Nongenetic Factors in the Causation of Breast Cancer
Walter C. Willett
Rulla Tamimi
Susan E. Hankinson
Aditi Hazra
A. Heather Eliassen
Graham A. Colditz
Breast cancer has an enormous impact on the health of women. Approximately 227,000 women are diagnosed with invasive breast cancer annually in the United States, accounting for approximately 29% of all incident cancers among women (1). Each year, 40,000 women die of breast cancer, making it the second leading cause of cancer deaths among U.S. women, after lung cancer, and the leading cause of death among women aged 40 to 55 years. Breast cancer is rare among men, with only 2,190 incident cases and 410 deaths estimated for the United States in 2012 (1). The lifetime risk of being diagnosed with breast cancer through age 85 years for an American woman is approximately 1 in 8, or 12.15%, whereas the lifetime risk of dying from breast cancer is approximately 3.4% (2).
This chapter begins with a description of the marked variations in breast cancer rates among populations and over time. Decades of research have led to a substantial understanding of the factors involved in the development of breast cancer; known and suspected risk factors are reviewed and considered in relation to etiologic mechanisms leading to breast cancer. The contribution that known risk factors make to the existing variations in rates is considered; this contribution is central to the question of whether unidentified pollutants or dietary factors explain the current high rates in the United States. Because of the major investments in breast cancer research, the means for preventing a substantial fraction of breast cancer now exists; strategies that can be adopted by individual women, their healthcare providers, and societies and governments as a whole are examined.
DESCRIPTIVE EPIDEMIOLOGY OF BREAST CANCER
High- and Low-Risk Populations
The incidence of female breast cancer varies markedly between countries, being highest in the United States, western and northern Europe, intermediate in southern and eastern Europe and South America, and lowest in Asia and Africa (3). In 2008, the age-adjusted incidence rate of breast cancer varied by about a factor of five across countries worldwide (Fig. 18-1) (3). However, incidence rates have been rising in traditionally low-incidence Asian countries, particularly in Japan, Singapore, and urban areas of China as these regions are making the transition toward a Western style of economy and pattern of reproductive behavior (4, 5). As a result of unfavorable trends in these countries, the international gap in breast cancer incidence has narrowed since 1970 (6).
Age-Incidence Curve of Breast Cancer Risk
Breast cancer is extremely rare among women younger than 20 years and is uncommon among women younger than 30 years. Incidence rates increase sharply with advancing age, however, and become substantial before age 50 years. From 2000 to 2009, the incidence of breast cancer among American women aged 30 to 34 years was 26 per 100,000 and increased to 188 per 100,000 among women aged 45 to 49 years (1). The rate of increase in breast cancer incidence continues throughout life but slows somewhat around ages 45 to 50 years, strongly suggesting the involvement of reproductive hormones in breast cancer etiology because non-hormone-dependent cancers do not exhibit this change in slope of the incidence rate curve around the time of menopause (7). By age 70 to 74 years, the incidence of breast cancer among American women rises to 425 per 100,000 (8). The shape of the age-incidence curve in low- and intermediate-risk populations is similar to that of the United States, although the absolute rates are lower at each age (9) (Fig. 18-2).
Racial/Ethnic Groups within the United States and Studies of Migrants
According to recent data from the Surveillance, Epidemiology, and End Results (SEER) registries (1), the lifetime risk of breast cancer for white women in the United States is 12.8%, approximately 1 in 8, whereas that for black women is 10.1%, approximately 1 in 10. Between 2000 and 2009, the overall age-adjusted incidence rate of breast cancer among white women in the United States averaged 127 per 100,000 women, whereas the corresponding rate among black women averaged 121 per 100,000 women (1). However, these age-adjusted figures conceal a crossover pattern in which the risk of breast cancer at a young age is modestly higher among black women than white women. At older ages, incidence rates for white women are substantially higher than those among black women (Fig. 18-2).
Unlike that of most other illnesses, the lifetime risk of breast cancer is positively associated with higher socioeconomic status. This association is largely explained by the known reproductive risk factors (10); women in lower socioeconomic strata are more likely to have more children and to have them at younger ages than women in higher socioeconomic strata. It is likely that much, if not all, of black/white differences in breast cancer rates among older women reflect racial differences in social class distribution (11) and, thus, in the distribution of established reproductive risk factors. The modestly higher incidence rates of breast cancer among young black women relative to young white women are consistent with the hypothesis of a short-term increase in breast cancer risk immediately following pregnancy, although the overall lower lifetime risk of breast cancer among black women is consistent with the hypothesis of a long-term benefit of early and repeated pregnancy (12). The effect of these reproductive factors on breast cancer risk is described in greater detail in the following section on modeling. Although black women have a lower probability of developing breast cancer over their lifetime, their risk of dying from breast cancer is the same or perhaps even slightly higher than white women (3.26% compared to 2.86% for black and white women, respectively) (13). Black women have poorer 5-year survival rates from breast cancer at all ages of diagnosis compared to white women (2). This poorer survival can be attributed, in part, to the tendency of black women to be diagnosed at later stages of disease (2). In addition, there is evidence that molecularly defined subtypes of breast cancer associated with poor prognosis, specifically basal-like tumors, are more likely to occur among black women (2).
Breast cancer incidence rates among Asian, Hispanic, and American Indian women in the United States are considerably lower than those of (non-Hispanic) white women (2). The magnitude of the difference in incidence rates among various ethnic groups often depends on migrant status. For instance, breast cancer incidence for Chinese American and Japanese American women from 1973 to 1986 was about 50% lower for those born in Asia and about 25% lower for those born in the United States compared to U.S.-born white women (14). Among Filipino residents of the United States, the incidence rate of breast cancer was nearly identical between foreign-born and U.S.-born women, and both were less than half that of U.S.-born white women. Compared with Chinese women living in the mainland, Singapore, and Hong Kong, Asian-born Chinese women living in the United States had an almost twofold higher annual rate of breast cancer, and U.S.-born Chinese women had a higher rate still (14, 15). The pattern for Japanese women was similar (14).
These findings are consistent with a large body of literature showing increases in breast cancer incidence following migration from a low-risk country to the United States (16, 17, 18, 19, 20 and 21). Ziegler et al. (21) noted a six-fold gradient in risk of breast cancer among Asian women, depending on recency of migration. Asian American women with three or four grandparents born in the West were at highest risk, whereas women who were born in rural areas of Asia and whose length of residence in the United States was a decade or less were at lowest risk. Although the studies of breast cancer risk among migrants have focused almost exclusively on migrants from low-risk to high-risk countries and have shown convergence of rates, there are also data suggesting that a convergence of rates similarly occurs when migrants move from high-risk to low-risk countries. For instance, Kliewer and Smith (22), reporting on immigrants to Australia and Canada, note that immigrant groups coming from countries where breast cancer mortality rates were higher than those of native-born women often showed a decrease in mortality. Such findings strongly suggest that factors associated with the lifestyle or environment of the destination country influence breast cancer risk and are consistent with a positive relationship between length of time in the destination country and adoption of that country’s lifestyle. For example, among immigrants, the fertility rate and the average number of children born tend to converge to the rates of the destination country (23, 24).
Geographic Variation in the United States
Breast cancer incidence and mortality rates vary within the United States, although to a much smaller degree than among countries. During the 1980s, the incidence of breast cancer in the San Francisco Bay Area was somewhat higher than that for the rest of the United States, and international comparisons based on data from this decade led to an often-quoted statement that white women in the San Francisco Bay Area had the highest incidence of breast cancer in the world (15, 25). Based on the most recent SEER data (1) and the National Cancer Institute (Fig. 18-3A), incidence of breast cancer is above the national average among women in the Pacific Northwest and the northeastern United States (ageadjusted incidence rate for Connecticut is 137 per 100,000 and for Washington is 132 per 100,000) (1). Previous reports have concluded that the high incidence of breast cancer in the San Francisco area and in the Northeast can likely be accounted for by regional differences in the prevalence of known risk factors, including parity, age at first full-term pregnancy, age at menarche, and age at menopause (25, 26, 27, 28 and 29). Among the 17 SEER registry sites, the lowest age-adjusted incidence rates among women are found in Arkansas (109 per 100,000), Utah (108 per 100,000), and New Mexico (110 per 100,000) (1, 9). Again, regional differences in reproductive risk factors largely explain these lower rates.
Geographic differences in breast cancer mortality across the United States vary by approximately 1.5 comparing areas of highest versus lowest mortality (1). Figure 18-3B illustrates these regional differences from 2005 to 2009. Although incidence rates are highest in the Pacific Northwest and Northeast, these regions tend to have lower breast cancer mortality rates than the national average. These differences may be due to regional differences in breast cancer risk factors, mammographic screening, and treatment. Geographic differences in the prevalence of established risk factors explain much of the geographic differences in mortality. In 1987, age-adjusted mortality ratios among women 50 years and older were 1.15, 1.18, and 1.30 in the West, Midwest, and Northeast, respectively, compared with the South. After adjustment for established breast cancer risk factors, these mortality ratios fell to 1.13, 1.08, and 1.13, respectively (29).
Trends in Incidence and Mortality
Incidence rates of breast cancer have steadily increased in the United States since the 1930s, when formal record keeping began in Connecticut, until 2000. Between 1950 and 2000, the age-adjusted incidence rate rose by an average of 1.4% per year in this state (30), which has the oldest cancer registry in continuous operation. This represents a cumulative increase of about 70% over the 50 years. During the 1980s, incidence rates rose more sharply. Data from the SEER program, which began collecting data from different registries across the country in 1973, confirm the trends in incidence portrayed in the Connecticut registry since that time (Fig. 18-4). Increases have occurred in all age groups since 1935, although the magnitude of increase has been greater for older women. Between 1975 and 2000, incidence rates among black women younger than 50 years increased by 22% compared to a cumulative increase of 10% for white women younger than 50 years (8). Among women 50 years and older, the cumulative increase was 40% for both black and white women. Between 2001 and 2004, incidence rates of breast cancer decreased by approximately 3.5% per year. Between 2005 and 2009, incidence rates among white women have remained relatively constant; while among black women incidence rates have increased.
Several studies have examined whether the increase in breast cancer incidence in the United States has been due to the increasing use of screening mammography (31, 32, 33, 34, 35 and 36). Because screening causes at most a transient increase in incidence, and because its use was limited before the 1980s, it can explain little of the long-term increase between the 1930s and the 1980s. However, during the 1980s, the
increased incidence was almost entirely due to an increase in localized disease and in tumors measuring less than 2 cm in diameter; the incidence of tumors 2 cm or larger remained stable. These findings suggest that the increase in use of screening mammography accounts for part of the recent increase in breast cancer incidence (34, 37). A recent analysis of SEER data suggests that 31% of breast cancers diagnosed in 2008 are due to overdiagnosis and in the absence of mammographic screening would have never been clinically detected (38). Although this study was limited by making a number of assumptions, including that the underlying incidence was constant over this time and was unable to distinguish between DCIS and regional disease, the results are in line with other studies suggesting that a substantial amount of breast cancers are overdiagnosed with mammographic screening (39). The continued increase in breast cancer rates during the 1990s may be due in part to increased use of hormone replacement therapy, obesity, and mammography screening. The decline in rates observed between 2001 and 2004 likely reflect decreases in both mammographic screening and postmenopausal hormone use after publication of results from the Women’s Health Initiative (WHI) randomized trial in 2002 (30, 40). Since 2005, breast cancer rates have plateaued in the United States, Canada, Australia, and parts of Europe (3).
increased incidence was almost entirely due to an increase in localized disease and in tumors measuring less than 2 cm in diameter; the incidence of tumors 2 cm or larger remained stable. These findings suggest that the increase in use of screening mammography accounts for part of the recent increase in breast cancer incidence (34, 37). A recent analysis of SEER data suggests that 31% of breast cancers diagnosed in 2008 are due to overdiagnosis and in the absence of mammographic screening would have never been clinically detected (38). Although this study was limited by making a number of assumptions, including that the underlying incidence was constant over this time and was unable to distinguish between DCIS and regional disease, the results are in line with other studies suggesting that a substantial amount of breast cancers are overdiagnosed with mammographic screening (39). The continued increase in breast cancer rates during the 1990s may be due in part to increased use of hormone replacement therapy, obesity, and mammography screening. The decline in rates observed between 2001 and 2004 likely reflect decreases in both mammographic screening and postmenopausal hormone use after publication of results from the Women’s Health Initiative (WHI) randomized trial in 2002 (30, 40). Since 2005, breast cancer rates have plateaued in the United States, Canada, Australia, and parts of Europe (3).
Trends in breast cancer mortality are of major public health interest, but their interpretation is complex because they reflect the combined effects of trends in underlying risk of breast cancer, changes in screening practices, and effectiveness of treatment. Also, the divergence between breast cancer incidence and mortality rates began in the United States even before the use of mammography or chemotherapy (Fig. 18-5), suggesting that awareness of breast cancer and earlier use of simple surgical treatment have also influenced mortality rates (41). Further, mortality rates lag behind changes in breast cancer incidence, screening, and treatment by at least 5 to 10 years (42). Age-adjusted mortality rates in the United States were relatively stable between the 1950s and the mid-1980s, when an overall decline was first noted (37). Mortality rates in the late 1980s began to decline slightly (about 1% per year). Rates through the 1990s declined somewhat more (3% decline per year) (8, 43), perhaps because of enhanced treatment and screening. These overall trends obscure important variation by age and race, however. Since the 1970s, mortality rates have fallen for younger white women, and this decline has accelerated since the late 1980s. From 1973 to 1995, the cumulative decline in mortality rates for white women younger than 60 years has been more than 20%, with much of this decline occurring since 1988. In contrast to these trends among younger white women, mortality rates for white women 60 years and older increased slowly during the 1970s and 1980s, although since the late 1980s, mortality has also begun to decline in this group (37, 42). The trends in breast cancer mortality among black women have been unfavorable; between the 1970s and 1990, mortality rates increased for black women in all age groups (42), and only recently is there evidence of a decline, but to a lesser extent than what is observed in white women (Fig. 18-6). From 1992 to 2009, breast cancer death rates have declined by 1.8% per year in whites compared with 1.0% in black women (1).
![]() FIGURE 18-4 Agestandardized incidence of breast cancer in the United States. (Data from Surveillance Epidemiology, and End Results Program, Cancer Incidence, and Mortality Rates.) |
Trends in Incidence and Mortality around the World
Since the 1950s, breast cancer incidence has been increasing in many of the lower risk countries and in high-risk Western countries. Some of the recent increases in incidence in high-risk populations may be due to greater use of mammography, as in the United States. This appears to be the case in Sweden (44) and in England and Wales (45). Breast cancer incidence rates have nearly doubled in recent decades in traditionally low-risk countries such as Japan (4, 9) and Singapore (5) and in the urban areas of China (46). Dramatic changes in lifestyle in such regions brought about by growing economies, increasing affluence, and increases in the proportion of women in the industrial workforce have had an impact on the population distribution of established breast cancer risk factors, including age at menarche and fertility, as well as nutritional status (47). These changes have resulted in a convergence toward the risk factor profile of Western countries (47).
Trends in breast cancer mortality around the world have largely paralleled the trends in incidence. Between the 1970s and 1990s mortality increased in both high-risk and lower risk populations, although since the 1990s mortality has declined moderately in the United Kingdom, the Netherlands, and Sweden, similar to the decline observed in the United States (3, 48, 49). As in the United States, some of the downturn in mortality in these countries may be due to more widespread use of screening mammography, adjuvant chemotherapy during the 1980s (48, 50), and more recently targeted therapies (51). Countries with mortality rates that are still increasing tend to be those with the lowest mortality (48). For instance, Russian Federation, Republic of Korea, and Japan all have breast cancer mortality rates that are continuing to increase (3). Thus, a convergence of breast cancer mortality rates may be occurring internationally, in part reflecting an international convergence of reproductive and behavioral risk factors (48).
REPRODUCTIVE FACTORS
This section addresses reproductive factors during the course of a woman’s life in relation to the risk of breast cancer. An underlying concept is that ovarian hormones initiate breast development and that subsequent monthly menstrual cycles induce regular breast cell proliferation. Puberty is a critical period during breast development. The onset of puberty is marked by a surge of hormones that induce regular breast cell proliferation. This pattern of cell division terminates with menopause, as indicated by cessation of ovulation and menstrual periods.
Menarche
Menarche represents the development of the mature hormonal environment for a young woman and the onset of monthly cycling of hormones that induce ovulation, menstruation, and cell proliferation within the breast and endometrium. Earlier age at menarche has been consistently associated with increased risk of breast cancer (52). Most studies suggest that age at menarche is related to both premenopausal and postmenopausal breast cancer, although the magnitude of effect appears to be greater for premenopausal than postmenopausal women (53). In a pooled analysis of 7,764 premenopausal women and 16,467 postmenopausal women, each additional year in delay of menarche was associated with a 9% decrease in premenopausal breast cancer and a 4% decrease in postmenopausal breast cancer (54). In addition, age at menarche is inversely associated with both estrogen receptor positive (ER+)/progesterone receptor positive (PR+) and estrogen receptor negative (ER-)/progesterone receptor negative (PR-) breast tumors, although the protective effect of late age at menarche is greater for hormone receptor positive tumors (55). More recently, studies have evaluated the association between reproductive factors and molecular subtypes of breast cancer. At least four major categories of invasive breast cancer have been reproducibly identified by gene expression profiling: luminal A, luminal B, HER2-type, and basal-like (56). Large-scale epidemiologic studies have used immunohistochemical markers as proxies to characterize tumors into these subtypes. These studies have tended to show an association with increasing age at menarche and reduced risk of luminal A tumors (57, 58).
Although menarche is most clearly related to the onset of ovulation, some studies suggest that hormone levels may be higher through the reproductive years among women who have early menarche (59). In addition, early menarche may be associated with earlier onset of regular ovulatory menstrual cycles and hence greater lifetime exposure to endogenous hormones (60). Whether the levels of ovarian hormones or their cyclic characteristics are the underlying influence on breast cancer risk is unsettled (7); both likely play a role.
Menstrual Cycle Characteristics
Shorter cycle length has been quite consistently related to greater risk of breast cancer (52), although not all studies support this relation (61). Shorter cycle length during ages 20 to 39 years may be associated with higher risk of breast cancer, perhaps because the shorter cycle length is associated with a greater number of cycles and more time spent in the luteal phase when both estrogen and progesterone levels are high. Long and irregular cycles may also be related to reduced risk of breast cancer (61).
Ovulatory infertility, an indicator of infertility due to hormonal causes, has not been consistently related to risk of breast cancer, although one cohort study suggested a substantially lower risk among women with this condition (relative risk [RR] = 0.4 compared to women with no infertility history) (61). The significant inverse association seen in this study may be related to the young age of the cohort and thoroughness of investigation of the cause of infertility in this group of health professionals.
Pregnancy and Age at First Full-term Pregnancy
Nulliparous women are at increased risk of breast cancer compared to parous women. This risk is evident after age 40 to 45 years, but not for breast cancer diagnosed at younger ages. In the majority of epidemiologic studies, a younger age at first full-term pregnancy predicts a lower lifetime risk of breast cancer (52). The reduction in risk following pregnancy compared to nulliparous women is not immediate but takes approximately 10 to 15 years to manifest (62). In fact, risk of breast cancer is increased for the first decade following first pregnancy (12, 63, 64). The proliferation of breast cells during the first pregnancy results in differentiation into mature breast cells prepared for lactation; this may also lead to growth of mutated cells and excess risk over the next decade. Epidemiologic evidence for the transient excess risk after the first pregnancy is consistent. Less clear is the presence of a transient increase in risk after subsequent pregnancies; some studies suggest an adverse effect (65) but others do not (64).
The first pregnancy is associated with permanent changes in the glandular epithelium and with changes in the biologic properties of the mammary cells. After the differentiation of pregnancy, epithelial cells have a longer cell cycle and spend more time in the G1 phase, the phase that allows for DNA repair (66). The longer the interval from menarche to first pregnancy, the greater the adverse effect of the first pregnancy (12). The later the age at first full-term pregnancy, the more likely that DNA mistakes have occurred that will be propagated with the proliferation of mammary cells during pregnancy. The susceptibility of mammary tissue to carcinogens decreases after the first pregnancy, reflecting the differentiation of the mammary gland. This is also seen in the age-dependent susceptibility of the breast to radiation, reviewed later in this chapter.
Number and Spacing of Births
A higher number of births is consistently related to lower risk of breast cancer; each additional birth beyond the first reduces long-term risk of breast cancer. Although in some analyses, this has not been independent of earlier age at first birth, the overall evidence indicates an independent effect of greater parity (67). In addition to a protective effect of higher parity, several studies now indicate that more closely spaced births are associated with lower lifetime risk of breast cancer (64, 68). This may be due to the breast having less time to accumulate DNA damage before it attains maximal differentiation by repeated pregnancies.
Lactation
As early as 1926, it was proposed that a breast never used for lactation is more liable to become cancerous (69). There are two major biologic mechanisms proposed to induce the protective effect: Breast-feeding may result in further terminal differentiation of the breast epithelium, and lactation delays the resuming of ovulatory menstrual cycles after pregnancy. Ecological studies demonstrate a consistency with the patterns of international variation in breast cancer incidence: Rates are lower in populations where breast-feeding is both
common and of long duration. The overall evidence from case-control and cohort studies supports a reduction in risk with longer duration of breast-feeding, but the findings have varied substantially in the level of risk reduction. Some of the differences may relate to the pattern of breast-feeding, for example, whether feeding was exclusively from the breast or supplemented with other food; this needs to be evaluated further. A pooled analysis from almost 50 studies in 30 countries reported an overall 4% reduction in risk per 12 months of breast-feeding for all parous women (70). The authors estimate that if women in developed countries had the number of births and lifetime duration of breast-feeding of women in developing countries, cumulative incidence of breast cancer by age 70 years would be reduced by as much as 60%. About two-thirds of this reduction would be related to breast-feeding (70).
common and of long duration. The overall evidence from case-control and cohort studies supports a reduction in risk with longer duration of breast-feeding, but the findings have varied substantially in the level of risk reduction. Some of the differences may relate to the pattern of breast-feeding, for example, whether feeding was exclusively from the breast or supplemented with other food; this needs to be evaluated further. A pooled analysis from almost 50 studies in 30 countries reported an overall 4% reduction in risk per 12 months of breast-feeding for all parous women (70). The authors estimate that if women in developed countries had the number of births and lifetime duration of breast-feeding of women in developing countries, cumulative incidence of breast cancer by age 70 years would be reduced by as much as 60%. About two-thirds of this reduction would be related to breast-feeding (70).
Recent studies have examined the association between lactation in relation to breast cancer subtype. In the Black Women’s Health Study, ever breast-feeding was associated with a nonsignificant reduced risk of ER-/PR- breast cancer (Hazard ratio [HR]=0.78, 95% confidence Interval [CI], 0.60-1.03), but not ER+/PR+ breast cancer (HR=1.13; 95% CI, 0.91-1.42) (71). This study also found that higher parity was associated with an increased risk of ER-/PR-breast cancer and that breast-feeding appeared to ameliorate the increased risk. In addition, three recent studies have reported inverse associations between breast-feeding and reduced risk of basal-like breast cancers defined using immunohistochemical markers ranging from 20%-40% reduction comparing 4+ months of breast-feeding to never breast-feeding (57, 72, 73). In the Nurses’ Health Study, 4+ months of breast-feeding relative to never breast-feeding was associated with a 40% reduced risk of basal-like tumors (RR = 0.6; 95% CI, 0.4-0.9) and a 20% reduced risk of luminal A tumors (RR = 0.8; 95% CI, 0.7-1.0) (57). Given that there are only a few studies that have assessed this relation and all three studies had less than 300 basal-like cases, additional studies are needed to better understand the association between lactation and tumor subtypes.
Spontaneous and Induced Abortion
Close to one-fourth of all clinically identified pregnancies in the United States end as induced abortions (74), and for women whose pregnancies continue for 8 to 28 weeks, the probability of spontaneous abortion ranges from 8% to 12% (75). It has been suggested that breast cells are the most vulnerable to mutation when breast tissue consists of rapidly growing and undifferentiated cells, such as during adolescence and pregnancy. In early pregnancy, the number of undifferentiated cells increases as rapid growth of the breast epithelium is taking place. If the pregnancy continues to term, these cells differentiate by the third trimester, thus, the number of cells susceptible to malignancy decreases. The interruption of the differentiation of breast cells that takes place as the result of spontaneous and induced abortions has been hypothesized to increase a woman’s risk of developing breast cancer (76). This hypothesis appears to be supported by a meta-analysis that included data from 28 published reports on induced abortion and breast cancer incidence (77). However, this analysis, based largely on case-control studies, contains the underlying serious potential for bias in retrospective studies of the relation between abortion and breast cancer. Induced abortion can be an extremely sensitive topic, and reporting on abortion history by women with a life-threatening condition such as breast cancer may be more complete than reporting by women without breast cancer.
By far the largest study on the association between breast cancer and abortion was a population-based cohort study made up of 1.5 million Danish women born April 1, 1935, through March 31, 1978 (78). Of these women, 280,965 (18.4%) had one or more induced abortions. After adjusting for potential confounders of age, parity, age at delivery of first child, and calendar period, the risk of breast cancer for women with a history of induced abortion was not different from that of women who had not had an induced abortion (RR = 1.0; 95% CI, 0.94-1.06). In addition, there was no trend in risk with increasing number of induced abortions in a woman’s history. Similarly, no association between induced abortion and breast cancer incidence was observed in four prospective cohort studies including the Iowa Women’s Study (79), the Shanghai Textile Workers Study (80), the European Prospective Investigation into Cancer and Nutrition (81), and the Nurses’ Health Study II (82). Taken as a whole and accounting for the limitations of the case-control study design, the available evidence does not support any important relation between induced abortion and risk of breast cancer. In 2003, the Early Reproductive Events and Breast Cancer Workshop, convened by the National Cancer Institute to assess the state of evidence between reproductive factors and breast cancer, recognized that spontaneous and induced abortions are not associated with breast cancer risk (83).
Age at Menopause
Early studies of age at menopause and risk of breast cancer focused on women who had undergone bilateral oophorectomy at a young age; these women have a greatly reduced risk of breast cancer (84, 85). Women with bilateral oophorectomy before age 45 years have approximately half the risk of breast cancer compared to those with a natural menopause at 55 years or older. On average, the risk of breast cancer increases by some 3% per year of delay in age at menopause. Although some studies suggest the effect of age at menopause decreases with advancing age at breast cancer diagnosis (86), this may reflect greater error in recall of age at menopause as women are further removed from the event (87). Adjustment for error in recall removes this apparent decrease in the effect of menopause with advancing age.
The reduction in risk of breast cancer with early menopause is likely due to the reduction of breast cell division with the termination of menstrual cycles and the decline in endogenous hormone levels, which become substantially lower than during the premenopausal years.
Models of Reproductive Factors and Breast Cancer Incidence
Biomathematical models are derived by translating a series of hypotheses about the biologic process involved in carcinogenesis into mathematical terms. The classical models of carcinogenesis proposed by Armitage and Doll (88) and by Moolgavkar and Knudson (89) are the best known. Armitage and Doll noted that the gradient of 6 to 1 (i.e., 6 units increase in the logarithm of death rate per unit increase in logarithm of age) was more or less consistent across 17 cancer sites, but also noted a deficit in mortality from breast cancer among older women. They attributed this to a reduction during middle life in the rate of production of one of the later changes in the process of carcinogenesis (88). Pike et al. (63) reviewed the epidemiologic evidence in the early 1980s and proposed a model of tissue aging that accounted for the relation between reproductive risk factors and breast cancer incidence. Ultimately, models will ideally be developed that take into account all known risk factors.
The mathematical model proposed by Pike et al. (63) was based on the observed age-incidence curve and on the known relations of ages at menarche, first birth, and menopause to the risk of breast cancer. The model proposed by Pike et al. (63) is built on earlier work by Moolgavkar et al., who fitted mathematical parameters to breast cancer incidence data from several countries. The Pike et al. model related breast cancer rates to the growth of the breast. The model allowed a short-term increase in risk with first pregnancy followed by a subsequent decrease in risk accumulation. Finally, at menopause the breast begins an involutional process that is thought to reflect a decrease in cell turnover and eventual disappearance of epithelium. The original Pike et al. model, however, did not include terms for the second or subsequent births or for the spacing of pregnancies, nor did it easily accommodate pregnancies after age 40 years. Type of menopause was not considered either (bilateral oophorectomy vs. natural menopause). Although there has been controversy about whether the bearing of additional children beyond the first reduces the risk of breast cancer, substantial evidence reviewed earlier indicates that both the number of births and their spacing are associated with risk: The greater the number of births and the closer they are spaced, the lower a woman’s risk of breast cancer.
An extension of the Pike et al. model of breast cancer incidence utilized prospective data from the Nurses’ Health Study (12, 64, 90) and added a term to summarize the spacing of births. Nonlinear models produced parameters that were difficult to interpret (64), but a subsequent modification allowed ready estimation of RRs (12), thus making the results more accessible to epidemiologists and clinicians familiar with the RR as measure of the relation between an exposure and disease. Prior to menopause, the incidence of breast cancer increased 1.7% for a 1-year increase in age at first birth. Closer spacing of births was related to significantly reduced risk of breast cancer. For each additional year of delay between the first and second births, for example, the risk of breast cancer increased by 0.4%. The increase in risk with first pregnancy originally observed with this modified Pike model has since been documented in a prospective study from Sweden (65) and in an analysis from an international case-control study (91). The effect of age at first and subsequent births on breast cancer incidence was still greater after menopause (Fig. 18-7).
According to the extended Pike et al. model, a parous woman with a single birth at age 35 years has a 34% increase in breast cancer incidence at the time of the birth relative to a nulliparous woman. The excess risk goes down very slowly over time. Even at age 70 years such a woman has excess risk versus a nulliparous woman. In sum, the cumulative risk to age 70 is 16% greater than that of a nulliparous woman. Conversely, a parous woman with an early age at first birth (20 years of age) and multiple births conceived at a young age has a slight excess risk immediately after the first birth relative to the nulliparous woman (RR = 1.10), which slowly diminishes over time, reaching equality at age 32 years and continuing to decline until menopause (age 50 years), at which time the RR is 0.82. Since the relationship between breast cancer incidence and reproductive history changes with age, cumulative incidence, rather than age-specific incidence, is a useful summary (see Fig. 18-7). Compared to a nulliparous woman, a woman with one birth at age 35 years has a 16% excess risk over the age period 30-70 years, while the woman with births at ages 20, 23, and 26 years has a 27% decrease in risk over the similar age period (90).
In the original Pike et al. model (63), factors associated with reduced risk of breast cancer were each considered to slow the rate of breast tissue aging, which correlates with the accumulation of molecular damage in the pathway to breast cancer. In the Rosner and Colditz (12, 90) extension of the Pike et al. model, the rate of tissue aging was highest between menarche and first birth, consistent with the hypothesis that this is the period when the breast is most vulnerable to mutagenesis. The transient increase in the risk of breast cancer associated with the first pregnancy is followed by a 20% decrease in the rate of breast tissue aging (12). This observation helps explain the cross-over effect in certain subgroups of women: Around menopause, rates of one subgroup that were initially higher drop below rates of a second subgroup. For instance, using data from New York State, Janerich and Hoff showed a cross-over in breast cancer incidence between single and married women at age 42, such that married women had a higher incidence before this age and lower mortality thereafter (92). A similar cross-over of incidence has been reported for black and white women in the United States (11, 93), consistent with the distribution of age at first birth by race. Over many decades, pregnancy rates have been higher and age at first birth has been younger for black women than for white women (94).
The age-incidence curve from biomathematical models of reproductive events and breast cancer incidence also mirrors the observed patterns of breast cancer incidence across many countries. In China and many developing countries, the estimated number of births in the early 1960s was 6.5 births per woman (95), which is not associated with a late age at first birth. Also, the average age at menarche in China was about 17 years, even through the 1960s (96). Fitting the Rosner and Colditz model with menarche at age 16 years, first birth at age 19 years, six births spaced a year apart, and age at menopause 50 years, we estimate an annual rate of breast cancer incidence for 65-year-old Chinese women is 93.6 per 100,000. For the cohort of U.S. women born between 1921 and 1925, the average age at menarche was approximately 13.5 years, the median age at first birth was 23 years, the mean number of children was three, and the mean interval between births was 3 years (97). Considering these characteristics, and holding age at menopause constant at 50 years, the annual rate of breast cancer incidence predicted for 65-year-old U.S. women is 279 per 100,000—close to the observed SEER rate of 300 per 100,000 for women of
this age, and approximately three times the rate for Chinese women. Applying this model to typical reproductive patterns for women from low-incidence countries suggests that reproductive factors alone account for more than half of the international variation in the risk of breast cancer (98). These results were confirmed when the model was applied to data from a Chinese cohort (99).
this age, and approximately three times the rate for Chinese women. Applying this model to typical reproductive patterns for women from low-incidence countries suggests that reproductive factors alone account for more than half of the international variation in the risk of breast cancer (98). These results were confirmed when the model was applied to data from a Chinese cohort (99).
The extension of the Rosner and Colditz model to include history of benign breast disease, height, weight, alcohol intake, and type of postmenopausal hormone used, in addition to reproductive factors and family history, gives a model that compares favorably to the Gail model for risk prediction (100). In a meta-analysis of breast risk prediction models that have been validated, the Gail model and the Rosner model have equivalent performance with area under the curve or c-statistic values of 0.63 (101). Furthermore, this extended Rosner model has been applied to the evaluation of risk factors for ER+ and ER- breast cancer. Incidence of ER+/PR+ tumors increases at 11.0% per year during premenopausal years and at 4.6% per year after natural menopause. In contrast, the incidence of ER-/PR- tumors increases at 5.0% per year during premenopausal years and 1.3% after natural menopause. The one-time adverse effect of first pregnancy is present for ER-/PR- breast cancer but not ER+/PR+ tumors. Parity shows a strong inverse association with ER+/PR+ tumors (RR = 0.6 for four births at 20, 23, 26, and 29 versus nulliparous), but not ER-/PR- tumors (RR = 1.1 for four births vs. nulliparous). Other risk factors, including benign breast disease, family history of breast cancer, alcohol use, and height, show consistent relations with both ER+/PR+ and ER-/PR- breast cancer, while body mass index after menopause is related to incidence of PR+ but not PR- tumors. The concordance statistic (indicating predictive ability of the model) adjusted for age was 0.64 (95% CI, 0.63-0.66) for ER+/PR+ tumors, and for ER-/PR- the concordance statistic was 0.61 (95% CI, 0.58-0.64) (102). Addition of circulating estrogen levels to the model adds further to the performance (103) as does refining benign breast disease (BBD) to consider atypical hyperplasia and proliferative disease without atypia (104).
Risk Prediction
Breast cancer incidence models have been applied to predict the risk of breast cancer over a defined time period, say 5 or 10 years. The larger the number of risk factors considered, the higher the likelihood the prediction model will separate those at risk of disease from those who are not as likely to develop disease. However, as Wald notes (105), to be useful as a screening test or an individual marker of risk or to identify those who will develop disease and those who will not, the magnitude of association for a predictor must be on the order of 10 or higher comparing extreme quintiles for a detection rate of 20%. No prediction models for breast cancer have achieved this level of discrimination to date. The Rosner model generates a relative risk of 6 or more comparing top versus bottom decile of risk among women in each 5-year age group.
Ottman et al. published a simple model in 1983 that calculates a probability of breast cancer diagnosis for mothers and sisters of breast cancer patients (106). They used life-table analysis to estimate the cumulative risks to various ages based upon two groups of patients from the Los Angeles County Cancer Surveillance Program, then derived a probability within each decade between ages 20 and 70 for mothers and sisters of the patients, according to the age of diagnosis of the patient and whether the disease was bilateral or unilateral.
Because risk factors may change over the life course (weight gain, change in alcohol intake, menopausal status, use of postmenopausal hormones for some years, etc.), it becomes more helpful to consider the impact of all these risk factors on breast cancer cumulative risk up to a given age, say 70 or 75. This approach has been developed for breast cancer risk according to family history (107), and the prediction of BRCA1 carrier status (108, 109), but more general applications joining carrier status and lifestyle factors remain limited (110).
The complex nature of breast cancer incidence, with many possible time-dependent risk factors, requires prediction models that account for this variation over time. These are now shown to outperform traditional approaches that fit indicator variables with fixed effects across time (100). In addition, the log-incidence model of Rosner and Colditz performs significantly better than the commonly used Gail model for total breast cancer incidence that includes only five variables (age, age at menarche, age at first birth, number of benign breast biopsies, and family history). Growing emphasis is placed on mammographic breast density as a contributor to risk prediction (111, 112), and while some models have incorporated this measure, none yet also include the details of reproductive risk factors, specific type of postmenopausal hormone therapy used, and breast cancer incidence.
The efficacy of chemoprevention for breast cancer is clearly shown for ER+ disease reducing risk by 50% (113). Given the need to balance risks and benefits when implementing a tamoxifen-based chemoprevention strategy (114), a model that successfully identifies women at increased risk of ER+ breast cancer will, therefore, improve the risk benefit ratio. Rosner and Colditz have applied their logincidence model to breast cancers classified according to receptor status and reported that the area under the ROC curve adjusted for age was 0.630 (95% CI, 0.616-0.644) for ER+/PR+ tumors and was 0.601 (95% CI, 0.575-0.626) for ER-/PR- tumors, indicating adequate discriminatory accuracy. On the other hand, when we fit the Gail model to the same data set, it had performance characteristics that were somewhat lower than the Rosner and Colditz model with values of 0.578 for total cancer and 0.57 for ER+/PR+ tumors. The difference between the area under the ROC for the Rosner and Colditz model versus the Gail model for total breast cancer was statistically significant (p < .0001), indicating that the more complete modeling of risk factors across the life course could be more useful for discriminating among those women at high and low risk for breast cancer.
The clinical application of risk prediction models with performance evaluation showing improved patient satisfaction with decision-making, improved health outcomes, or cost-effectiveness of care remains the gold standard for evidence of clinical utility (115). To date, none of the breast risk prediction models have been evaluated in this routine use setting to show such benefits for women.
ENDOGENOUS SEX HORMONES AND RISK OF BREAST CANCER
Several lines of evidence have long suggested that sex hormones play a central role in the etiology of breast cancer. As noted earlier, rates of breast cancer increase rapidly in the premenopausal years, but the rate of increase slows sharply at the time of menopause, when estrogen levels decline rapidly. In addition, several reproductive variables that alter hormone status affect risk of breast cancer; for example, early age at menarche and late age at menopause
are associated with increased risk of breast cancer while parity is inversely associated with risk. After menopause, adipose tissue is the major source of estrogen, and obese postmenopausal women have both higher levels of endogenous estrogen and a higher risk of breast cancer (116). In animals, estrogens, progesterone, and prolactin all promote mammary tumors. Also, hormonal manipulations such as anti-estrogens (e.g., tamoxifen) are useful in the treatment of breast cancer and reduce breast cancer incidence in highrisk women (117, 118 and 119).
are associated with increased risk of breast cancer while parity is inversely associated with risk. After menopause, adipose tissue is the major source of estrogen, and obese postmenopausal women have both higher levels of endogenous estrogen and a higher risk of breast cancer (116). In animals, estrogens, progesterone, and prolactin all promote mammary tumors. Also, hormonal manipulations such as anti-estrogens (e.g., tamoxifen) are useful in the treatment of breast cancer and reduce breast cancer incidence in highrisk women (117, 118 and 119).
Methodologic Issues in Studies of Endogenous Hormones and Breast Cancer Risk
In contrast to clinical needs where discerning grossly abnormal from normal hormone levels is the focus, epidemiologic studies are usually aimed at detecting modest differences within the normal range of levels. Considerable laboratory error has been reported in studies of assay reproducibility, with several hormones being measured quite poorly by some laboratories (120, 121). Low reproducibility could result in true (and important) exposure/disease associations being missed. Varying sensitivities and specificities of different laboratory assays also have made comparison of results between studies difficult (122, 123). For example, in studies of postmenopausal plasma estradiol, mean levels in control subjects have ranged from 9 (124) to 28 (125) pg/ml. Although these differences may result in part from differences in characteristics of study subjects (i.e., differences in adiposity), a substantial component is likely due to the use of varying laboratory methods. Increasing efforts by the CDC and several professional organizations are addressing these measurement issues (e.g., testosterone) (126).
Several hormones, particularly estrogens, fluctuate markedly over the menstrual cycle. In some early studies, hormone levels were measured in samples collected without regard to the menstrual cycle phase, thus adding considerable “noise” to the comparison of hormone levels between breast cancer cases and controls. This noise could mask true associations or, because of chance differences in the distribution of cycle phase between cases and controls, could result in associations that do not truly exist. More recent studies have tended to collect all samples at approximately the same time in the cycle, have matched on cycle day, or have carefully controlled for cycle day in the analysis—all appropriate strategies.
For both logistic and financial reasons, in most epidemiologic studies only a single blood sample can be collected per study subject. Whether a single sample can reflect longterm hormone levels (generally the exposure of greatest etiologic interest) is therefore an important issue. In several studies, repeated blood samples were collected over a 1- to 3-year period in postmenopausal women and the correlation between the samples calculated. Overall, steroid hormones were reasonably stable, with intra-class correlations ranging from 0.5 to 0.9 (127, 128, 129 and 130). This level of reproducibility is similar to that found for other biologic variables such as blood pressure and serum cholesterol measurements, all parameters that are considered reasonably measured and that are consistent predictors of disease in epidemiologic studies. Data on premenopausal women are more limited, although follicular or luteal estrogens were reasonably reproducible over a 3-year period (131), and androgens have been reasonably correlated over a several-year period (128, 129, 131). Data on circulating levels of insulin-like growth factors also indicates substantial stability over a several-year period (131, 132).
Over the last decade a number of well-conducted prospective studies have assessed the role of circulating hormone levels and breast cancer risk; their findings are summarized below.
Estrogens
Estradiol, considered the most biologically active endogenous estrogen, circulates in blood either unbound (“free”) or bound to sex hormone-binding globulin or albumin. Free or bioavailable (free plus albumin-bound) estradiol is thought to be readily available to breast tissue and thus may be more strongly related to risk than total estradiol. Postmenopausally, estrone is the source of most circulating estradiol, and estrone sulfate is the most abundant circulating estrogen (133). Both normal and malignant breast cells have sulfatase and 17-beta-dehydrogenase activity (134). Thus, estrone and estrone sulfate could serve as ready sources of intracellular estradiol.
In 2002, a pooled analysis was published consisting of all prospective studies of endogenous estrogens and androgens in postmenopausal women that had been available at that time (135). Data were from nine prospective studies with a total of 663 breast cancer cases and 1,765 healthy controls; none of the women were using exogenous hormones at blood collection. The risk of breast cancer increased with increasing estrogen levels. For example, the relative risks (95% CI) for increasing quintiles of estradiol level, all relative to the lowest quintile, were 1.4 (1.0-2.0), 1.2 (0.9-1.7), 1.8 (1.3-2.4), and 2.0 (1.5-2.7). Estrone, estrone sulfate, and free estradiol were similarly related to risk. No significant heterogeneity in results was noted between the studies. Subsequent to the pooled analysis, several additional prospective studies have been published and all have supported these findings (136, 137 and 138). Further, urinary hormone levels have been assessed in relation to breast cancer in two prospective studies (139, 140) and in each, positive associations were observed.
The association between circulating estrogens and breast cancer risk appears stronger for ER+ and PR+ tumors, with relative risks ranging from 2.0-2.6 comparing the extreme 20-25% of levels (141, 142 and 143). Data are sparse and less consistent for ER- tumors. In the only two studies with more than 100 ER- cases, the association of estradiol with ER-/PR-tumors was similar to that observed for ER+/PR+ tumors in one (143) and considerably weaker than that observed in ER+ tumors in the second (142). The association with ER+ tumors is in line with findings from the tamoxifen and raloxifene trials, where risk of only ER+ tumors was reduced (118, 144) and also from epidemiologic studies of obesity and breast cancer where stronger associations have been noted for ER+ tumors.
Whether the association between plasma estrogens and postmenopausal breast cancer is similar in women at varying levels of breast cancer risk has been addressed in two studies. The first was conducted in the high-risk population of the National Surgical Adjuvant Breast and Bowel Project Cancer Prevention Trial (P-1) with 89 cases and 141 non-cases enrolled in the placebo arm of the trial (145). In P-1, high risk was defined as having at least a 1.66% 5-year risk of breast cancer as estimated from the Gail model (146). No association was observed between estradiol levels and breast cancer risk: The relative risk for the top (vs. bottom) quartile of levels was 0.96 (95% CI, 0.47-1.95). In contrast, in the Nurses’ Health Study cohort (with over 400 cases and 800 controls) (147), women were classified as high or low risk in several ways: according to family history of breast cancer, by their 5-year modified Gail risk score, and by their 5-year Rosner and Colditz risk score (90). Overall, the associations of plasma estrogens with breast cancer were robust across risk
categories regardless of which metric was used to define risk. Thus, the data from this larger cohort suggest that circulating estrogens are predictive of risk in women at low and at high risk of breast cancer; however, confirmation in other studies is needed.
categories regardless of which metric was used to define risk. Thus, the data from this larger cohort suggest that circulating estrogens are predictive of risk in women at low and at high risk of breast cancer; however, confirmation in other studies is needed.
Two prospective studies have addressed whether circulating estradiol levels are associated with breast cancer risk even in women using postmenopausal hormones (estrogen only or estrogen plus a progestin) (148, 149). In the first and largest study, modest positive associations with estradiol and free estradiol were observed (top versus bottom quartile RR for estradiol = 1.3; 95% CI, 0.9-2.0, p-trend = 0.20 and RR for free estradiol = 1.7; 95% CI, 1.1-2.7, p-trend = 0.06) (148). In the second study, similar associations were observed between circulating estrogens and breast cancer, regardless of postmenopausal hormone use (149). Thus, although women using postmenopausal hormones have a different hormonal profile than non-users, plasma estradiol concentrations appear to be associated, albeit possibly more modestly, with breast cancer in this group of women.
Data on premenopausal estrogen levels and breast cancer risk are more limited, in large part because of the complexities related to sampling during the menstrual cycle. Eight prospective studies have been published to date, although 6 of the 8 had fewer than 100 cases (range 14-98 cases) and, not surprisingly, no significant associations with plasma estrogens were observed in the 6 small studies (150, 151, 152, 153, 154 and 155). Two much larger studies have recently been published. In the first, conducted in the European Prospective Investigation into Cancer and Nutrition (EPIC) cohort, with 285 breast cancer cases and 555 controls, one blood sample was collected per woman, and the day in the menstrual cycle was recorded (156). Controls were matched to cases on age and phase of the menstrual cycle at blood collection (defined in 5 categories). Comparisons between case and control hormone levels were based on residuals from spline regression models; the residuals indicated how much an individual’s hormone level deviated from the predicted hormone levels on that day. Overall, no association was observed for either estradiol or estrone (e.g., top to bottom quartile comparison [RR = 1.0; 95% CI, 0.7-1.5] for estradiol). In the second large prospective study, conducted within the NHSII, both early follicular and mid-luteal samples were collected from each woman (157). The analysis included 197 cases with 394 controls also matched on age and luteal day. Follicular, but not luteal, total and free estradiol were significantly associated with breast cancer risk (top to bottom quartile comparison [RR = 2.1; 95% CI, 1.1-4.1] for follicular total estradiol). No association was observed with either estrone or estrone sulfate (in either phase of the cycle). Clearly, additional data, with careful matching of cases and controls and detailed evaluation by timing in the menstrual cycle, are needed.
To date, only one study has examined the role of circulating estrogen levels during pregnancy and subsequent breast cancer risk (158). Among 536 cases and 1,049 controls, higher levels of serum estradiol and estrone in the first trimester of first pregnancies were not associated with higher breast cancer risks overall, but were associated with higher risk of breast cancers diagnosed before age 40 (top vs. bottom quartile RR (95% CI) estradiol = 1.81) (1.08-3.06), p-trend = 0.03; estrone = 1.63 (1.01-2.60), p-trend = 0.04).
Estrogen Metabolites
A woman’s pattern of estrogen metabolism also has been hypothesized to influence her breast cancer risk. Estradiol and estrone can be metabolized through several pathways, including the 2-, 4-, and 16-hydroxylation pathways (159). Products of these pathways have markedly different biologic properties, and opposing hypotheses have been proposed concerning their influence on risk (159). Several epidemiologic studies have examined estrogen metabolites and breast cancer risk, but many have assessed only 2-hydroxyestrone, 16α-hydroxyestrone, and the 2:16α-hydroxyestrone ratio. In three prospective assessments among premenopausal women, nonsignificant inverse associations with the 2-:16α-hydroxyestrone ratio were observed in each (160, 161 and 162). Six prospective studies of either urinary (161, 162 and 163) or circulating (164, 165) metabolite levels among postmenopausal women who were not using postmenopausal hormones also observed no significant associations for 2-hydroxyestrone, 16α-hydroxyestrone or their ratio and breast cancer risk. While these data do not support an important relationship with these metabolites and risk, these studies do not include other biologically active metabolites.
Two recent studies have measured estrogen metabolites in urine (166) or serum (167), using a high performance liquid chromatography/tandem mass spectrometry (LC/MS-MS) assay that measures 15 estrogens and metabolites simultaneously, including parent estrogens and metabolites in the 2-, 4-, and 16-hydroxylation pathways (168). In premenopausal women, urinary levels of estrogen metabolites, measured in the mid-luteal phase of the menstrual cycle, were assessed among 247 cases and 485 controls (166). Higher levels of one metabolite in the 16-hydroxylation pathway, 17-epiestriol, were associated with higher breast cancer risk (top vs. bottom quartile RR = 1.74; 95% CI, 1.08-2.81, p-trend = 0.01). However, higher levels of the parent estrogens, estradiol and estrone, were associated with lower risks of breast cancer (top vs. bottom quartile RR for estradiol=0.51; 95% CI, 0.30-0.86); RR for estrone 0.52; 95% CI, 0.30-0.88). Generally, although not significantly, inverse associations were observed with metabolites in the 2- and 4-hydroxylation pathways. Associations were unchanged with adjustment for plasma estrogens, suggesting women with increased urinary excretion of estrogens are at reduced risk. In postmenopausal women, serum levels of 15 estrogen metabolites were investigated in 277 cases and 423 controls (167). Although several metabolites were individually positively associated with breast cancer risk, none of the associations remained after adjustment for unconjugated estradiol, which was associated with a two-fold increased risk, consistent with other studies. The ratios of 2-hydroxylation pathway:parent estrogens and 4-hydroxy catechols:methylated catechols remained significantly associated with risk after adjustment for unconjugated estradiol: interdecile comparison RR (95% CI) 0.72 (0.52-1.00) and 1.31 (1.03-1.61), respectively.
Androgens and Breast Cancer Risk
Androgens have been hypothesized to increase breast cancer risk either directly, by increasing the growth and proliferation of breast cancer cells, or indirectly, by their conversion to estrogen (60). In animal and in vitro experiments, androgens either increase or decrease cell proliferation, depending upon the model system (169). Dehydroepiandrosterone (DHEA) administered to rodents can decrease tumor formation. In humans, DHEA may act like an antiestrogen premenopausally but an estrogen postmenopausally in stimulating cell growth (170); in part because of the estrogenic effect of its metabolite, 5-androstene-3b, 17b-diol also can bind to the estrogen receptor (171).
In postmenopausal women, the best summary of evidence on circulating androgens and breast cancer risk is from the pooled analysis of nine prospective studies described
above (135) along with the recently published report from the EPIC study (136). In the pooled analysis, testosterone was positively associated with breast cancer risk: The relative risks (95% CI) for increasing quintile category (all relative to the lowest quintile of levels) were 1.3 (1.0-1.9), 1.6 (1.2-2.2), 1.6 (1.1-2.2) and 2.2 (1.6-3.1). Findings were generally similar for several other androgens measured. In EPIC, similar positive associations were observed for each of the androgens assessed. In each of these analyses, when estradiol was added to the statistical models, relative risks for the androgens were only modestly attenuated, suggesting some independent effect of circulating androgens on breast cancer risk. As with estradiol, associations with androgens and breast cancer have tended to be stronger for ER+ tumors than for ER- tumors (141, 142 and 143). Whether this differential is because androgens are serving as a source of estrogens at the breast, or because ER+ tumors are more likely to also be AR+ is unclear. Interpretation of these data is complicated because of possible differences between estradiol and the androgens in terms of assay precision, hormone stability within woman over time, and intracellular conversion of androgens to estrogens that cannot be accounted for in epidemiologic analyses.
above (135) along with the recently published report from the EPIC study (136). In the pooled analysis, testosterone was positively associated with breast cancer risk: The relative risks (95% CI) for increasing quintile category (all relative to the lowest quintile of levels) were 1.3 (1.0-1.9), 1.6 (1.2-2.2), 1.6 (1.1-2.2) and 2.2 (1.6-3.1). Findings were generally similar for several other androgens measured. In EPIC, similar positive associations were observed for each of the androgens assessed. In each of these analyses, when estradiol was added to the statistical models, relative risks for the androgens were only modestly attenuated, suggesting some independent effect of circulating androgens on breast cancer risk. As with estradiol, associations with androgens and breast cancer have tended to be stronger for ER+ tumors than for ER- tumors (141, 142 and 143). Whether this differential is because androgens are serving as a source of estrogens at the breast, or because ER+ tumors are more likely to also be AR+ is unclear. Interpretation of these data is complicated because of possible differences between estradiol and the androgens in terms of assay precision, hormone stability within woman over time, and intracellular conversion of androgens to estrogens that cannot be accounted for in epidemiologic analyses.
The association of plasma testosterone levels and subsequent breast cancer risk also was positive and of the same general magnitude in women using postmenopausal hormones (148). In the two studies previously described, the association between circulating testosterone and breast cancer across categories of predicted breast cancer risk has been addressed. No association was observed between testosterone levels and breast cancer risk in the P-1 trial with 89 cases and 141 non-cases (RR for top versus bottom quartile = 0.5; 95% CI, 0.2-1.1) (145), although the association was noted to be quite robust in the larger NHS cohort (147).
Among premenopausal women, although data are much more limited, prospective nested case-control studies are quite consistent in showing a positive association of similar magnitude to that reported among postmenopausal women between circulating androgen levels and risk of breast cancer (153, 155, 156 and 157, 172, 173).
Progesterone
Progesterone exerts powerful influences on breast physiology and can influence tumor development in rodents (174). Based largely on indirect evidence, progesterone has been hypothesized both to decrease breast cancer risk by opposing estrogenic stimulation of the breast (174) and to increase risk because breast mitotic rates are highest in the luteal (high progesterone) phase of the menstrual cycle (60). In three large prospective studies, results have not been consistent with inverse (156, 172) and no association (157) reported. However, progesterone levels vary substantially throughout the menstrual cycle and are difficult to measure in the context of large epidemiologic studies, hence further assessments with better measures are warranted. In postmenopausal women, only a single prospective study has been conducted and no association found (138).
Prolactin
Prolactin receptors have been found on more than 50% of breast tumors (175), and prolactin can increase the growth of both normal and malignant breast cells in vitro (176). Cumulatively, substantial laboratory evidence suggests that prolactin could play a role in mammary carcinogenesis (177) by promoting cell proliferation and survival (178, 179, 180 and 181), increasing cell motility (182), and supporting tumor vascularization (177, 183). Because prolactin is influenced by both physical and emotional stress (184, 185), levels in women with breast cancer may not reflect their predisease levels. Thus, evaluation of this association in prospective studies is particularly important.
Prolactin levels and risk of breast cancer have been evaluated in several studies to date (137, 150, 151, 186, 187, 188, 189 and 190). Most, though not all (137), studies have observed a significant positive association, with case numbers ranging from 26 (151) to 1,539 (188). In by far the largest study to date, an updated analysis within the NHS and NHSII cohorts with 1,539 cases (premenopausal and postmenopausal women combined), a modest but significant association was observed across quartiles of prolactin level, with a top (versus bottom) quartile RR = 1.4; 95% CI, 1.0-1.9, p-trend = 0.05 (188). In this analysis, the association of prolactin with breast cancer did not differ by menopausal status (p = 0.95). The association was stronger for invasive cases (top vs. bottom quartile RR = 1.4; 95% CI, 1.1-1.7, p-trend = 0.001) than in situ cases (comparable RR = 1.2; 95% CI, 0.8-1.6, p-trend = 0.43). In addition, the association was significantly different by ER/PR status of the tumor (p-heterogeneity=0.03) with RRs for top versus bottom quartiles of 1.6; 95% CI, 1.3-2.0, p-trend <0.001 for ER+/PR+, RR = 1.7; 95% CI, 1.0-2.7, p-trend = 0.06 for ER+/PR-, and RR = 0.9; 95% CI, 0.6-1.3, p-trend = 0.70 for ER-/PR-. Cumulatively, epidemiologic data support a role for prolactin in the etiology of breast cancer.
Insulin-like Growth Factor
Insulin-like growth factor I (IGF-I) is a protein hormone with structural homology to insulin. The growth hormone-IGF-I axis can stimulate proliferation of both breast cancer and normal breast epithelial cells (191). Rhesus monkeys treated with growth hormone or IGF-I show histologic evidence of mammary gland hyperplasia. In addition, positive associations have been observed between breast cancer and birth weight as well as height, which are both positively correlated with IGF-I levels (192). These associations were carefully evaluated in a large pooled analysis, combining data from 17 prospective studies and including 4,790 cases and 9,428 controls (193). Overall, a modest but statistically significant positive association was observed (top vs. bottom 20% of IGF levels RR = 1.28; 95% CI, 1.14-1.44) that did not vary by menopausal status at blood collection. Associations were apparent among ER+ tumors (comparable RR = 1.38; 95% CI, 1.14-1.68) but not ER-tumors (comparable, RR = 0.80; 95% CI, 0.57-1.13; p for heterogeneity = 0.007). The primary IGF binding protein IGFBP-3 was not independently associated with breast cancer risk, and the IGF-I association did not vary by level of IGFBP-3. In addition, the association between IGF-I levels during pregnancy and the mother’s subsequent risk of breast cancer has been assessed. Of two large prospective studies, a positive association was observed in one (194), while no association was seen in the second (195). Reasons for these differences are not clear. Cumulatively, data point to a modest positive association between circulating IGF-I levels and breast cancer risk.
Insulin
Insulin is a known mitogen and circulating levels have been evaluated in relation to subsequent breast cancer risk. Some studies evaluated insulin levels in fasting or nonfasting subjects; others assessed c-peptide levels, which is a marker of insulin secretion. Among premenopausal women, overall no consistent associations have been observed (132, 196, 197 and 198). Similarly, in postmenopausal women, where at least 10 studies with over 2,500 postmenopausal cases have been published, no consistent associations have been reported (132, 197, 198, 199, 200, 201, 202, 203, 204 and 205).
Melatonin
Laboratory evidence in conjunction with recent epidemiologic data suggests a possible relation between melatonin and breast cancer risk. In vitro studies, although not entirely consistent (206), find that both pharmacological and physiologic doses of melatonin reduce the growth of malignant cells of the breast (207, 208, 209, 210 and 211). In rodent models pinealectomy boosts tumor growth (212), whereas exogenous melatonin administration exerts anti-initiating (213) and oncostatic activity (214, 215, 216 and 217) in various chemically induced cancers. The hormone could influence risk through antimitotic or antioxidant activity (218), by modulating cell-cycle length through control of the p53-p21 pathway (211) or by reducing estrogen levels (219, 220).
To date, five prospective studies have assessed the association between urinary 6-sulfatoxymelatonin levels (a metabolite of melatonin) and breast cancer risk. In the first, where a 24-hour urine sample was collected, no association between levels and breast cancer were observed (221). In three subsequent analyses conducted in the Nurses’ Health Studies and the ORDET cohort and utilizing either a first morning urine or a 12-hour overnight urine, consistent inverse associations were observed, with 30-40% lower breast cancer risk seen among women with the highest (versus lowest) melatonin levels (222, 223 and 224). In the most recent prospective analysis (225), no significant association was observed overall. However, in this study, a significant positive association was observed in the first two years of follow-up, and a significant inverse association was seen with longer follow-up, suggesting that time from urine collection to diagnosis may be important. Additional studies are clearly needed. There is relatively consistent indirect evidence from observational studies for an association between night work and breast cancer risk (226). Night work is associated with substantially reduced melatonin levels (227, 228). Two retrospective studies of flight attendants with occupational exposure to light at night linked employment time to an increased breast cancer risk (229, 230). Two nationwide record linkage studies (231, 232) and a retrospective case-control study (219) associated night work with an approximately 50% higher breast cancer risk. In the only two prospective studies, working 20 to 30 or more years of rotating night work as a nurse was associated with an increased risk of breast cancer (233, 234).
Other Hormones and Hormone Scores
In one prospective study, the influence of multiple hormones, considered simultaneously, on postmenopausal breast cancer risk was evaluated (235). Postmenopausal levels of estrone, estradiol, estrone sulfate, testosterone, androstenedione, dehydroepiandrosterone (DHEA), DHEA sulfate, and prolactin and, secondarily, IGF-I and c-peptide, were evaluated among 265 cases and 541 controls. Several hormone scores were evaluated, including ranking women by the number of hormones above the age- and batchadjusted geometric mean. Having seven or eight compared to zero hormones above the geometric mean level was associated with total (RR = 2.7; 95% CI, 1.3-5.7, p trend <0.001) and ER+ (RR = 3.4; 95% CI, 1.3-9.4, p trend <0.001) breast cancer risk. Overall, these results suggest that multiple hormones with high circulating levels substantially increase the risk of breast cancer, particularly ER+ disease.
Anti-Müllerian hormone (AMH; also called Müllerian inhibiting substance [MIS]) is produced in ovarian granulosa cells and plays a key role in regulating folliculogenesis (236). Circulating levels vary over a woman’s life, being low or undetectable before puberty, peaking from puberty through the premenopausal years, and becoming undetectable after menopause. Although limited laboratory data suggest a protective role of AMH in breast carcinogenesis, the one prospective study to date observed a significant positive association between circulating AMH and breast cancer risk (237). With 105 cases and 204 matched controls, RR (95% CI) with increasing quartile categories were 1.0, 2.8, 5.9, 9.8 (3.3-28.9). Associations appeared somewhat stronger among women ≥55 versus <55 years of age at diagnosis (top vs. bottom quartile RR [95% CI]: <55 years = 3.9 [0.9-16.3]; ≥55 years = 9.6 [2.8-33.3]). This strong association deserves further study, because AMH has not been assessed in any other prospective studies to date.
ORAL CONTRACEPTIVES
Since oral contraceptives were first introduced in the 1960s, they have been used by millions of women (238). Most combined oral contraceptives contain ethinyl estradiol (or mestranol, which is metabolized to ethinyl estradiol) and a progestin. The estrogen dose in oral contraceptives has ranged from at least 100 mg in 1960 to 20-30 mg, the doses most commonly used today; during this same time period, at least nine different progestins have been used (239, 240). Patterns of use also have changed considerably over time, with both increasing durations of use and a trend toward earlier age at first use. Over 70 epidemiologic studies have evaluated the relationship between oral contraceptive use and breast cancer risk.
Any Use and Total Duration of Use
Most studies have observed no significant increase in breast cancer risk even with long durations of use. Individual data from 54 epidemiologic studies were collected and analyzed centrally (86). In this large pooled analysis, in which data from 53,297 women with and 100,239 women without breast cancer were evaluated, no overall relationship was observed between duration of use and risk of breast cancer. Similar findings were generally observed when long-term use was evaluated among either postmenopausal women or women over the age of 45 years.
Recency of Use
In the pooled analysis (86), current and recent users of oral contraceptives had an increased risk of breast cancer (for current vs. never-users, RR = 1.24). This increased risk disappeared within 10 years of stopping oral contraceptive use (relative risk by years since stopping use vs. never use: 1-4 years, 1.16; 5-9 years, 1.07; 10-14 years, 0.98; more than 15 years, 1.03) (Fig. 18-8). When the investigators evaluated both time since last use and duration of use, they observed a modestly increased risk only among current and recent users, and no independent effect of long duration of use on the risk of breast cancer even among very young women. In more recent studies, past use of oral contraceptives (generally use in the more distant past) also has not been associated with breast cancer mortality (241, 242 and 243). Thus, the increased risk of breast cancer observed among young, longterm users of oral contraceptives in past individual studies (and meta-analyses) appears due primarily to recency of use rather than to duration. These data suggest that oral contraceptives may act as late-stage promoters. Importantly, current and recent users, the women who appear to have a modest increase in risk, are generally young (under 45 years of age) and thus have a low absolute risk of breast cancer. Hence, a modest increase in their risk will result in few additional cases of breast cancer. Nevertheless, this apparently
increased risk among current and recent users should be considered in deciding whether to use oral contraceptives. On the basis of these data in conjunction with supporting laboratory evidence, the International Agency for Research on Cancer (IARC) classified oral contraceptives as carcinogenic to humans (i.e., group 1 carcinogens) in 2005 (244).
increased risk among current and recent users should be considered in deciding whether to use oral contraceptives. On the basis of these data in conjunction with supporting laboratory evidence, the International Agency for Research on Cancer (IARC) classified oral contraceptives as carcinogenic to humans (i.e., group 1 carcinogens) in 2005 (244).
Use before a First Pregnancy
Because any influence of oral contraceptives on the breast has been hypothesized to be greatest prior to the cellular differentiation that occurs with a full-term pregnancy (245), a number of investigators have evaluated the effect of oral contraceptive use prior to a first full-term pregnancy. In two meta-analyses, the summary relative risk indicated a modest increase in risk with long-term use (246, 247). In the pooled analysis (86), a significant trend of increasing risk with first use before age 20 years was observed. Among women ages 30-34 years, the relative risk associated with recent oral contraceptive use was 1.54 if use began before age 20 years and 1.13 if use began at age 20 years or older. Overall, there was no consistent evidence of a differential effect according to type or dose of either estrogen or progestin, but few studies had examined this issue (60).
Risk according to Breast Cancer Risk Factor Profile
Possible interactions with other breast cancer risk factors were evaluated in detail for the first time in the collaborative pooling project (86). In this study, the investigators defined oral contraceptive use in terms of recency and age at first use, rather than “ever use,” as done in most previous individual studies. Overall, the relationship between oral contraceptive use and breast cancer did not vary appreciably by family history of breast cancer, weight, alcohol intake, or other breast cancer risk factors. In a recent metaanalysis among women with BRCA1 and BRCA2 mutations (248), neither ever use nor duration of use was associated with breast cancer risk. However, use of oral contraceptives prior to 1975 was associated with a modest increased risk while use after 1975 was not associated with risk, suggesting possible differences by formulation.
Receptor Status and Histologic Subtypes of Breast Cancer
Few studies have addressed possible differences in these associations by estrogen receptor status or by intrinsic molecular subtype (e.g., luminal A, luminal B subtypes). Ever use of oral contraceptives was assessed by hormone receptor status in eight case-control studies (249). Results were inconsistent, and only one study reported a significantly stronger association for ER- than for ER+ breast cancer. Only three of these studies evaluated time since last use, and again results were mixed. Recently, in the Black Women’s Health Study, ever use of oral contraceptives was significantly stronger for ER-/PR- breast cancers (ever versus never use RR = 1.7; 95% CI, 1.2-2.3) than for ER+/PR+ breast cancers (comparable RR = 1.1; 95% CI, 0.9-1.4) (250). In the Women’s Health Initiative, no association was observed between total duration of use and triple negative breast cancer; recent or current use could not be examined in this older population. In case-case analyses, compared to luminal A breast cancers, luminal B cases were less likely to ever use oral contraceptives in one study (251) but not in a second study (252); no differences were observed with triple negative, basal-like or HER-2 breast cancers, although case numbers were small. More data are needed to determine if oral contraceptives differentially influence breast cancer subtypes.
Newer Oral Contraceptive Formulations and Specific Formulations
Until the time of the large pooled analysis published in 1996, limited data existed regarding the influence of the newer oral contraceptive formulations on breast cancer risk (86) and data on specific formulations was particularly sparse. In a study that assessed risk by hormonal dose/potency, the relative risks associated with recent use of lower dose formulations were generally lower than relative risks associated with higher dose formulations (253). Further, associations appeared to vary by type of progestin used (with borderline significant positive associations observed for levonorgestrel, ethynodiol diacetate, and norethindrone acetate) although these differences were based on small numbers. In the population based Women’s CARE case-control study (254), overall no increased risk of breast cancer among current users or former users was observed regardless of estrogen or progestin dose. Among specific formulations, an increased risk was observed for current use of ethynodiol diacetate, and no association was seen with levonorgestrel formulations. In the Nurses’ Health Study II, the only prospective cohort to examine newer generation oral contraceptives as well as specific formulations, current use of any oral contraceptives was associated with a modest increase in risk (RR = 1.33; 95% CI, 1.03-1.73) (255). However, this association was largely accounted for by use of a single type of preparation, triphasic contraceptives with levonorgestrel (comparable RR = 3.05; 95% CI, 2.00-4.66). Although no firm conclusions can yet be drawn, accruing data suggest that specific oral contraceptive formulations indeed may have a differential impact on breast cancer risk.
Progestin-only Contraceptives
Progestin-only contraceptives include progestin-only pills (“mini-pill”), depot-medroxyprogesterone (DMPA, an injectable contraceptive), and implantable levonorgestrel
(Norplant); very few epidemiologic studies have evaluated their association with breast cancer risk. Longer-term users of the progestin-only pill have been observed to have either a similar or lower risk of breast cancer than never-users (256). Four of five case-control studies reported relative risks of 1.5-2.2 associated with recent use of DMPA versus non-use (257, 258 and 259), while a fifth study found no association regardless of recency or duration (260). As with other contraceptives, the risk appears to subside several years after stopping use. In the only study to have assessed these associations, the relative risks didn’t vary by tumor hormone receptor subtype or histologic subtype. Norplant, a longacting contraceptive that is implanted subdermally, was introduced in the United States only in 1990. In the CARE study, no association was observed for ever Norplant use, although only 12 women were exposed (260). Further epidemiologic research is needed for each of these drugs.
(Norplant); very few epidemiologic studies have evaluated their association with breast cancer risk. Longer-term users of the progestin-only pill have been observed to have either a similar or lower risk of breast cancer than never-users (256). Four of five case-control studies reported relative risks of 1.5-2.2 associated with recent use of DMPA versus non-use (257, 258 and 259), while a fifth study found no association regardless of recency or duration (260). As with other contraceptives, the risk appears to subside several years after stopping use. In the only study to have assessed these associations, the relative risks didn’t vary by tumor hormone receptor subtype or histologic subtype. Norplant, a longacting contraceptive that is implanted subdermally, was introduced in the United States only in 1990. In the CARE study, no association was observed for ever Norplant use, although only 12 women were exposed (260). Further epidemiologic research is needed for each of these drugs.
POSTMENOPAUSAL HORMONE USE
Postmenopausal estrogens have been used for more than half a century. By the mid-1970s, almost 30 million prescriptions were being filled annually in the United States (261). A challenge in studying the relationship between postmenopausal hormones and breast cancer is the substantial variation in formulations and patterns of use that has occurred over time. By the time sufficient use of one type of hormone has occurred to allow a detailed epidemiologic evaluation, new formulations are already being introduced.
The possible relation between postmenopausal estrogen use and risk of breast cancer has been investigated in more than 50 epidemiologic studies over the past 40 years. Most of these studies focused on unopposed estrogen and have been summarized in meta-analyses (262, 263, 264, 265, 266 and 267) and a large pooled analysis (268). More recently, data from randomized controlled trials have confirmed the epidemiologic relations of combination estrogen plus progestin hormone therapy to increased risk of breast cancer, and IARC has now classified estrogen plus progestin therapy as a human carcinogen (269). On the other hand, the Women’s Health Initiative randomized trial did not observe an increase in risk with short term use (median 5.9 years) of unopposed estrogen (270), although the duration of use was shorter than in the epidemiologic studies that continue to show increased risk with longer durations of unopposed estrogen therapy (15 plus years of current use) (271). A summary of these findings, plus a more detailed discussion of several of the most important and most recent studies, is provided below. Particular attention is focused on use of estrogen alone versus estrogen plus progestin therapy.
Any Use
All meta-analyses have concluded that overall, ever users of postmenopausal estrogens have little or no increase in risk of breast cancer compared with women who have never used this therapy. Depending on the inclusion criteria for the meta-analyses, the RR estimates across studies range from 1.01 to 1.07. The RR observed in the pooled individual patient data analysis was 1.14 (268). However, as for oral contraceptives, ever use is a poor measure of exposure because it fails to distinguish between short and long duration and recent and past users, nor does it distinguish type of hormone therapy used.
Duration of Use
In the meta-analyses, significant increases in risk of approximately 30% to 45% with more than 5 years of use have been observed. In updated results from the Nurses’ Health Study (272), with 1,935 breast cancer cases, an excess risk of breast cancer was limited to women with current or very recent use of postmenopausal hormones. Within this group, the risk increased with longer duration of use and was statistically significant among current users who have used for 5 or more years (e.g., compared to never users of postmenopausal hormones, RR for ≥10 years of use =1.47; 95% CI, 1.22-1.76) (272). While the WHI with median duration of use of unopposed estrogen (5.9 years) shows no excess risk of breast cancer (270), longer durations in epidemiologic studies show significant increase in risk (273). With over 5,600 invasive breast cancer cases in the Nurses’ Health Study, Chen et al. show that risk is significantly increased beyond 10 years of current use and continues to increase with longer durations (274).
Risk is greater for users of estrogen plus progestin compared to users of estrogen alone (275, 276 and 277). These epidemiologic results were corroborated by the Women’s Health Initiative, a randomized controlled trial of estrogen plus progestin use that showed a significant increase in risk of breast cancer with duration of use of this hormone combination (40). Given the high dropout and noncompliance with therapy during the trial (approximately 40% stopped taking drug or placebo in each arm), analysis of compilers showed a substantially greater increase in risk with duration of therapy (278), closer to that observed in epidemiologic studies that by their nature evaluate risk among compliers or users of hormone therapy.
Recency of Use
Data on recency of use have been sparse because many studies do not distinguish current from past users. One meta-analysis calculated an RR for current use of 1.63 for women with natural menopause and 1.48 for women with surgical menopause. In a second, the summary RR was 1.40 (95% CI, 1.20-1.63) comparing current to never users. In the report from the Nurses’ Health Study cohort (272), an excess risk of breast cancer was limited to women with current or very recent use of postmenopausal hormones. In the Breast Cancer Detection Demonstration Project (BCDDP) cohort, a positive association with invasive breast cancer was noted among current users with ≥5 to 15 years of use (279). In the U.K. Million Women Study, risk was likewise substantially larger among current users than past users, and returned to the risk of never users within 4 years of stopping use (280).
These relationships were evaluated in considerable detail in the pooled analysis that combined results of 51 epidemiologic studies (268). Importantly, in these analyses, women with an uncertain age at menopause were excluded (e.g., women with simple hysterectomies) because inadequate accounting for age at menopause in the analysis can lead to substantial attenuation of the observed relationships between postmenopausal hormone use and breast cancer risk (281). The median year of case diagnosis was before 1990 in the majority of studies and accordingly few recorded type of hormone used. The vast majority of use across these 51 studies was unopposed estrogen, but type of hormone therapy was not addressed in the overall assessment of these data. The investigators observed a statistically significant association between current or recent use of postmenopausal hormones and risk of breast cancer; the positive association was strongest among those with the longest duration of use (Fig. 18-9). For example, among women who used postmenopausal hormones within the previous 5 years (compared to never users of postmenopausal hormones), the RRs for duration of use were 1.08 for 1 to 4 years
of use, 1.31 for 5 to 9 years, 1.24 for 10 to 14 years, and 1.56 for 15 years or more of use. No significant increase in breast cancer risk was noted for women who had quit using postmenopausal hormones 5 or more years in the past, regardless of their duration of use. Whether this holds true for all types and durations of use of hormone therapy remains to be evaluated more precisely.
of use, 1.31 for 5 to 9 years, 1.24 for 10 to 14 years, and 1.56 for 15 years or more of use. No significant increase in breast cancer risk was noted for women who had quit using postmenopausal hormones 5 or more years in the past, regardless of their duration of use. Whether this holds true for all types and durations of use of hormone therapy remains to be evaluated more precisely.
Type, Dose, and Mode of Delivery of Estrogen
Limited data are available regarding the effects of dose or type of estrogen on breast cancer risk. Again, the best data come from the pooled analysis (268). No significant differences in the RRs were observed according to either the type of estrogen used (conjugated estrogen vs. other) or the estrogen dose (<0.625 versus ≥1.25 mg), although some modest differences in estimates suggested that further evaluation is warranted.
Although the effect of estrogen use on breast cancer risk could be reasonably hypothesized to vary by mode of estrogen delivery (e.g., patch estrogen, by avoiding the first pass effect in the liver, does not increase SHBG to the extent that oral preparations do), no important differences are observed in the largest study to date; the Million Women Study included over 40,000 users of transdermal estrogen and observed no significant difference in relative risk of breast cancer (1.24) compared to that among the 60,000 users of oral therapy (1.32) (277).
Time since Menopause, Initiating Use of Hormone Therapy, and Risk
Considerable evidence has recently accumulated addressing timing of use of hormones and the magnitude of the adverse effect on breast cancer risk (282). A rigorous analysis of the U.K. Million Women Study shows risk is substantially greater among women who start hormone therapy before or less than 5 years after menopause. This effect is observed for estrogen alone and for combination estrogen plus progestin (280). The U.K. Million Women Study contrasts with the WHI where 90% of women in the estrogen-only arm of the trial were more than 5 years beyond menopause at randomization.
Risk according to Breast Cancer Risk Factor Profile
The risk associated with postmenopausal hormone use was assessed in a number of specific subgroups in the pooled analysis (268). Risk did not appear to vary according to reproductive history, alcohol intake, smoking history, or family history of breast cancer. However, the RRs associated with 5 or more years of postmenopausal hormone use were highest among the leanest women (p for
heterogeneity = 0.001); this interaction has been consistently observed (271, 277, 283). Risk for unopposed estrogen therapy is also more clearly observed to increase with duration of use among women with bilateral oophorectomy than those without (271), again consistent with precise statistical control for underlying risk of breast cancer because age at menopause is more accurately assessed in women undergoing bilateral oophorectomy than in those who have hysterectomy without oophorectomy (284). This consistent finding that risk of unopposed estrogen is attenuated among overweight and obese women may account for the apparent lower risk of breast cancer among women in the WHI trial of unopposed estrogen, given the overweight and obese population included in the trial (285).
heterogeneity = 0.001); this interaction has been consistently observed (271, 277, 283). Risk for unopposed estrogen therapy is also more clearly observed to increase with duration of use among women with bilateral oophorectomy than those without (271), again consistent with precise statistical control for underlying risk of breast cancer because age at menopause is more accurately assessed in women undergoing bilateral oophorectomy than in those who have hysterectomy without oophorectomy (284). This consistent finding that risk of unopposed estrogen is attenuated among overweight and obese women may account for the apparent lower risk of breast cancer among women in the WHI trial of unopposed estrogen, given the overweight and obese population included in the trial (285).
Use of Estrogen Plus Progestin (E & P)
The addition of a progestin to estrogen regimens became increasingly common through the 1990s because it minimizes or eliminates the increased risk of endometrial hyperplasia and cancer associated with using unopposed estrogens. In the United States, by the mid-1980s, almost 30% of postmenopausal hormone prescriptions included a prescription for progestin (286). The impact of an added progestin to the risk of breast cancer has been evaluated only in the last 25 years.
Two of the first studies to assess this relationship suggested that the addition of a progestin could decrease breast cancer risk (287, 288). However, these studies were small and potentially important confounders (e.g., age and parity) were not accounted for in the analyses. Since this time, several additional studies have assessed this relationship and together indicate that a protective effect of typical doses used in postmenopausal hormone therapy can be ruled out (268). Prospective studies reporting on this relationship had similar findings. Bergkvist et al. (289) observed an RR of 4.4 (95% CI, 0.9-22.4) among women who used estrogen plus progestin for 6 or more years compared to never users. Women using hormones for a shorter duration did not appear to be at increased risk, but CIs again were wide and did not exclude either a modest increase or a decrease in risk. In findings from the Nurses’ Health Study (272), in which among women using progestins, about twothirds used 10 mg of medroxyprogesterone for 14 or fewer days per month, the RR associated with current estrogen plus progestin use versus never use was 1.4 (95% CI, 1.2-1.7). The BCDDP found that the risk of breast cancer went up by about 1% for every year that women took estrogen alone and about 8% for every year that they took estrogen plus progestin (290). Although these yearly increases in risk seem minimal, their accumulation over time is of concern. For example, if women take estrogen with progestin for 10 years, their risk of breast cancer will be 80% higher than if they had never used hormones (291). For both types of therapy, however, this increase in risk begins to drop after hormone use stops (268). In the pooled analysis (268), data on the postmenopausal hormone formulation were available from only 39% of women, and only 12% of these reported using estrogen plus a progestin. The RR associated with 5 or more years of recent use, relative to never use, was 1.53. More recent case-control studies also support this increase in risk with combination estrogen plus progestin (292, 293). The Women’s Health Initiative randomized controlled trial of estrogen plus progestin versus placebo among 16,608 postmenopausal women with an intact uterus, ages 50 to 79, stopped early at the recommendation of the data safety monitoring board (DSMB) in part due to the prespecified harm boundary for breast cancer (40). At an average of 5.2 years of follow-up, women randomized to estrogen plus progestin had a significantly elevated risk of breast cancer, RR compared to placebo 1.26 (95% CI, 1.00-1.59), and analysis accounting for adherence showed an RR of 1.49 for estrogen plus progestin. Importantly, the trend for increasing risk with increasing duration of use of estrogen plus progestin was also significant. These results among women adherent to use of estrogen plus progestin at an average of 5 years of use are consistent with the epidemiologic data, which also shows the trend in risk with duration of use continuing to increase beyond 5 years and no evidence of a plateau (274).
In addition to their effect on breast cancer, postmenopausal hormones also have a major impact on other aspects of women’s health. Results from the Women’s Health Initiative (a large randomized clinical trial) definitively show that after 5 years of use, estrogen plus progestin does more overall harm to women than good (40), although the Women’s Health Initiative studied only one type and dose of estrogen plus progestin (Prempro); because widespread use of estrogen plus progestin is relatively recent, few data are available to evaluate the effect of different formulations, doses, or schedules of use of progestin on risk of breast cancer (294, 295). The British Million Women Study with over 9,000 cases of breast cancer during follow-up again confirmed the excess risk of breast cancer among women currently using combination estrogen plus progestin and noted this is significantly greater relative risk than among women using estrogen alone. Risk increased with duration of use but did not vary significantly according to the progestagen content or whether use was sequential or continuous (277). The possibility remains that dose of progestagen is important, including total monthly exposure, but variation in studies to date has not allowed rigorous and valid comparisons. Despite issues such as dose of progestagen that remain open research questions, additional follow-up of the WHI trial participants shows excess breast cancer mortality among the women receiving estrogen plus progestin compared to placebo with almost double the risk of death from breast cancer through 11 years of follow-up (296).
Receptor Status and Histologic Subtypes of Breast Cancer
Consistent evidence from larger epidemiologic studies shows combination estrogen plus progestin and unopposed estrogen therapy are associated with increased risk of ER+ breast cancer (297). While the WHI did not observe any significant difference in the distribution of invasive cancer by receptor status, the trial had limited power to detect an association with fewer than 500 cases of breast cancer. Some have suggested that risk is limited to lobular subtypes of breast cancer (298), but the majority of evidence does not support this claim. Also, given the higher proportion of receptor positive tumors in lobular rather than ductal cancers, a stronger relative risk observed for lobular cancer (297) would be expected for this subset of breast cancers. This is confirmed in the Million Women Study with over 8,000 cases of invasive ductal and over 1,500 invasive lobular cancers, where significant increases in risk of ductal carcinoma are observed for estrogen alone and for combination therapy (273).
Decline in Breast Cancer Incidence
Numerous studies in the United States and internationally have reported on the decline in breast cancer incidence after 2002. Based on data from the San Francisco mammography registry, prescribing of E&P peaked in 1999. Before publication of the Heart and Estrogen/progestin Replacement Study (HERS) the use of hormone therapy was increasing at 1% per quarter, but declined by 1% per
quarter after the publication (299). This decline in prescribing continued until the publication of the WHI in 2002, at which point a more substantial decline of 18% per quarter was observed. The peak and decline through 1999 to 2002 is concordant with the HERS report (300) in 1998 showing a significant increase in coronary heart disease (CHD) in the first year of therapy among women with prevalent coronary disease, and in addition, no long-term benefit in reducing CHD (301). The growing epidemiologic evidence published since 2000 on the adverse effects of combination therapy on breast cancer added further evidence against the use of this therapy. Based on a prevalence of use of E&P in California, Clarke et al. estimated a population attributable risk (PAR or the proportion of cases caused by E&P) of up to 11% based on a prevalence of use of 30% and a relative risk of 1.4 (302). Given that substantially higher relative risks of 2 or more have been reported (277), this estimate of the PAR is conservative. Assuming a prevalence of use of 17.5%, the average reported for California in 2001 (302), a relative risk of 1.49 gives a PAR of 7.9% and a relative risk of 2.0 gives a PAR of 14.9%.
quarter after the publication (299). This decline in prescribing continued until the publication of the WHI in 2002, at which point a more substantial decline of 18% per quarter was observed. The peak and decline through 1999 to 2002 is concordant with the HERS report (300) in 1998 showing a significant increase in coronary heart disease (CHD) in the first year of therapy among women with prevalent coronary disease, and in addition, no long-term benefit in reducing CHD (301). The growing epidemiologic evidence published since 2000 on the adverse effects of combination therapy on breast cancer added further evidence against the use of this therapy. Based on a prevalence of use of E&P in California, Clarke et al. estimated a population attributable risk (PAR or the proportion of cases caused by E&P) of up to 11% based on a prevalence of use of 30% and a relative risk of 1.4 (302). Given that substantially higher relative risks of 2 or more have been reported (277), this estimate of the PAR is conservative. Assuming a prevalence of use of 17.5%, the average reported for California in 2001 (302), a relative risk of 1.49 gives a PAR of 7.9% and a relative risk of 2.0 gives a PAR of 14.9%.
Evidence for breast cancer incidence rates now clearly shows a parallel drop in breast cancer consistent with the pattern of decreased prescribing. The rigorous, state-of-theart analysis by Jemal et al. (303) using joint point analysis and drawing on SEER incidence data from 1975 through 2003 shows that there is a significant decrease in incidence of invasive breast cancer from 1999 to 2003 in all 5-year age groups from 45 years and above, and a sharp decrease largely limited to ER positive tumors in age groups 50 to 69 between 2002 and 2003. Furthermore, while others have suggested that a 1% to 3% drop in screening mammography may account for this drop in incidence, Jemal et al. show strong evidence against this. If screening was to account for a drop in incidence, rates of in situ disease would also need to drop because they are almost only detected by mammography. Prior to screening becoming widespread, Jemal et al. show in situ rates were low and rose with the uptake of screening to plateau from 1999 through 2003. The lack of a drop in in situ cancer offers compelling evidence that a reduction in screening does not account for the drop in incidence of invasive breast cancer.
Others have analyzed SEER data over a shorter period (30) or draw on the unique resources of the California tumor registry and the health maintenance organization (HMO) data sets (304) to show similar relations between change in hormone therapy and a decrease in breast cancer incidence. Robbins and Clarke (305) have also evaluated the change in prescribing as estimated from the California Health Interview Survey (CHIS) for almost 3 million non-Hispanic white women aged 45 to 74 against the change in breast cancer incidence across 58 counties in California. This thoughtful analysis shows that from 2001 to 2004, incidence declined by 8.8% in the counties with the smallest E&P reductions, by 13.9% in those with intermediate reductions, and by 22.6% in counties with the largest reductions in combination postmenopausal hormone therapy (305). Between 2001 and 2003, CHIS data did not show any significant change in the proportion of women who reported having a mammogram in the previous two years adding further evidence against this as a plausible major explanatory factor in the observed declines in incidence. Analysis of women undergoing routine mammography in San Francisco rules out a drop in screening as a cause of the decrease in incidence and confirms other reports of the changes in incidence of invasive breast cancer (306). Even more evidence in support of this relation between decrease in E&P and breast cancer comes from declines in incidence that parallel those in the United States as reported in New Zealand (307), Australia (308), and Germany (309). Based on these data and the IARC classification of estrogen plus progestin as a carcinogen, we can conclude that removal of estrogen plus progestin acting as a promoter accounts for this rapid drop in incidence (310).
Summary of Postmenopausal Hormone Use and Breast Cancer Risk
Although some aspects of the relationship between postmenopausal hormones and breast cancer risk remain unresolved, several areas of clear agreement have emerged. The finding of no increase in risk comparing ever users to never users is consistent and reassuring. However, much of that observation reflects the experience among short-term users and hormone use in the past, predominantly unopposed estrogen.
Overall, the findings also indicate an increased risk in two important subgroups of users: users of long duration and current users. These increases are particularly marked among women beginning use within 5 years of menopause. In general, users of long duration are more likely to be current users, so in many studies these two groups overlap substantially. From a biological perspective, these are the groups one would most expect to demonstrate a relation with breast cancer risk, because exogenous estrogens appear to act as a promoter at a late stage.
The increase in breast cancer risk associated with estrogen plus progestin use appears considerably greater than that for use of estrogen alone. Combination estrogen plus progestin therapy increases mortality from breast cancer, not just incidence of disease. The impact on risk of differing progestins and patterns of use of progestins remains to be resolved.
GENETIC SUSCEPTIBILITY TO BREAST CANCER
Hereditary Syndromes
Family history of breast cancer is an accepted risk factor for breast cancer; however, the proportion of breast cancer estimated to be due to rare highly penetrant genes such as BRCA1 and BRCA2 is less than 10% (311), perhaps as low as 3% (312). A few highly penetrant genes and hereditary syndromes for breast cancer are described below; however, a more extensive discussion of this topic is covered in Chapter 17, Inherited Genetic Factors and Breast Cancer. Among 2,389 incident cases of breast cancer occurring in the Nurses’ Health Study between 1976 and 1988, the ageadjusted RR associated with having a maternal history of breast cancer was 1.8 (95% CI, 1.5-2.0) (313). This risk rose to 2.1 if the mother’s breast cancer was diagnosed before age 40. Having a sister with breast cancer was associated with a RR of 2.3, and this rose to 2.5 for having both and mother and a sister with breast cancer. Risk of developing breast cancer by age 70 for a 30-year-old woman with both a mother and sister history of breast cancer was estimated to be 17.5%. Segregation analyses of breast-cancer-prone families showed that inheritance in these families is consistent with an autosomal dominant mode of inheritance (314). These families represent a heterogeneous group of syndromes such as the Li-Fraumeni syndrome (a disorder that includes predisposition to sarcomas, lung cancer, brain cancer, leukemia, lymphoma, and adrenal-cortical carcinoma), Cowden disease (a syndrome involving mucocutaneous and gastrointestinal lesions and breast cancer), and a syndrome called by some “early onset breast cancer” (314) in which
breast cancer often occurs in the 20s and 30s. The molecular basis for certain of these syndromes is associated with high penetrance mutations. The Li-Fraumeni syndrome is due to germline mutations in the p53 gene (315). Cowden syndrome is due to germline mutations of the PTEN gene (316). The breast cancer susceptibility gene on chromosome 17q was called BRCA1 and was cloned in 1994 (317). A second breast cancer susceptibility locus, BRCA2, was localized on chromosome 13q and cloned in 1995 (318). Estimates of the cumulative lifetime risk of breast cancer in BRCA1 and BRCA2 carriers range from about 85% (estimated from the families selected for linkage analysis) to 50% or even less (estimated from population-based studies) (311). The higher estimates from the linkage analysis studies could be due to higher penetrance mutations in these families or to ascertainment bias resulting in failure to select families in which BRCA1 and BRCA2 mutations are present but do not give rise to a sufficiently striking breast cancer predisposition to qualify for enrollment into the linkage studies. In case series from “high-risk” clinics to which women with a notable family history of breast cancer are referred, BRCA1 mutations may be responsible for 20% to 30% of early-onset breast cancer (319). However, estimates in unselected breast cancer cases are much lower, in the range of 2% to 3% (319, 320). Estimates for BRCA2 tend to be lower (319). In unpublished data from the Nurses’ Health study, only 2 of 192 consecutive cases had truncation mutations in BRCA1, and 1 of 192 had a truncation mutation in BRCA2. Genetic testing and management of patients with highly penetrant mutations is discussed in Chapter 19. Moderate penetrance genes with minor allele frequencies (MAFs) ranging from .0005-.01 such as CHEK2 (rare deletion mutation) and PALB2 also increases breast cancer risk (321).
breast cancer often occurs in the 20s and 30s. The molecular basis for certain of these syndromes is associated with high penetrance mutations. The Li-Fraumeni syndrome is due to germline mutations in the p53 gene (315). Cowden syndrome is due to germline mutations of the PTEN gene (316). The breast cancer susceptibility gene on chromosome 17q was called BRCA1 and was cloned in 1994 (317). A second breast cancer susceptibility locus, BRCA2, was localized on chromosome 13q and cloned in 1995 (318). Estimates of the cumulative lifetime risk of breast cancer in BRCA1 and BRCA2 carriers range from about 85% (estimated from the families selected for linkage analysis) to 50% or even less (estimated from population-based studies) (311). The higher estimates from the linkage analysis studies could be due to higher penetrance mutations in these families or to ascertainment bias resulting in failure to select families in which BRCA1 and BRCA2 mutations are present but do not give rise to a sufficiently striking breast cancer predisposition to qualify for enrollment into the linkage studies. In case series from “high-risk” clinics to which women with a notable family history of breast cancer are referred, BRCA1 mutations may be responsible for 20% to 30% of early-onset breast cancer (319). However, estimates in unselected breast cancer cases are much lower, in the range of 2% to 3% (319, 320). Estimates for BRCA2 tend to be lower (319). In unpublished data from the Nurses’ Health study, only 2 of 192 consecutive cases had truncation mutations in BRCA1, and 1 of 192 had a truncation mutation in BRCA2. Genetic testing and management of patients with highly penetrant mutations is discussed in Chapter 19. Moderate penetrance genes with minor allele frequencies (MAFs) ranging from .0005-.01 such as CHEK2 (rare deletion mutation) and PALB2 also increases breast cancer risk (321).
“Sporadic” and Later-onset Breast Cancers
As the high-penetrance genes responsible for single gene disorders have been found, the field of genetic epidemiology has seen a shift to studies using unrelated controls, often described as “association studies.” This has been largely motivated by the lack of power in family-based studies if allele penetrance is low, as few members of even large families will be affected. There are additional parameters that can be calculated in association studies; for instance, to assess the population attributable risk for alleles associated with familial risk or specific allelic variants, it is necessary to screen population-based case series.
Low-penetrance Alleles and Breast Cancer Risk
Until recently, the main method used in the search for these low-penetrance alleles has been the “candidate gene” approach in which polymorphic variants in genes that plausibly influence breast cancer risk are assessed in conventional epidemiologic studies (i.e., case-control or cohort studies). The principal candidates studied have been genes involved in steroid hormone metabolism, carcinogen metabolism genes, and genes that may influence cell proliferation. Despite a large number of positive reports of association in a single study, few of these reports have been replicated (322). The failure to replicate initially positive findings has been ascribed to a variety of factors including publication bias, the “winner’s curse” phenomenon (the first report of an association is often more positive than subsequent studies), underpowered studies, multiple comparisons, and genetic heterogeneity (322). The major problem with candidate gene studies may be the low prior probability associated with any specific candidate gene chosen from among the approximately 24,000 human genes. Despite this, when all published studies are combined, approximately 20% to 30% of association studies yield statistically significant pooled estimates, usually of modest effects (322, 323). Until recently, none of these replicated positives have applied to breast cancer. Recent results from the Breast Cancer Association Consortium suggest that a nonsynonymous polymorphism in a coding variant (D302H) in Caspase 8 (Casp8) is associated with lower risk of breast cancer (324).
Genome-wide Scans and Cancer Susceptibility
Advances in genotyping technology coupled with decreases in genotyping cost have enabled genome-wide association studies (GWAS) in large-scale study populations. In contrast to the candidate gene approach, GWAS offer the potential to conduct a comprehensive and unbiased search for modest associations (325). The generation of a draft sequence of the human genome led to subsequent efforts to define the spectrum of variability in the sequence. These efforts include the International HapMap and 1000 Genomes Project.
The International HapMap provides a database of common SNPs (single nucleotide polymorphisms; defined as SNPs with minor allele frequency, MAF, >5%) at an average spacing of every 1,250 bases across the 3 billion base pairs of genomic sequence (326). Analysis of this dataset indicates that over 90% of the nearly 10 million common SNPs estimated to exist are highly correlated with at least one other SNP (a phenomenon known as linkage disequilibrium). This observation suggests that much of the information on genetic variation can be extracted with the genotyping of a carefully chosen subset of SNPs called tagSNPs, which can serve as surrogates for untested SNPs. The informativeness of a set of tagSNPs can be increased by selecting SNPs that maximize the r2 to untyped SNPs in a region, and further increased by ranking SNPs according to the number of proxies they have (327). The extent of diversity in population genetics history is evident in the substantial differences in patterns of linkage disequilibrium between the multiple continental populations studied in HapMap 1 included European, East Asian, and West African populations. Draft 1 of the HapMap 3 data release includes 1,301 samples from 11 populations.
The 1000 Genomes Project provides an extensive catalog of SNPs, structural variants and their haplotype context. Using the next generation sequencing technologies, this international collaboration will sequence genomes from approximately 2,500 individuals from about 25 populations around the world. The Phase I data, an integrated release of genetic variation from 1,092 human genomes is currently available.
Replication in Whole Genome SNP Studies
However, testing 500,000 or more independent SNPs at conventional levels of statistical significance will generate a very large number of “statistically significant” results. Consideration of other factors, such as whether an SNP is in a known candidate gene pathway or network or a candidate genomic region (identified for instance, by previous linkage analyses or cytogenetic abnormalities in tumors) might be useful in advancing SNPs of interest, but since so little is known about the majority of the genes in the genome, this exercise will only apply to a small portion of genic regions. Thus, while there is considerable novelty for the first whole genome scan conducted for a specific disease, the reality is that it does not do much more than identify a list of SNPs for further testing in follow-up replication studies.
Fortunately, simulations have shown that carefully designed multistage studies in large consortia in which the best candidate SNPs identified in the first stage are advanced in subsequent studies of comparable cases and controls maintain high statistical power and enable a substantial decrease in genotyping cost (328, 329).
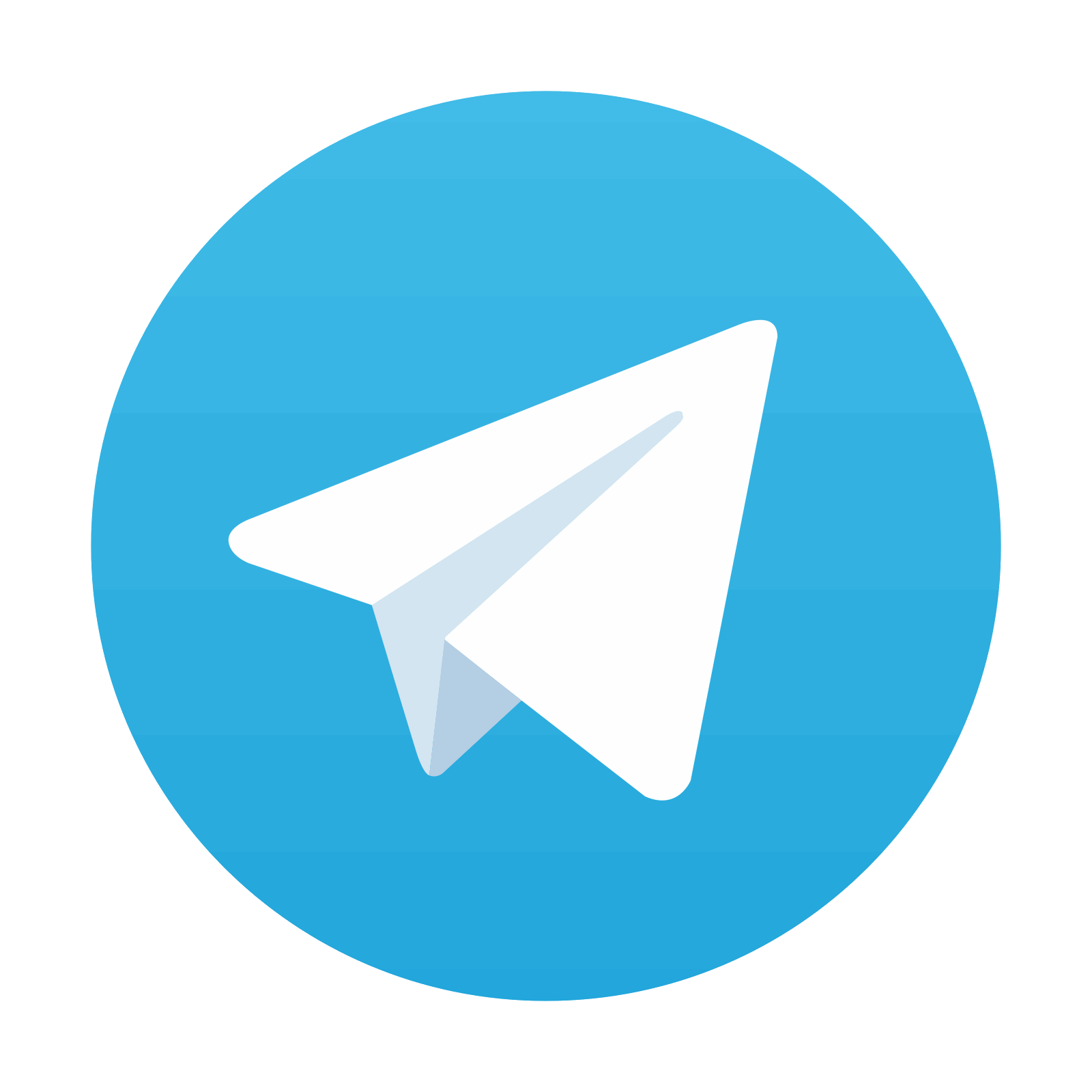
Stay updated, free articles. Join our Telegram channel

Full access? Get Clinical Tree
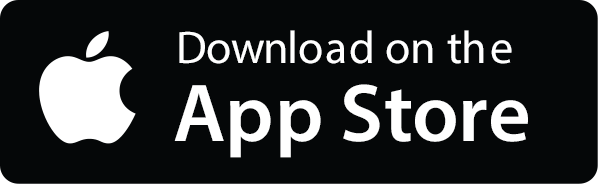
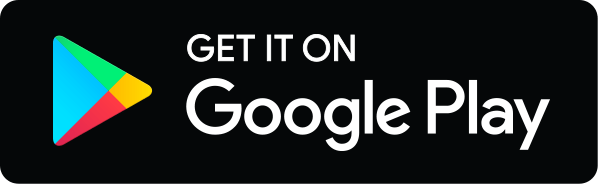