Epigenetics and Drug Resistance
Tumor Stroma, Cell-to-Cell Interactions, and Drug Resistance
Drug Efflux Transporters
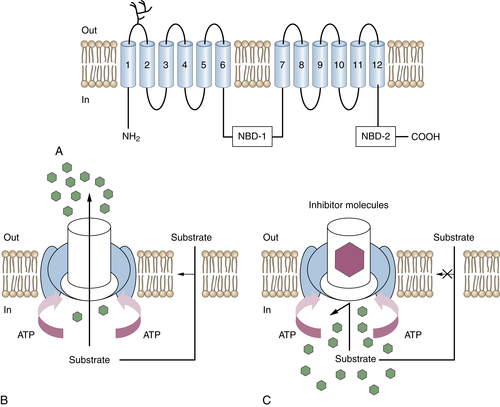
Impaired Drug Uptake
Mutation or Altered Expression of Molecular Targets
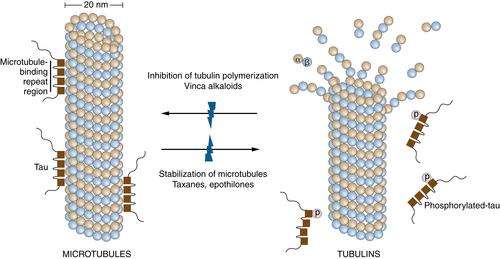
Intracellular Redistribution of Drug
Detoxification of Drug or Intermediate Drug Product
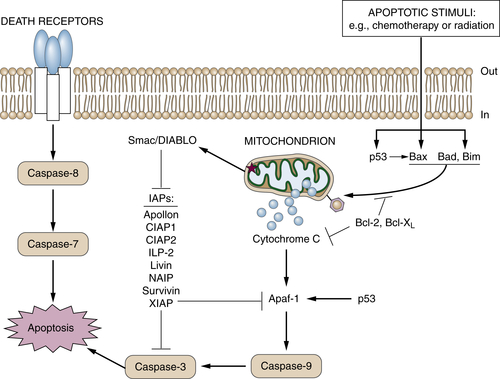
Enhanced DNA Repair
Decreased Drug Activation
Altered Pathways for Programmed Cell Death (Apoptosis)
Individualization of Therapy Based on Predictive Multigenic Markers
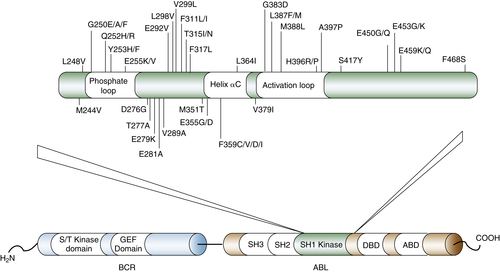
1. Continuous infusion or bolus injection in cancer chemotherapy . Ann Intern Med . 1983 ; 99 : 823 – 833 .
2. Chemotherapy administration: doses, infusions and choice of schedule . Ann Oncol . 1994 ; 5 ( suppl 4 ) : 25 – 29 discussion 29–30 .
3. Dose and outcome: the hurdle of neutropenia (Review) . Oncol Rep . 2006 ; 16 : 233 – 248 .
4. Multidrug-resistance gene (P-glycoprotein) is expressed by endothelial cells at blood-brain barrier sites . Proc Natl Acad Sci U S A . 1989 ; 86 : 695 – 698 .
5. Disruption of the mouse mdr1a P-glycoprotein gene leads to a deficiency in the blood-brain barrier and to increased sensitivity to drugs . Cell . 1994 ; 77 : 491 – 502 .
6. Limited tissue penetration of taxanes: a mechanism for resistance in solid tumors . Clin Cancer Res . 2007 ; 13 : 2804 – 2810 .
7. Drug penetration in solid tumours . Nat Rev Cancer . 2006 ; 6 : 583 – 592 .
8. Antiangiogenic agent sunitinib transiently increases tumor oxygenation and suppresses cycling hypoxia . Cancer Res . 2011 ; 71 : 6350 – 6359 .
9. Mechanisms of cancer drug resistance . Annu Rev Med . 2002 ; 53 : 615 – 627 .
10. Identification of molecular determinants of tumor sensitivity and resistance to anticancer drugs . Adv Exp Med Biol . 2007 ; 593 : 95 – 104 .
11. Tumour stem cells and drug resistance . Nat Rev Cancer . 2005 ; 5 : 275 – 284 .
12. Thymocyte apoptosis induced by p53-dependent and independent pathways . Nature . 1993 ; 362 : 849 – 852 .
13. bcl-2 modulation of apoptosis induced by anticancer drugs: resistance to thymidylate stress is independent of classical resistance pathways . Cancer Res . 1993 ; 53 : 3321 – 3326 .
14. bcl-2 gene transfer increases relative resistance of S49.1 and WEHI7.2 lymphoid cells to cell death and DNA fragmentation induced by glucocorticoids and multiple chemotherapeutic drugs . Cancer Res . 1992 ; 52 : 5407 – 5411 .
15. p53 gene mutations are associated with decreased sensitivity of human lymphoma cells to DNA damaging agents . Cancer Res . 1994 ; 54 : 5824 – 5830 .
16. Bcl-2 family proteins: regulators of apoptosis and chemoresistance in hematologic malignancies . Semin Hematol . 1997 ; 34 : 9 – 19 .
17. The role of the bcl-2/ced-9 gene family in cancer and general implications of defects in cell death control for tumourigenesis and resistance to chemotherapy . Biochim Biophys Acta . 1997 ; 1333 : F151 – F178 .
18. Apoptosis and therapy . J Pathol . 1999 ; 187 : 127 – 137 .
19. Apoptosis and anticancer drug resistance . Hum Cell . 2001 ; 14 : 211 – 221 .
20. Apoptosis: a link between cancer genetics and chemotherapy . Cell . 2002 ; 108 : 153 – 164 .
21. Extrinsic versus intrinsic apoptosis pathways in anticancer chemotherapy . Oncogene . 2006 ; 25 : 4798 – 4811 .
22. p53 is required for radiation-induced apoptosis in mouse thymocytes . Nature . 1993 ; 362 : 847 – 849 .
23. p53-dependent apoptosis modulates the cytotoxicity of anticancer agents . Cell . 1993 ; 74 : 957 – 967 .
24. bcl-x, a bcl-2-related gene that functions as a dominant regulator of apoptotic cell death . Cell . 1993 ; 74 : 597 – 608 .
25. Bcl-2 oncoprotein blocks chemotherapy-induced apoptosis in a human leukemia cell line . Blood . 1993 ; 81 : 151 – 157 .
26. Drug resistance and the microenvironment: nature and nurture . Drug Resist Updat . 2003 ; 6 : 169 – 172 .
27. Multiple paths to a drug resistance phenotype: mutations, translocations, deletions and amplification of coding genes or promoter regions, epigenetic changes and microRNAs . Drug Resist Updat . 2007 ; 10 : 59 – 67 .
28. The clonal evolution of tumor cell populations . Science . 1976 ; 194 : 23 – 28 .
29. Clonal evolution in cancer . Nature . 2012 ; 481 : 306 – 313 .
30. A mathematic model for relating the drug sensitivity of tumors to their spontaneous mutation rate . Cancer Treat Rep . 1979 ; 63 : 1727 – 1733 .
31. Genetic instability in the development of drug resistance . Semin Oncol . 1985 ; 12 : 2222 – 2230 .
32. The genetic basis of resistance to cancer chemotherapy . Ann Med . 1995 ; 27 : 157 – 167 .
33. Mutation rates and mechanisms of resistance to etoposide determined from fluctuation analysis . J Natl Cancer Inst . 1994 ; 86 : 1152 – 1158 .
34. Prevalence of multidrug resistance related to activation of the mdr1 gene in human sarcoma mutants derived by single-step doxorubicin selection . Cancer Res . 1994 ; 54 : 4980 – 4987 .
35. Decreased mutation rate for cellular resistance to doxorubicin and suppression of mdr1 gene activation by the cyclosporin PSC 833 . J Natl Cancer Inst . 1995 ; 87 : 1593 – 1602 .
36. Resistance mechanisms in human sarcoma mutants derived by single-step exposure to paclitaxel (Taxol) . Cancer Res . 1996 ; 56 : 1091 – 1097 .
37. Cellular adaptation to drug exposure: evolution of the drug-resistant phenotype . Cancer Res . 1997 ; 57 : 5086 – 5092 .
38. Genetic and epigenetic modeling of the origins of multidrug-resistant cells in a human sarcoma cell line . Cancer Res . 2005 ; 65 : 9388 – 9397 .
39. Intratumor heterogeneity and branched evolution revealed by multiregion sequencing . N Engl J Med . 2012 ; 366 : 883 – 892 .
40. Tumour heterogeneity and drug resistance: personalising cancer medicine through functional genomics . Biochem Pharmacol . 2012 ; 83 : 1013 – 1020 .
41. Intratumor heterogeneity: evolution through space and time . Cancer Res . 2012 ; 72 : 4875 – 4882 .
42. Selective multiplication of dihydrofolate reductase genes in methotrexate-resistant variants of cultured murine cells . J Biol Chem . 1978 ; 253 : 1357 – 1370 .
43. Regional activation of chromosomal arm 7q with and without gene amplification in taxane-selected human ovarian cancer cell lines . Genes Chromosomes Cancer . 2006 ; 45 : 365 – 374 .
44. Epigenetic resensitization to platinum in ovarian cancer . Cancer Res . 2012 ; 72 : 2197 – 2205 .
45. Overexpression of P-glycoprotein in mammalian tumor cell lines after fractionated X irradiation in vitro . J Natl Cancer Inst . 1990 ; 82 : 607 – 612 .
46. Induction of drug resistance and protein kinase C genes in A2780 ovarian cancer cells after incubation with antineoplastic agents at sublethal concentrations . Anticancer Res . 2002 ; 22 : 4229 – 4232 .
47. Rapid activation of MDR1 gene expression in human metastatic sarcoma after in vivo exposure to doxorubicin . Clin Cancer Res . 1999 ; 5 : 3352 – 3356 .
48. Epigenetic mechanisms in tumorigenesis, tumor cell heterogeneity and drug resistance . Drug Resist Updat . 2012 ; 15 : 21 – 38 .
49. Epigenetic targeting therapies to overcome chemotherapy resistance . Adv Exp Med Biol . 2013 ; 754 : 285 – 311 .
50. Candidate DNA methylation drivers of acquired cisplatin resistance in ovarian cancer identified by methylome and expression profiling . Oncogene . 2012 ; 31 : 4567 – 4576 .
51. Cisplatin resistance: a cellular self-defense mechanism resulting from multiple epigenetic and genetic changes . Pharmacol Rev . 2012 ; 64 : 706 – 721 .
52. Epigenetic reprogramming reverses the relapse-specific gene expression signature and restores chemosensitivity in childhood B-lymphoblastic leukemia . Blood . 2012 ; 119 : 5201 – 5210 .
53. Molecular pathways: regulation and therapeutic implications of multidrug resistance . Clin Cancer Res . 2012 ; 18 : 1863 – 1869 .
54. Acquired resistance to drugs targeting receptor tyrosine kinases . Biochem Pharmacol . 2012 ; 83 : 1041 – 1048 .
55. A chromatin-mediated reversible drug-tolerant state in cancer cell subpopulations . Cell . 2010 ; 141 : 69 – 80 .
56. The role of the miR-200 family in epithelial-mesenchymal transition . Cancer Biol Ther . 2010 ; 10 : 219 – 222 .
57. Resistance to chemotherapy: short-term drug tolerance and stem cell-like subpopulations . Adv Pharmacol . 2012 ; 65 : 315 – 334 .
58. Ovarian carcinoma tumor-initiating cells have a mesenchymal phenotype . Cell Cycle . 2012 ; 11 : 1966 – 1976 .
59. Breast cancer stem cells: Obstacles to therapy . Cancer Lett . 2012 Apr 30 [Epub ahead of print] .
60. Cancer stem cells and chemosensitivity . Clin Cancer Res . 2011 ; 17 : 4942 – 4947 .
61. The tumor stroma as mediator of drug resistance—a potential target to improve cancer therapy? Curr Pharm Biotechnol . 2012 ; 13 : 2259 – 2272 .
62. Molecular pathways: involving microenvironment damage responses in cancer therapy resistance . Clin Cancer Res . 2012 ; 18 : 4019 – 4025 .
63. Adhesion-mediated apoptosis resistance in cancer . Drug Resist Updat . 2009 ; 12 : 127 – 136 .
64. Fibroblasts contribute to melanoma tumor growth and drug resistance . Mol Pharm . 2011 ; 8 : 2039 – 2049 .
65. Overview: ABC transporters and human disease . J Bioenerg Biomembr . 2001 ; 33 : 453 – 458 .
66. Pharmacogenetics/genomics of membrane transporters in cancer chemotherapy . Cancer Metastasis Rev . 2007 ; 26 : 183 – 201 .
67. The role of ABC transporters in drug resistance, metabolism and toxicity . Curr Drug Deliv . 2004 ; 1 : 27 – 42 .
68. MRP subfamily transporters and resistance to anticancer agents . J Bioenerg Biomembr . 2001 ; 33 : 493 – 501 .
69. Genetic and biochemical characterization of multidrug resistance . Pharmacol Ther . 1985 ; 28 : 51 – 75 .
70. P-glycoprotein: from genomics to mechanism . Oncogene . 2003 ; 22 : 7468 – 7485 .
71. Vinblastine photoaffinity labeling of a high molecular weight surface membrane glycoprotein specific for multidrug-resistant cells . J Biol Chem . 1986 ; 261 : 6137 – 6140 .
72. Expression of a full-length cDNA for the human “MDR1” gene confers resistance to colchicine, doxorubicin, and vinblastine . Proc Natl Acad Sci U S A . 1987 ; 84 : 3004 – 3008 .
73. Cellular localization of the multidrug-resistance gene product P-glycoprotein in normal human tissues . Proc Natl Acad Sci U S A . 1987 ; 84 : 7735 – 7738 .
74. Tyrosine kinase inhibitors as modulators of ABC transporter-mediated drug resistance . Drug Resist Updat . 2012 ; 15 : 70 – 80 .
75. Drug-resistance in multiple myeloma and non-Hodgkin’s lymphoma: detection of P-glycoprotein and potential circumvention by addition of verapamil to chemotherapy . J Clin Oncol . 1989 ; 7 : 415 – 424 .
76. Clinical significance of multidrug resistance P-glycoprotein expression on acute nonlymphoblastic leukemia cells at diagnosis . Blood . 1992 ; 79 : 473 – 476 .
77. P-glycoprotein expression in malignant lymphoma and reversal of clinical drug resistance with chemotherapy plus high-dose verapamil . J Clin Oncol . 1991 ; 9 : 17 – 24 .
78. Multidrug resistance (mdr1) gene expression in adult acute leukemias: correlations with treatment outcome and in vitro drug sensitivity . Blood . 1991 ; 78 : 586 – 592 .
79. Clinical relevance of transmembrane drug efflux as a mechanism of multidrug resistance . J Clin Oncol . 1998 ; 16 : 3674 – 3690 .
80. Targeting the multidrug resistance-1 transporter in AML: molecular regulation and therapeutic strategies . Blood . 2004 ; 104 : 1940 – 1951 .
81. Multidrug resistance/P-glycoprotein and breast cancer: review and meta-analysis . Semin Oncol . 2005 ; 32 : S9 – S15 .
82. Modulation of multidrug resistance: at the threshold . J Clin Oncol . 1993 ; 11 : 1629 – 1635 .
83. Clinical studies with modulators of multidrug resistance . Hematol Oncol Clin North Am . 1995 ; 9 : 363 – 382 .
84. Pharmacologic approaches to reversing multidrug resistance . Semin Hematol . 1997 ; 34 : 40 – 47 .
85. Alteration of etoposide pharmacokinetics and pharmacodynamics by cyclosporine in a phase I trial to modulate multidrug resistance . J Clin Oncol . 1992 ; 10 : 1635 – 1642 .
86. Effect of high-dose cyclosporine on etoposide pharmacodynamics in a trial to reverse P-glycoprotein (MDR1 gene) mediated drug resistance . Cancer Chemother Pharmacol . 2000 ; 45 : 305 – 311 .
87. A phase I trial of doxorubicin, paclitaxel, and valspodar (PSC 833), a modulator of multidrug resistance . Clin Cancer Res . 2001 ; 7 : 1221 – 1229 .
88. Benefit of cyclosporine modulation of drug resistance in patients with poor-risk acute myeloid leukemia: a Southwest Oncology. Group study . Blood . 2001 ; 98 : 3212 – 3220 .
89. Reversal of multidrug resistance by the P-glycoprotein modulator, LY335979, from the bench to the clinic . Curr Med Chem . 2001 ; 8 : 39 – 50 .
90. Biology of the multidrug resistance-associated protein, MRP . Eur J Cancer . 1996 ; 32A : 945 – 957 .
91. Overexpression of the gene encoding the multidrug resistance-associated protein results in increased ATP-dependent glutathione S-conjugate transport . Proc Natl Acad Sci U S A . 1994 ; 91 : 13033 – 13037 .
92. ATP-dependent transport of bilirubin glucuronides by the multidrug resistance protein MRP1 and its hepatocyte canalicular isoform MRP2 . Biochem J. 327 part . 1997 ; 1 : 305 – 310 .
93. MRP3, an organic anion transporter able to transport anti-cancer drugs . Proc Natl Acad Sci U S A . 1999 ; 96 : 6914 – 6919 .
94. Characterization of vincristine transport by the M(r) 190,000 multidrug resistance protein (MRP): evidence for cotransport with reduced glutathione . Cancer Res . 1998 ; 58 : 5130 – 5136 .
95. Transport of glutathione conjugates and glucuronides by the multidrug resistance proteins MRP1 and MRP2 . Biol Chem . 1997 ; 378 : 787 – 791 .
96. The MRP gene encodes an ATP-dependent export pump for leukotriene C4 and structurally related conjugates . J Biol Chem . 1994 ; 269 : 27807 – 27810 .
97. Coordinated action of glutathione S-transferases (GSTs) and multidrug resistance protein 1 (MRP1) in antineoplastic drug detoxification. Mechanism of GST A1-1- and MRP1-associated resistance to chlorambucil in MCF7 breast carcinoma cells . J Biol Chem . 1998 ; 273 : 20114 – 20120 .
98. ABC transporters and their role in nucleoside and nucleotide drug resistance . Biochem Pharmacol . 2012 ; 83 : 1073 – 1083 .
99. Atypical multidrug resistance: breast cancer resistance protein messenger RNA expression in mitoxantrone-selected cell lines . J Natl Cancer Inst . 1999 ; 91 : 429 – 433 .
100. The role of half-transporters in multidrug resistance . J Bioenerg Biomembr . 2001 ; 33 : 503 – 511 .
101. Breast cancer resistance protein and P-glycoprotein in 149 adult acute myeloid leukemias . Clin Cancer Res . 2004 ; 10 : 7896 – 7902 .
102. MRP3, BCRP, and P-glycoprotein activities are prognostic factors in adult acute myeloid leukemia . Clin Cancer Res . 2005 ; 11 : 7764 – 7772 .
103. Breast cancer resistance protein (BCRP) in acute leukemia . Leuk Lymphoma . 2004 ; 45 : 649 – 654 .
104. Breast cancer resistance protein in drug resistance of primitive CD34+38- cells in acute myeloid leukemia . Clin Cancer Res . 2005 ; 11 : 2436 – 2444 .
105. Multidrug resistance and stem cells in acute myeloid leukemia . Clin Cancer Res . 2006 ; 12 : 3231 – 3232 .
106. ABCB1 gene polymorphisms are not associated with treatment outcome in elderly acute myeloid leukemia patients . Clin Pharmacol Ther . 2006 ; 80 : 427 – 439 .
107. Copper transporters regulate the cellular pharmacology and sensitivity to Pt drugs . Crit Rev Oncol Hematol . 2005 ; 53 : 13 – 23 .
108. Cellular processing of platinum anticancer drugs . Nat Rev Drug Discov . 2005 ; 4 : 307 – 320 .
109. Methotrexate transport and resistance . Leuk Lymphoma . 1998 ; 30 : 215 – 224 .
110. Cellular and molecular determinants of cisplatin resistance . Eur J Cancer . 1998 ; 34 : 1535 – 1542 .
111. Unstable amplification of an altered dihydrofolate reductase gene associated with double-minute chromosomes . Cell . 1981 ; 26 : 355 – 362 .
112. Mechanisms of action of and resistance to antitubulin agents: microtubule dynamics, drug transport, and cell death . J Clin Oncol . 1999 ; 17 : 1061 – 1070 .
113. Mechanisms of taxol resistance related to microtubules . Oncogene . 2003 ; 22 : 7280 – 7295 .
114. Class III beta-tubulin expression in tumor cells predicts response and outcome in patients with non-small cell lung cancer receiving paclitaxel . Mol Cancer Ther . 2005 ; 4 : 2001 – 2007 .
115. Paclitaxel resistance: molecular mechanisms and pharmacologic manipulation . Curr Cancer Drug Targets . 2003 ; 3 : 1 – 19 .
116. Conservation of the class I beta tubulin gene in human populations and lack of mutations in lung cancers and paclitaxel resistant ovarian cancers . Mol Cancer Ther . 2002 ; 1 : 215 – 225 .
117. No significant role for beta tubulin mutations and mismatch repair defects in ovarian cancer resistance to paclitaxel/cisplatin . BMC Cancer . 2005 ; 5 : 101 – 106 .
118. Microtubule-associated protein tau: a marker of paclitaxel sensitivity in breast cancer . Proc Natl Acad Sci U S A . 2005 ; 102 : 8315 – 8320 .
119. Microtubule Associated Protein (MAP)-Tau: a novel mediator of paclitaxel sensitivity in vitro and in vivo . Cell Cycle . 2005 ; 4 : 1149 – 1152 .
120. Dependence of paclitaxel sensitivity on a functional spindle assembly checkpoint . Cancer Res . 2004 ; 64 : 2502 – 2508 .
121. Mechanisms of paclitaxel-induced apoptosis in an ovarian cancer cell line and its paclitaxel-resistant clone . Oncology . 2004 ; 66 : 53 – 61 .
122. Epothilones: mechanism of action and biologic activity . J Clin Oncol . 2004 ; 22 : 2015 – 2025 .
123. Development of novel chemotherapeutic agents to evade the mechanisms of multidrug resistance (MDR) . Semin Oncol . 2005 ; 32 : S22 – S26 .
124. Characterization of a mammalian mutant with a camptothecin-resistant DNA topoisomerase I . Proc Natl Acad Sci U S A . 1987 ; 84 : 5565 – 5569 .
125. Direct correlation between DNA topoisomerase II activity and cytotoxicity in adriamycin-sensitive and -resistant P388 leukemia cell lines . Cancer Res . 1989 ; 49 : 58 – 62 .
126. Topoisomerase I alteration in a camptothecin-resistant cell line derived from Chinese hamster DC3F cells in culture . Cancer Res . 1992 ; 52 : 1848 – 1854 .
127. Tumor cell resistance to DNA topoisomerase II inhibitors: new developments . Drug Resist Updat . 1999 ; 2 : 382 – 389 .
128. Irinotecan: mechanisms of tumor resistance and novel strategies for modulating its activity . Ann Oncol . 2002 ; 13 : 1841 – 1851 .
129. Cellular resistance to topoisomerase poisons . Cancer Treat Res . 1996 ; 87 : 243 – 260 .
130. Mechanisms of acquired resistance to targeted cancer therapies . Future Oncol . 2012 ; 8 : 999 – 1014 .
131. Bcr-Abl kinase domain mutations, drug resistance and the road to a cure of chronic myeloid leukemia . Blood . 2007 ; 110 : 2242 – 2249 .
132. Mechanisms of resistance to BCR-ABL kinase inhibitors . Leuk Lymphoma . 2011 ; 52 ( Suppl 1 ) : 12 – 22 .
133. Vaults are up-regulated in multidrug-resistant cancer cell lines . J Biol Chem . 1998 ; 273 : 8971 – 8974 .
134. Overexpression of the major vault transporter protein lung-resistance protein predicts treatment outcome in acute myeloid leukemia . Blood . 1996 ; 87 : 2464 – 2469 .
135. Cytidine deaminase and the development of resistance to arabinosyl cytosine . Nat New Biol . 1971 ; 233 : 109 – 110 .
136. Can dihydropyrimidine dehydrogenase impact 5-fluorouracil-based treatment? Eur J Cancer . 2000 ; 36 : 37 – 42 .
137. Biochemical and cellular determinants of bleomycin cytotoxicity . Cancer Surv . 1986 ; 5 : 81 – 91 .
138. The role of glutathione-dependent enzymes in drug resistance . Pharmacol Ther . 1991 ; 51 : 139 – 154 .
139. Glutathione S-transferases in nitrogen mustard-resistant and -sensitive cell lines . Mol Pharmacol . 1987 ; 31 : 575 – 578 .
140. Reduced levels of drug-induced DNA cross-linking in nitrogen mustard-resistant Chinese hamster ovary cells expressing elevated glutathione S-transferase activity . Cancer Res . 1987 ; 47 : 6022 – 6027 .
141. Role of the glutathione redox cycle in acquired and de novo multidrug resistance . Science . 1988 ; 241 : 694 – 697 .
142. Amplification and increased expression of alpha class glutathione S-transferase-encoding genes associated with resistance to nitrogen mustards . Proc Natl Acad Sci U S A . 1988 ; 85 : 8511 – 8515 .
143. Elevation of pi class glutathione S-transferase activity in human breast cancer cells by transfection of the GST pi gene and its effect on sensitivity to toxins . Mol Pharmacol . 1989 ; 36 : 22 – 28 .
144. Adriamycin activation and oxygen free radical formation in human breast tumor cells: protective role of glutathione peroxidase in adriamycin resistance . Cancer Res . 1989 ; 49 : 3844 – 3848 .
145. Glutathione S-transferases and drug resistance . Cancer Cell . 1990 ; 2 : 15 – 22 .
146. Involvement of human glutathione S-transferase isoenzymes in the conjugation of cyclophosphamide metabolites with glutathione . Cancer Res . 1994 ; 54 : 6215 – 6220 .
147. Glutathione-associated enzymes in anticancer drug resistance . Cancer Res . 1994 ; 54 : 4313 – 4320 .
148. The role of human glutathione S-transferase isoenzymes in the formation of glutathione conjugates of the alkylating cytostatic drug thiotepa . Cancer Res . 1995 ; 55 : 1701 – 1706 .
149. MGMT: its role in cancer aetiology and cancer therapeutics . Nat Rev Cancer . 2004 ; 4 : 296 – 307 .
150. Insights into oxazaphosphorine resistance and possible approaches to its circumvention . Drug Resist Updat . 2005 ; 8 : 271 – 297 .
151. DNA repair in resistance to alkylating anticancer drugs . Int J Clin Pharmacol Ther . 2002 ; 40 : 354 – 367 .
152. Cisplatin and DNA repair in cancer chemotherapy . Trends Biochem Sci . 1995 ; 20 : 435 – 439 .
153. ERCC1 and RRM1 gene expressions but not EGFR are predictive of shorter survival in advanced non-small-cell lung cancer treated with cisplatin and gemcitabine . Ann Oncol . 2006 ; 17 : 1818 – 1825 .
154. DNA repair by ERCC1 in non-small-cell lung cancer and cisplatin-based adjuvant chemotherapy . N Engl J Med . 2006 ; 355 : 983 – 991 .
155. DNA synthesis and repair genes RRM1 and ERCC1 in lung cancer . N Engl J Med . 2007 ; 356 : 800 – 808 .
156. DNA repair and survival in lung cancer—the two faces of Janus . N Engl J Med . 2007 ; 356 : 771 – 773 .
157. Clinical resistance to antimetabolites . Hematol Oncol Clin North Am . 1995 ; 9 : 397 – 413 .
158. Biochemical determinants of responsiveness to 5-fluorouracil and its derivatives in xenografts of human colorectal adenocarcinomas in mice . Cancer Res . 1981 ; 41 : 144 – 149 .
159. Inhibitors of apoptosis proteins (IAPs) as potential molecular targets for therapy of hematological malignancies . Curr Mol Med . 2011 ; 11 : 633 – 649 .
160. The intrinsic apoptosis pathways as a target in anticancer therapy . Curr Pharm Biotechnol . 2012 ; 13 : 1426 – 1438 .
161. Gene expression profiles in paraffin-embedded core biopsy tissue predict response to chemotherapy in women with locally advanced breast cancer . J Clin Oncol . 2005 ; 23 : 7265 – 7277 .
162. Pharmacogenomic predictor of sensitivity to preoperative chemotherapy with paclitaxel and fluorouracil, doxorubicin, and cyclophosphamide in breast cancer . J Clin Oncol . 2006 ; 24 : 4236 – 4244 .
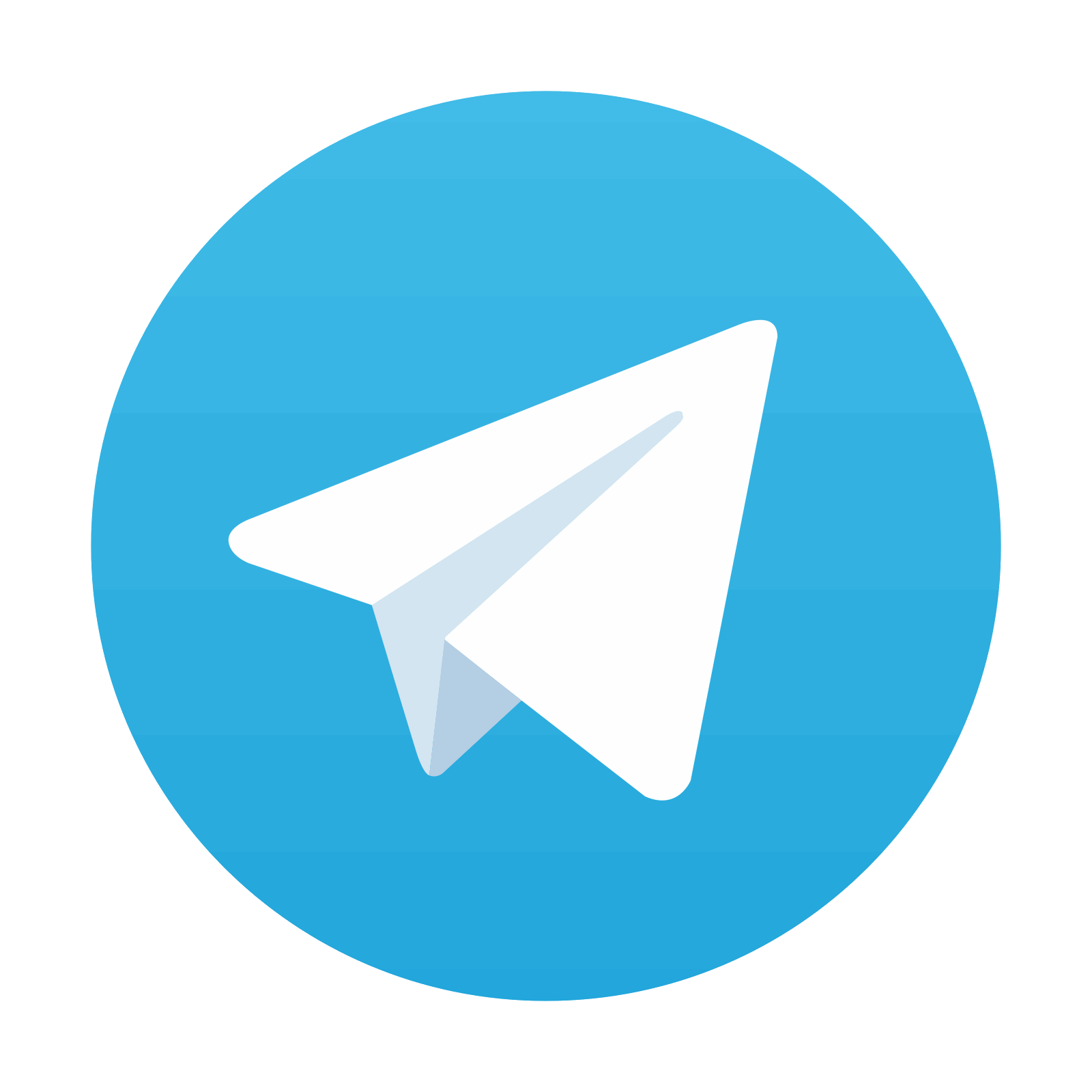
Stay updated, free articles. Join our Telegram channel

Full access? Get Clinical Tree
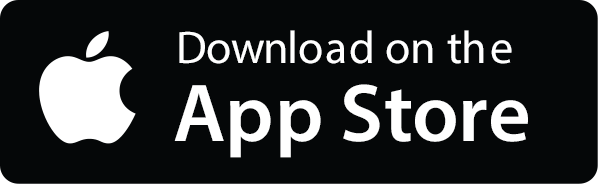
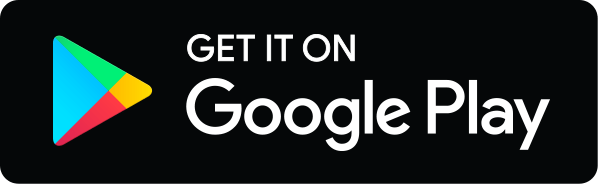