New Breast Imaging Techniques
Maxine S. Jochelson
More than ever before, the cost of medical care and concern for radiation exposure are being taken into account in the process of deciding which tests and treatments we recommend for our patients. These concerns certainly apply to breast imaging particularly because the examinations we perform are repeated regularly over the course of a woman’s lifetime contributing to cumulative radiation exposure. Additionally many of the newer technologies are costly; we need to learn which ones are worth the cost. Fortunately, there is a great deal of research and development in the field of breast imaging geared toward improved diagnostic capability while keeping cost and radiation exposure in mind. This chapter will review the current status of the current and more advanced breast imaging techniques.
Despite the seemingly never-ending controversies regarding its use, screening mammography remains the only breast imaging examination that reduces overall breast cancer mortality. This decrease in mortality is approximately 30%. Mammography is inexpensive and widely available. While there is radiation exposure, it is relatively low: The radiation dose has decreased from analog to digital mammography and is now 3.91 mGy to the breasts and 0.47 mSv to the whole body. The cost of mammography per life saved is less than that of seat belts, approximately $18,000.00.
The overall sensitivity of mammography is 70% to 85%. However, this sensitivity is dramatically decreased in women with denser breast tissue. As medicine becomes more personalized, screening recommendations are adjusted based on individual patient characteristics. Currently patient risk is arbitrarily divided into three categories. Average or normal risk is defined as less than 15% lifetime risk of developing breast cancer. Women at normal risk should begin screening mammography at the age of 40 and have yearly exams until their life expectancy is less than 5 years. Screening should begin earlier in women at intermediate (15% to 20%) risk and high risk (greater than 20%), generally 10 years earlier than the youngest family member who had breast cancer. If the increased risk is due to prior chest radiation, screening should begin 7 years after the completion of radiation therapy. With the application of these recommendations, there are many younger women having mammograms, and they generally have denser breast tissue. Sensitivity of mammography in these higher-risk women with dense breasts is only 30% to 50% (1, 2). Boyd et al. have shown that there is a 17.8-fold increase in interval cancers in women with extremely dense breasts compared with women with fatty breasts (3).
In addition to mammography, two other breast imaging tools are commonly used: ultrasound and breast MRI. Ultrasound has primarily been used to further evaluate clinical or mammographic findings and image-guided biopsies. It is inexpensive and widely available. Additionally there is no radiation exposure. Breast ultrasound is increasingly being used for screening in conjunction with screening mammography. However, it is time-consuming, operator dependent, and has a low positive predictive value (PPV). Ultrasound is not useful for the detection of DCIS and breast calcifications.
Breast MRI is an exquisitely sensitive method of breast evaluation with no radiation exposure. As opposed to mammography and breast ultrasound, which only evaluate anatomy, MRI combines physiologic with anatomic evaluation. Contrast-enhanced MRI enables detection of the neovascularity associated with breast cancer, sometimes before a discreet mass can be detected which is why it is so sensitive for detection of breast cancers and for staging cancers within the breast. It is also the most accurate method for following patients after neoadjuvant chemotherapy. However, MRI is very expensive and good-quality MRI is not universally
available. Claustrophobic patients and patients with certain implanted metallic clips and devices are unable to undergo breast MRI. Suboptimal specificity is also a limitation of breast MRI.
available. Claustrophobic patients and patients with certain implanted metallic clips and devices are unable to undergo breast MRI. Suboptimal specificity is also a limitation of breast MRI.
Clearly there is a need to develop better technologies for breast cancer detection. Two types of advances have been made. The first type of advancement builds on the standard technologies described above. These include the advance from analog mammography to digital mammography, and, based on the template of digital mammography: tomosynthesis and contrast-enhanced mammography. Advances in ultrasound include automated whole breast ultrasound (AWBU) and elastography. Microbubbles have been used as a contrast agent for ultrasound in various organs and have also been evaluated for breast imaging. MRI advances include improved interpretation criteria by the development of an MRI-specific BI-RADs system. Imaging sequences such as diffusionweighted imaging and spectroscopy may improve specificity.
The second type of advancement in breast imaging includes new platforms for imaging the breast. These include radionuclide breast imaging, CT of the breast, and optical breast imaging. Both types of advancements will be discussed in this chapter.
MAMMOGRAPHY
Xeromammography was the standard breast imaging modality until the early 1980s. Film screen (analog) mammography replaced xeromammography, leading to improvement in ability to detect soft-tissue lesions. Digital mammography has largely replaced film screen technique. In the United States approximately 70% of mammograms are performed with digital technique. Digital mammography has been shown to improve accuracy in breast cancer detection in women under 50, women with dense breast tissue, and premenopausal and perimenopausal women but has fallen short in improving overall accuracy (4). Radiation exposure is decreased and the interpreting radiologist is able to manipulate the images for better visualization. No hard-copy films are required. Moreover, the digital mammographic technology is the platform on which more advanced breast imaging techniques have been developed. These include digital breast tomosynthesis (DBT) and contrast-enhanced spectral mammography (CESM).
Tomosynthesis
Sometimes referred to as 3-D mammography, this technique was called the most exciting new technology in breast imaging in 2011. DBT is a purely anatomic evaluation of the breast. Multiple projections of the compressed breast are obtained using an x-ray tube that moves along an arc with a stationary detector. Image slices are reconstructed in the plane parallel to the detector both in a mediolateral oblique (MLO) or craniocaudal (CC) plane. A ā3-Dā volume can be reconstructed when both views are imaged. The theory behind this technique is that by performing these tomographic images, one can peel away the overlying breast tissue that may be obscuring lesions or their characteristics. This improves lesion conspicuity and margin feature analysis (Fig. 14-1). Additionally, false-positive lesions are seen on mammography due to superimposition of breast tissue and DBT can exclude an abnormality in this situation. Because of these capabilities, DBT can be more sensitive and specific than routine mammography. DBT produced by a single vendor is currently FDA approved to be performed in addition to a full-field digital mammogram (FFDM). Other vendors are applying for FDA approval.
DBT has been shown to reduce the number of patients called back for additional imaging 40% of the time (4, 5). This would be advantageous if DBT alone could be utilized for screening. Used in addition to a screening mammogram, it is essentially a callback of 100% of the patients merely performed at the same time. Noroozian et al. demonstrated that DBT images showed equivalent mass characterization when compared to routine spot films. In 67 patients, DBT detected 7 additional cancers as well as 5 additional falsepositive findings (6). Rafferty et al. compared FFDM alone to FFDM plus DBT in two multi-institutional reader studies of 1,192 subjects. Diagnostic accuracy for the combination of tomosynthesis with DBT showed a statistically significant improvement of 6.8% and 7.2% in the two studies (7). Of interest, in a test set of 130 women, two-view tomosynthesis had significantly better accuracy than FFDM but only for readers with the least experience in mammographic interpretation (8).
The benefits of DBT are seen only with soft-tissue lesions. Identification and characterization of calcifications is more problematic. This is partially due to the very thin slices (1 mm) and the blurring inherent to tomosynthesis. Spangler et al. showed superior sensitivity (84% vs. 75%) and specificity (71% vs. 64%) of FFDM over DBT (9). Technological improvements have been made to improve on this, but this remains an area where DBT does not currently offer an advantage over FFDM.
There are many issues to be resolved before any official recommendations can be made for use of DBT. The radiation dose of tomosynthesis is 4 to 8 mGy so that doing both routine mammography and DBT exams doubles or triples overall dose. In an effort to keep radiation dose to a minimum, studies have been and are being performed using various combinations of the two exams: FFDM with a single-view (MLO) tomosynthesis examination was performed in 200 women (376 breasts evaluated). The clinical performance of a singleview tomo was not significantly different from routine mammography (10). Other options being considered include a single-view tomo (i.e., MLO) with the other view (CC) digital mammogram. Another option would be to replace the digital mammogram with a mammogram synthesized from the tomosynthesis examination. Additional research is required to determine the approach with the least radiation and the highest yield.
DBT takes 50% to 70% longer to read than routine mammography which is clearly a significant limitation. Reimbursement does not reflect the additional time expenditure. Storage and display issues must also be resolved. Additionally, it is unclear whether DBT is best used as a screening tool in all patients, screening in selected patients, or as a diagnostic tool after an abnormal screening mammogram. A planned American College of Radiology Imaging Network (ACRIN) trial will address this question. However, it is clear that it will be decades before we are able to determine if screening with DBT will provide reduction of mortality from breast cancer over and above that of routine mammographic screening.
Contrast-Enhanced Mammography
Breast MRI is the most sensitive technique for breast cancer imaging with sensitivities reported to be nearly 100%. The excellent sensitivity is due to its ability to detect abnormal blood flow in a breast cancer in addition to identifying the mass itself. MRI may show vascular enhancement in tumor vascularity even when no discrete mass can be identified: an entity termed nonmass enhancement. With the advent of digital mammography, it was hypothesized that the addition of intravenous contrast to digital mammography could potentially approximate this capability of MRI. Contrastenhanced mammography would therefore combine physiology with anatomy.
Initial studies were performed using temporal subtraction technique and iodinated contrast material. Patients were injected following a baseline image while the breast was compressed and up to seven additional images were obtained after injection. Subtraction was performed yielding both kinetic curves and a contrast enhanced image. Although this technique was able to detect enhancement associated with breast carcinoma in most patients, there were technical limitations. Motion artifact due to long imaging times limited the quality of the images. Additionally only a single view of one breast could be imaged per injection.
An alternative solution utilizing dual-energy imaging was proposed. Hardware and software adaptations to a digital mammography unit automate the dual-energy technique. Approximately 3 minutes after the injection of iodinated contrast material a mammogram is performed. For each exposure, two images are obtained in each view: a low-energy image that is below the K-edge of iodine (33.2 keV) and a high-energy image that is just above. The two images are combined and processed so that the background breast tissue is subtracted out, maximizing the ability to see the iodine enhancement. The low-energy image is essentially a digital mammogram, although at the moment it cannot be used as such. This procedure was initially called dual-energy contrastenhanced digital mammography (DE CEDM) and is now called contrast-enhanced spectral mammography (CESM). Using this technology, Dromain et al. compared contrast-enhanced mammography plus noncontrast mammography to mammography alone or to mammography with ultrasound in the evaluation of 142 lesions in a single breast in 120 patients. Sensitivity for contrast-enhanced mammography with mammography was 93% versus 78% for mammography alone (p < .001). Specificity was unchanged. There was a trend toward improvement in sensitivity and specificity when DE CEDM plus mammography was compared to ultrasound with mammography, but this did not reach statistical significance (11).
Jochelson et al. then evaluated the feasibility of performing bilateral contrast-enhanced mammography in 10 patients with known carcinoma using the same technique described above. Bilateral DE CEDM was easily accomplished and well tolerated. The order in which the images were obtained did not matter. The bilateral examination was completed within 8 to 10 minutes. While contrast enhancement in MRI washes out quickly, contrast enhancement with DE CEDM remained for up to 10 minutes after injection. It is presumed that this may be due to the use of a different type of contrast material. Radiation dose from this dual-energy technique is 20% more than a routine digital mammogram or the equivalent of one extra mammographic image.
Once feasibility was demonstrated, DE CEDM was compared to breast MRI and digital mammography for the ability to identify the primary tumor in 52 patients with untreated unilateral breast cancer. Contrast-enhanced mammography was also compared to MRI for its ability to stage the cancer within the breast. DE CEDM and breast MRI were equivalent in their ability to detect the index tumors: 50 per 52 (96%) and significantly better than mammography 42 per 52 (81%) at p < .05 (Fig. 14-2). The lesions detected by DE CEDM ranged from 4 to 67 mm, median 17 mm. The size of the lesions approximated pathologic size in all but two patients in whom DE CEDM overestimated the size of the lesions. The two cancers not seen on DE CEDM included a 2-cm infiltrating lobular carcinoma (ILC) and a 5-mm infiltrating ductal carcinoma with ductal carcinoma in situ (IDC and DCIS). The two lesions
occult on MRI, which were in patients whose cancers were detected on DE CEDM, included a 7-mm IDC/DCIS and an area of DCIS that measured 14 mm on DE CEDM. The only contralateral cancer was Paget’s disease which was not evident on either DE CEDM or MRI, but was detected when the patient underwent a prophylactic contralateral mastectomy.
occult on MRI, which were in patients whose cancers were detected on DE CEDM, included a 7-mm IDC/DCIS and an area of DCIS that measured 14 mm on DE CEDM. The only contralateral cancer was Paget’s disease which was not evident on either DE CEDM or MRI, but was detected when the patient underwent a prophylactic contralateral mastectomy.
MRI surpassed DE CEDM in the ability to detect additional sites of malignancy. Sixteen patients had multifocal or multicentric cancers and MRI detected 15 per 16 (94%) while DE CEDM detected additional disease in 9 per 16 (56%). Twenty-five additional lesions were detected in these 16 patients. MRI detected 22 per 25(92%) and DE CEDM 14 per 25 (56%). The clinical impact of this difference in detection of additional lesions was seen in three patients who were originally thought to be candidates for breast conservation, but were demonstrated to have multicentric disease thus requiring mastectomy. The addition of DE CEDM or MRI to digital mammography conveyed a considerable advantage in the determination of which women required mastectomy.
One of the greatest limitations of MRI is its low specificity. DE CEDM was more specific than MRI in this series: 4% of patients undergoing DE CEDM had one false-positive finding while 25% of patients undergoing MRI had one falsepositive finding. Two patients demonstrated false-positive lesions in the ipsilateral breast on DE CEDM, and biopsy of both of these lesions was also recommended on the corresponding MRI. On pathology, one was a radial scar and the other a fibroadenoma. No contralateral false-positive lesions were found on DE CEDM. There were 13 false-positive findings on MRI: 8 in the ipsilateral breast and 5 in the contralateral breast. Eight core biopsies and 8 additional surgical procedures were performed as a result of these false-positive findings on MRI. Biopsies yielded the following: 1 radial scar, 2 fibroadenomas, 2 papillary lesions, 3 cases of ADH, 1 ALH, and 4 cases of benign tissue. None of the high-grade lesions were upgraded at surgery.
An enhancing lesion seen on DE CEDM was significantly more likely to be malignant than one seen on MRI, with a positive predictive value for DE CEDM of 97% (64 per 66) and for MRI of 85% (72 per 85) (p < .01) (12).
Since the initial trials, improvements to the software, hardware, and filters of the CEDM unit have been made. It is now FDA approved and called contrast-enhanced spectral mammography (CESM) because there is the potential to use more than two energies. With these changes, there is less time needed between exposures enabling a shorter examination time and less chance for motion. The processing mechanism has also been improved yielding better images. It remains to be seen if the improved technique will improve accuracy. At this time the ability of CESM to detect cancers in a pure screening setting has not been tested, but that study is underway.
Contrast-Enhanced Digital Breast Tomosynthesis
The natural next step from the latter two technologies is to combine them. Chen et al. performed a pilot study involving 13 patients with BI-RADS 4 or 5 lesions using tomosynthesis performed in the MLO projection and temporal subtraction technique (13). Ten of 11 cancers were detected. Neither of the two benign lesions enhanced. More recently, Carton et al. (14)
performed both temporal subtraction and dual-energy contrast enhancement with DBT on a single breast with a known cancer. The cancer was identified with both contrast techniques and was found to correlate with MRI images in that same patient. Not surprisingly there was less motion artifact when using dual-energy technique when compared to temporal subtraction. Dual energy also allowed for both breasts to be imaged. As this chapter is being written there is ongoing development of this promising technology.
performed both temporal subtraction and dual-energy contrast enhancement with DBT on a single breast with a known cancer. The cancer was identified with both contrast techniques and was found to correlate with MRI images in that same patient. Not surprisingly there was less motion artifact when using dual-energy technique when compared to temporal subtraction. Dual energy also allowed for both breasts to be imaged. As this chapter is being written there is ongoing development of this promising technology.
ULTRASOUND
Targeted breast ultrasound is a standard method of evaluating mammographic and clinical breast abnormalities. It is used to characterize a mass seen on mammography as cystic or solid and may distinguish malignant from benign features. Ultrasound is also used to investigate a mammographically occult palpable mass and to guide core biopsies.
Screening ultrasound has gained increasing popularity as an adjunct to screening mammography, particularly in women at increased risk for breast cancer. It is a method of cancer detection that is predominantly anatomic. Ultrasound is relatively inexpensive and widely available. There is no radiation exposure. Since ultrasound is operator dependent, reproducibility is suboptimal.
ACRIN trial 6666 evaluated the performance of screening breast ultrasound in addition to mammography in over 6,000 women who had dense breast tissue in at least one quadrant of the breast and at least one other risk factor. Interpretation was blinded to mammographic findings. There were 4.2 mammographically occult cancers detected per 1,000 women. These were primarily invasive cancers. Ultrasound was not shown to be useful for the detection of DCIS or microcalcifications. In this study, biopsy was recommended in 9% of the women and short-term follow-up was recommended in another 9%. PPV was only 9% (15).
In the last few years many states have passed legislation requiring patients to be directly informed that they have dense breasts with an associated increased risk for breast cancer. Additional imaging will likely be suggested or desired by the patient and currently this has primarily been screening ultrasound. The first state to enforce this legislation was Connecticut. Weigert et al. have reported the first data regarding the use of screening ultrasound in women with dense breast tissue and no other risk factors. In this retrospective study of six practices there were 72,030 mammograms and 8,647 screening ultrasounds. Twenty-eight cancers were diagnosed with an additional cancer detection rate of 3.25 per 1,000. PPV was only 6.7%, 9% of patients were called BI-RADS 3 requiring 6-month follow-up. 5% were BI-RADS 4 or 5. In their population the average cost of a breast ultrasound was $250 for which average insurance reimbursement was $72. Professional fee was $85 and reimbursement was $30. Ultrasound core biopsies were $2,400. Using these numbers the cost per breast cancer found was $110,241 (16).
Automated Whole Breast Ultrasound (AWBU)
AWBU is a technique developed to decrease the operator dependency of handheld ultrasound and thereby improve reproducibility. The device is placed over the breast and static images are obtained in a standard fashion which also allows 3-D reconstruction. The static images do not need to be interpreted in real time. This may improve efficiency, but patients will need to be called back if there are findings requiring additional evaluation. Kelly et al. reported their experience with the performance of AWBU with mammography in 6,425 studies in women with dense breasts. They demonstrated an improvement in cancer detection from 3.6 per 1,000 with mammography alone to 7.2 per 1,000 by the addition of AWBU. In their hands the PPV of AWBU was 38.4% versus 39% for mammography. Twenty-one cancers less than 10 mm were detected by ultrasound versus 7 by mammography (17). Shin et al. evaluated 55 patients with 121 lesions detected with handheld ultrasound. An additional 36 lesions were detected with AWBU. Lesion detection rate increased with size. It was 92% when mean lesion diameter was greater than 1.2 cm. Their false-positive detection rate was 6% (18). Should these data be confirmed in larger studies, this technology could potentially provide improved screening results with a more efficient method of imaging.
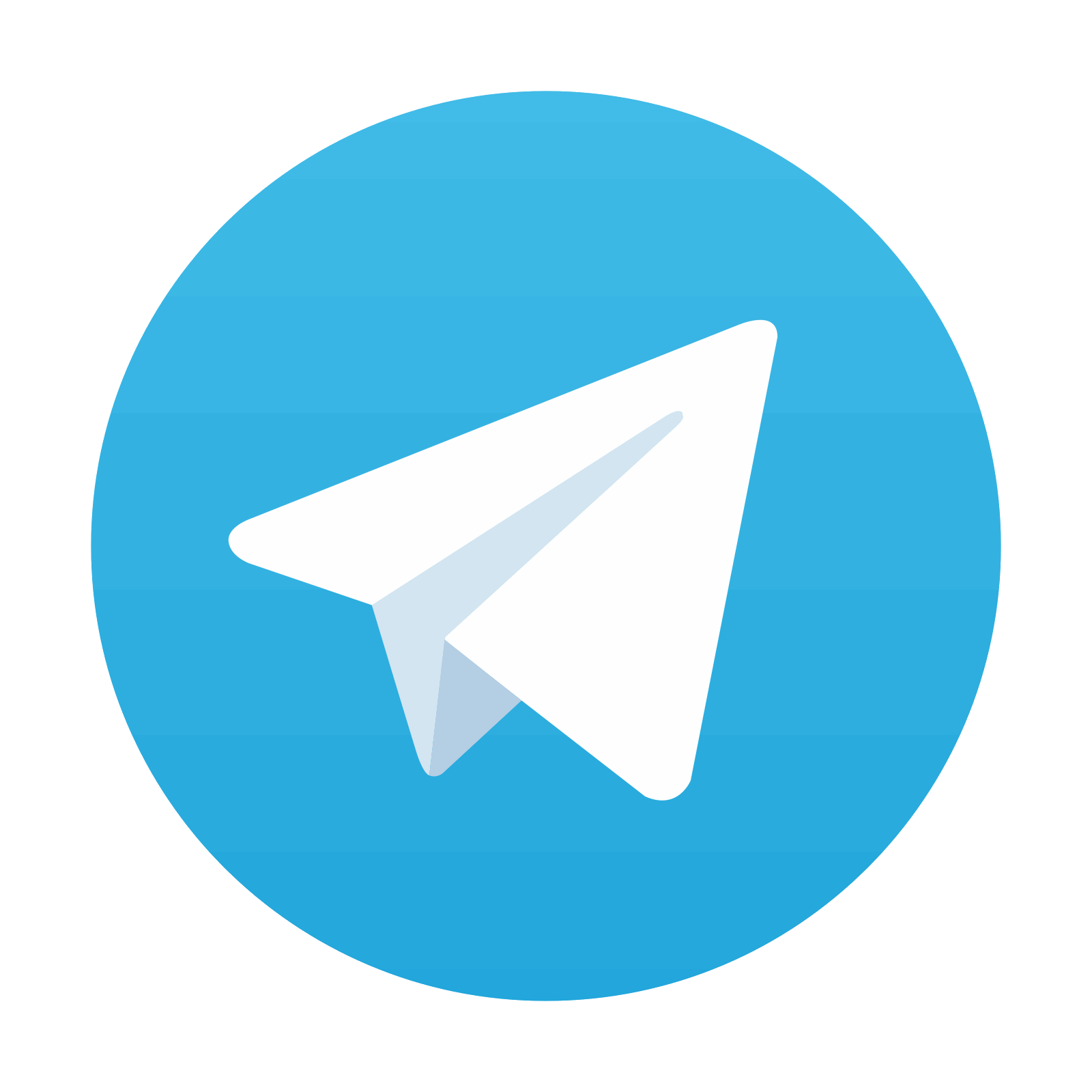
Stay updated, free articles. Join our Telegram channel

Full access? Get Clinical Tree
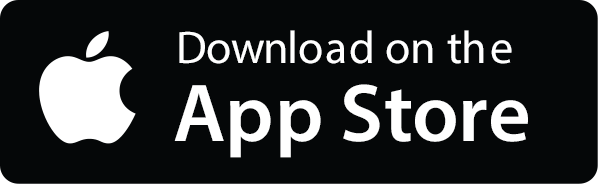
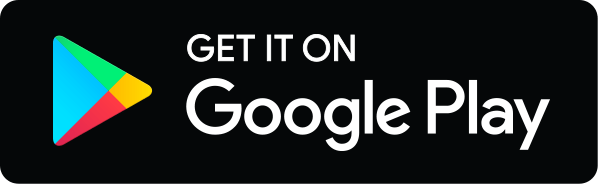