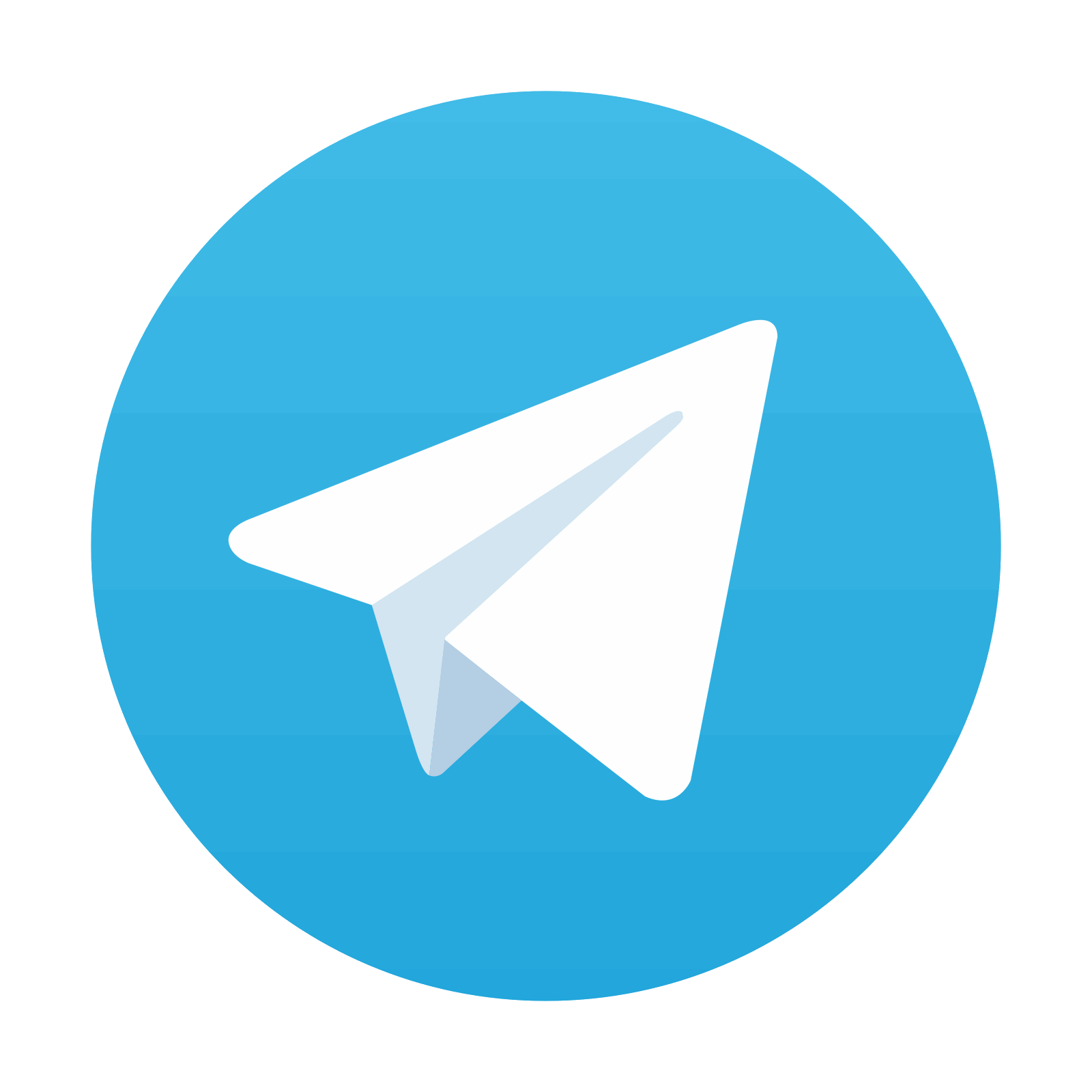
Stay updated, free articles. Join our Telegram channel

Full access? Get Clinical Tree
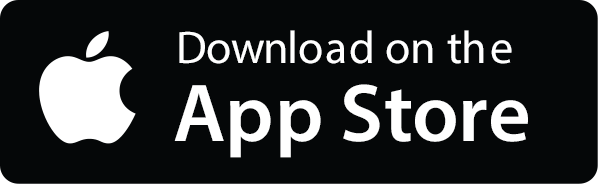
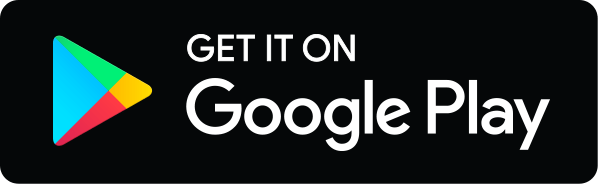