Domenico Coppola (ed.)Cancer Growth and ProgressionMolecular Pathology and Diagnostics of Cancer201410.1007/978-94-007-7192-5_16
© Springer Science+Business Media Dordrecht 2014
16. Molecular Pathology and Diagnostics of Cutaneous Malignancy
(1)
H. Lee Moffitt Cancer Center and Research Institute, 12902 Magnolia Drive, Tampa, FL 33612, USA
16.1 Introduction
16.2 Familial Melanoma
16.3 Sporadic Melanoma
16.4.1 BRAF
16.4.2 c-KIT
16.4.3 N-RAS
16.5 Basal Cell Carcinoma
16.8 Conclusion
Abstract
Advances and discoveries in molecular genetics over the past decade have literally changed the face of diagnosis and treatment decisions for patients with melanoma and non-melanoma skin cancer. These include genetic analysis of skin biopsies as a diagnostic aid, genotyping of tumors from patients with malignant melanoma for personalized treatment of metastatic disease, and discovery of a new virus thought to play a role in the pathogenesis of Merkel cell carcinoma. Initiated by the discovery of a familial cancer gene in patients with familial melanoma and atypical nevi over three decades ago, molecular pathology techniques now play a central role in genetic testing for melanoma risk, in evaluation of melanocytic proliferations with uncertain biologic potential, and in stratifying patients with metastatic melanoma for treatment with targeted therapeutic agents, driving the search for better markers of prognosis and response to therapy. Targeted therapy is now available for treatment of unresectable or metastatic basal cell carcinoma. It is likely that continual improvements in techniques for analyzing blood and fresh and archival tumor tissue will uncover the pathogenesis of skin tumors related and unrelated to sun exposure, spawn the development of vaccinations or chemopreventive agents for these tumors, and enable physicians to customize treatment based on unique patient and tumor characteristics. This chapter will highlight relevant information concerning the molecular pathogenesis of melanoma, basal and squamous cell carcinoma, and Merkel cell carcinoma. Sections on molecular techniques available for diagnosis, prognosis, and treatment selection, emphasizing melanoma where this is most highly developed, are included
Keywords
Nonmelanoma skin cancerMolecular genetics of cutaneous malignancyMelanoma prognosisPrognostic biomarkersMerkel cell carcinoma pathogenesisAbbreviations
AE
Aloe-emodin
ASIP
Agouti signaling protein
BCC
Basal cell carcinoma
CGH
Comparative genomic hybridization
EGFR
Epidermal growth factor receptor
ERK
Extracellular signal-regulated kinase
FISH
Fluorescent in situ hybridization
GST
Glutathione S-transferase
GWASs
Genome-wide association studies
Hh
Hedgehog
MAPK
Mitogen-activated protein kinase
MC1R
Melanocortin 1 receptor
McPyV
Merkel cell polyomavirus
MEK
MAPK extracellular signal-regulated kinase
PCR
Polymerase chain reaction
SCC
Squamous cell carcinoma
SNP
Single nucleotide polymorphism
UV
Ultraviolet
16.1 Introduction
An estimated 76,250 new cases of cutaneous melanoma will have been diagnosed in the United States in 2012 [1], and this disease will claim the lives of approximately 9,000 American men and women this same year [1]. In contrast, greater than two million new cases of nonmelanoma skin cancer are anticipated to occur in the United States in 2012, while deaths from these malignancies are projected to be less than 1,000 [2]. Given the disproportionate mortality of melanoma compared to other skin cancers, detection of early-stage disease constitutes a major effort in all diagnostic endeavors. Many of the strides made in the molecular biologic analysis of melanoma have been aimed at increasing diagnostic accuracy. This is an important goal, especially in light of the number of skin biopsies performed to rule out melanoma—estimated to be more than one to two million in the United States in 2008 alone [3]. While histopathologic examination of suspicious pigmented lesions remains the “gold standard” for diagnosis, and the vast majority of malignancies can be readily distinguished from melanocytic nevi on the basis of histopathology, cases not so easily discernable can wreak havoc. The consequences of both false negative and false positive diagnoses are considerable: false positive diagnosis may subject the patient to undue mental anguish, financial burden, difficulties in attaining health insurance, and disfiguring surgical as well as toxic medical interventions. False negative diagnosis of melanoma, in addition to delaying appropriate treatment, is the single most common cause of medical malpractice lawsuits against a pathologist [4]. There have been numerous studies focusing on diagnostic discrepancy rates in melanocytic lesions [5–16]. In one illustrative example, experienced dermatopathologists considered experts in their field rendered opposing histopathologic diagnoses in as many as 25 % of cases reviewed [14].
Current molecular diagnostic tests, employed for the purpose of increased diagnostic accuracy and selection of targeted therapy, are informed by the earliest research into the molecular pathogenesis of melanoma. Therefore, this section will highlight current knowledge into the molecular basis for the disease, followed by discussion of established and emerging molecular techniques that may assist in the diagnosis and treatment of melanoma.
16.2 Familial Melanoma
Familial melanoma accounts for only 10 % of melanoma cases [17] but formed the basis for the earliest studies on genetic abnormalities in this disease, specifically mutations in p14/ARF, p16/INK4a, and CDK4. The best-characterized high-penetrance susceptibility gene predisposing to melanoma is CDKN2A, located on chromosome 9p21 [18–23]. This gene encodes two distinct tumor suppressor proteins (p14/ARF and p16/INK4a), implicated in the oncogenesis of melanoma. The former hinders neoplasia through its indirect effect on p53 by binding to and inhibiting the function of human homolog of murine Mdm2 (HDM2), a protein that tags other proteins for ubiquitination. In mutated form, p14/ARF cannot bind to and suppress HDM2, allowing it to mark p53 for ubiquitination. With less p53 available to identify damaged DNA, genomic instability results, predisposing the afflicted individual to the development of melanoma. p16/INK4a functions in concert with retinoblastoma protein, a tumor suppressor protein, to regulate the G1 phase of the cell cycle. In familial melanoma patients, compromised expression of p16 allows for uninhibited phosphorylation of retinoblastoma protein. This leads to transcription of genes encoding cyclin E, a protein that is required for the initiation of DNA replication in the S phase. CDK4 is another high penetrance but low-frequency melanoma susceptibility gene identified in linkage studies [19–22]. Located on chromosome 12q14, CDK4 encodes cyclin-dependent kinase 4 protein, a constituent of the CDK4/6 complex discussed above. Germline mutations that activate this gene occur at codon 24 (Arg24Cys and Arg24His) and render the CDK4/6 complex resistant to p16 inhibition. Thus, mutations in the p15/INK4a and CDKN2A genes produce a similar effect.
Additional genes involved in cases of familial melanoma have been identified through the use of genome-wide association studies (GWASs). While linkage studies have allowed the identification of the aforementioned high-penetrance, low-frequency genetic variants implicated in familial melanoma, GWASs have enabled high-powered searches for low-penetrance, high-frequency melanoma susceptibility genes. The melanoma genetics consortium, GenoMEL, performed the largest of these studies with the purpose of identifying common genetic variants contributing to melanoma risk across populations of European ancestry residing at varying altitudes [24]. This study found the strongest association in a single nucleotide polymorphism (SNP) that is a likely variant of the MC1R (melanocortin 1 receptor) gene, located on chromosome 16q24. This SNP was also discovered to be associated with hair color and skin pigmentation in a separate GWAS [25]. The link between pigmentation phenotype and melanoma susceptibility has long been established by epidemiological studies [26]. Thus, the relationship between allelic variants of the MC1R gene and both pigmentation phenotype and melanoma risk is not unexpected. The GenoMEL study also corroborated other previously suggested melanoma associations—locus 11q14-21 encompassing tyrosinase and locus 20q11.22, near ASIP (agouti signaling protein)—as well as two SNPs, for which prior associations with melanoma were not made. These latter two variants were located on chromosome 9p21, respectively, within the methylthioadenosine phosphorylase gene, which flanks CDK2NA, and ANRIL, an antisense noncoding mRNA that overlaps with the promoter of the p14ARF transcript of the CDK2NA gene and the transcribed sequence of CDK2NB.
A separate GWAS of nevus count using cohorts from the UK and Australia identified alleles at two strongly associated loci: 9p21 (MATP) and 22q13.1 (PLA2G6, a member of the phospholipase A2 superfamily of genes) [27]. The authors of this study were then able to associate the SNPs at these loci with melanoma susceptibility by analyzing two melanoma case-control samples. Interestingly, the signals within 9p21 of this GWAS overlapped with those of the GenoMEL study. Furthermore, the authors of the GenoMEL study were able to replicate the association between the SNPs at 22q13 and melanoma risk as well. Given these associations of the SNPs identified in the nevus study with melanoma, as well as the well-known positive correlation between number of melanocytic nevi and risk for melanoma, it is reasonable to assume that the risk of developing melanoma at these loci with these SNPs is, at least in part, mediated through nevus count [28, 29]. The discovery of these and additional susceptibility genes may yield further insight into the biological pathways involved in melanomagenesis.
At present, there are no formal guidelines for genetic testing of melanoma patients, their kindred, or their tumor samples for the p16/CDKN2A mutation. Potential benefits of such testing include the possibility of increased compliance in preventive programs with a positive test result. A negative test result in a patient with relatives who have died from melanoma or pancreatic cancer with the germline mutation could be reassuring. Those opposed to such testing cite the lack of identifiable germline mutations in known high-risk genes in affected members of known melanoma families. Conversely, non-gene carriers in CDKN2A-positive families may still develop dysplastic nevus syndrome and develop melanoma. Thus, negative test results could confer a false sense of reassurance and be a disincentive to continued participating in screening/prevention activities. Finally, it is difficult to assign an absolute risk for development of disease in individuals who do have a germline mutation identified [30]. It is reasonable to conclude that genetic testing for germline CDKN2a mutations should be performed only in patients that have a significant risk of having a positive test result (i.e., those with three or more affected members) and only in conjunction with a thorough explanation of the limitations of the test, in conjunction with qualified genetic counseling and education [31].
16.3 Sporadic Melanoma
Research into the molecular basis of sporadic melanoma has implicated many genes, particularly those encoding proteins involved in the mitogen-activated protein kinase (MAPK) pathway [19, 20, 22, 23, 32, 33]. With up to 90 % of melanomas harboring constitutive activation of this pathway, great interest lies in detailing the precise mechanisms involved, with the hope of intervening at key junctions in an effort to thwart melanomagenesis and/or progression [33]. The MAPK pathway regulates cellular proliferation through signal transduction along the cascade of RAS, RAF, MAPK extracellular signal-regulated kinase (MEK), and extracellular signal-regulated kinase (ERK). Activated ERK induces several proliferative and survival processes, one of which is activation of the cyclin D-CDK4/6 complex (discussed above) upon translocation to the nucleus. Mutational analyses have yielded key aberrations in crucial players in the MAPK pathway, including BRAF, NRAS, c-Kit, and epidermal growth factor receptor (EGFR).
16.4 Molecular Diagnostic Tests
16.4.1 BRAF
There are three mammalian RAF proto-oncogenes—ARAF, BRAF, and CRAF. However, of these, only activating mutations of BRAF have thus far been shown to be present in a significant percentage of human malignancies, with the highest rate occurring in melanoma [19, 20, 22, 23]. BRAF-mutated melanomas tend to occur at anatomic sites exposed to intermittent, rather than chronic, sun damage. With approximately 70 % of cases harboring such a mutation, BRAF is the most commonly mutated proto-oncogene in cutaneous melanoma. Furthermore, a significant proportion of both benign and dysplastic melanocytic nevi have been shown to harbor mutations of BRAF as well, suggesting a relatively early event in melanomagenesis [19, 22, 23, 34].
Greater than 90 % of BRAF mutations in melanoma result from a single base missense mutation (T → A) at codon 1799 that leads to the substitution of valine in favor of glutamic acid at position 600 of the BRAF protein [35]. This alteration introduces a conformational change in BRAF’s kinase domain, which can lead to a 480-fold increase in kinase activity when compared to wild-type BRAF [36]. This mutated BRAF induces uncontrolled proliferation in melanoma. Ongoing research of this molecule includes its role in inducing senescence of benign melanocytic nevi, the mechanisms involved in bypassing this senescent response in the development of melanoma from such nevi, and the mechanisms for acquired resistance to targeted inhibition of this molecule in patients undergoing anti-BRAF therapy [37–48].
A major breakthrough in the treatment of metastatic melanoma has been the successful treatment of BRAF-mutant melanoma with selective BRAF inhibitors such as vemurafenib and dabrafenib [49]. Since this agent shows tenfold greater activity for V600E-mutant melanoma compared to wild-type melanoma, most current clinical trials require pre-treatment assessment for the presence of this mutation [50]. The mutation is ascertained by means of a polymerase chain reaction (PCR) assay (TaqMan, Applied Biosystems), which involves hybridizing a probe specific to the 1799T->A substitution with DNA isolated from formalin-fixed, paraffin-embedded tumor tissue and determining the presence or absence of amplification after repeated chain-reaction cycles [51]. This assay is now routinely available at most major commercial reference laboratories. There is also a commercially available monoclonal antibody (VE1) that can be used to assess for the BRAF V600E mutation immunohistochemically. This antibody was 100 % sensitive and specific for the mutation compared to the gold standard of molecular analysis in a recent series of 44 cases [52]. There have also been attempts at predicting BRAF mutation status by analyzing various clinical and histologic features of primary tumors. In a recent study of 302 primary melanomas, groupings of various combinations of 17 such factors revealed that phenotypic features such as increased upward scatter, intraepidermal nesting, epidermal thickening, sharp lateral demaracation of the tumor, and the presence of rounded, highly pigmented tumor cells were distinct features of melanomas with BRAF mutation. In this study, classification tree analysis showed that BRAF mutation status could be correctly predicted in up to 82 % of tumors using certain variables [53]. While there is certainly great interest in correlating easily analyzed clinicopathologic variables with mutation status, and active research aimed at this goal, at present, however, the gold standard for assessing tumors for BRAF status remains mutation analysis.
16.4.2 c-KIT
c-KIT, a proto-oncogene that encodes the type III receptor tyrosine kinase KIT, was first identified in 1987 as a result of sequence similarity to the Hardy-Zuckerman 4 feline sarcoma virus oncogene, v-KIT [54]. Upon binding its ligand, stem cell factor, KIT undergoes receptor dimerization, autophosphorylation, and activation of its intracellular tyrosine kinase domain [18, 55]. Once activated, KIT is capable of stimulating downstream signaling pathways, such as MAPK [18, 55, 56].
Activating mutations and/or gene amplification of KIT have been described in distinct subsets of melanoma [57–61]. In one study, aberrations were found in 39 % of mucosal melanomas, 36 % of acral melanomas, and 28 % of melanomas arising in chronically sun-damaged skin (as defined by the presence of solar elastosis on review of histopathology)—anatomic sites at which BRAF mutations occur far less frequently [60].
The most prevalent KIT mutations in melanoma occur in exons 11, 13, and 17 [62]. These mutations are thought to promote the constitutive activation of KIT either through precluding the protein from assuming its default autoinhibited conformation or by promoting its dimerization in the absence of stem cell factor [63, 64]. The precise manner in which constitutive KIT activation promotes melanomagenesis remains unclear. Nonetheless, recognizing activating mutations of KIT as well as copy number gains can be of great consequence, as this subtype of melanoma may respond the receptor tyrosine kinase imatinib [65–67]. Since the initial case report of a dramatic response to imatinib in c-KIT-mutated melanomas [65], interest has been raised in treating patients with acral and mucosal melanoma with this agent, leading to several ongoing phase II clinical trials (ClinicalTrials.gov) of imatinib and other c-KIT blockers such as sunitinib, dasatinib, and sorafenib. These studies require documentation of mutation or amplification of the c-kit gene. Currently, therefore, it seems reasonable that subgroups of patients with metastatic melanoma prone to c-kit mutations, such as primary mucosal or acral lentiginous tumors, be analyzed for KIT mutation status. If mutated, a therapeutic trial with a c-KIT blocking agent has been suggested. Testing, using PCR following by sequencing analysis of exons 11, 13, and 17 can be performed at several reference laboratories, including ARUP, as well as university laboratories such as those at University of Washington and University of Michigan.
16.4.3 N-RAS
The RAS proto-oncogene family is at the initiating point of the MAPK pathway, and theoretically activating mutations of these molecules should provide the affected cell with continuous growth signals in the absence of extracellular stimuli. Indeed, RAS molecules have documented roles in melanomagenesis [18–20, 22, 23, 33]. Of the three members of this family (H-RAS, K-RAS, and N-RAS), only the latter has been shown to sustain activating mutations in melanocytes, as well as nearly one third of all melanomas [19, 23, 33]. The most commonly documented N-RAS aberration in melanoma is a missense mutation at codon 61 (Q61R), which results in the substitution of arginine in place of glutamine and impairs GTP hydrolysis, locking the protein in a state of constitutive activation [68]. However, therapeutic attempts at blocking the farnesylation, which is required for proper function of the molecule, using farnesyltransfer inhibitors, have yielded disappointing results [56, 69].
There has been a resurgent interest in the role of the N-RAS pathway in sustaining melanoma growth in the wake of the phenomenon of acquired resistance to BRAF inhibitors. Specifically, investigations into this phenomenon in cell lines have revealed that hyperactivation of the MEK-ERK1/2 pathway occurs rapidly after treatment with the BRAF inhibitor PLX4032, and this has been noted most prominently in cell lines containing the activating N-RAS mutation, which demonstrated resistance to apoptosis [70, 71]. Therefore, future trials of targeted therapies may require genotyping for N-RAS to stratify treatment.
16.4.4 Epidermal Growth Factor Receptor (EGFR)
EGFR is yet another receptor tyrosine kinase that is involved in activation of the MAPK and PI3K/Akt signal transduction pathways. The latter pathway is also activated by RAS and results in decreased apoptosis [19]. The selective expression of this receptor in human melanoma cells harboring an extra copy of chromosome 7 was first studied in 1985 [72]. Numerous studies have since investigated the importance of this receptor in melanomagenesis and/or progression with contradictory results. More recently, however, researchers have shown an association between polysomy 7 and EGFR gene amplification by fluorescent in situ hybridization (FISH), with significantly higher amplification in tumors that metastasized within 2 years of surgical excision of the primary lesion [73]. Implying a poorer prognosis in this latter subset of patients, further studies into the potential benefit of anti-EGFR therapy for those with EGFR alterations have been recommended. However, at this time there appears to be no clinical utility to testing tumors for EGFR overexpression.
16.4.5 Comparative Genomic Hybridization
The technique of comparative genomic hybridization (CGH), wherein lesional DNA as well as normal control DNA are hybridized to either normal metaphase chromosomes or an array of thousands of defined DNA probes (array CGH) in order to detect copy number changes, has been used to examine differences between melanoma and benign melanocytic lesions. Specifically, gains of chromosomes 1q, 6p, 7p, 7q, 8q, 17q, and 20q and losses of chromosomes 6q, 9p, 9q, 10p, 10q, and 11q have been observed in greater than 95 % of melanomas [74]. Loss of chromosome 9, an early and common aberration in melanomagenesis, typically involves deletion of the distal region of the p arm that encompasses the 9p21 locus [75]. Of note, gain of 6p is a frequent aberration in both primary and malignant melanomas and may confer a poorer outcome [76, 77].
Melanocytic nevi rarely demonstrate chromosomal aberrations, with the exception of Spitz nevi, which may harbor gains on occasion [74, 78–80]. A gain of the entire short arm of chromosome 11 is the most commonly encountered aberration in Spitz nevus and is an abnormality not detected in primary cutaneous melanomas. Although most congenital nevi are readily distinguished from melanoma according to established histopathologic criteria, atypical nodular proliferations that arise in congenital nevi can be problematic [78]. These lesions simulate nodular melanomas microscopically, but tend to regress within a few months. CGH offers diagnostic aid in this area [78, 80]. While cases of atypical nodular proliferations show chromosomal aberrations by CGH, the pattern of involvement is distinct from that created by melanoma. Abnormalities in the former group involve only entire chromosomes. While gains or losses of entire chromosomes can frequently occur in melanoma as well, 95 % of such cases are paralleled by gains or losses of chromosomal fragments.
CGH has also underscored significant genetic differences between acral melanoma and superficial spreading melanoma [81], justifying the distinction between these subtypes by demonstrating disparity in pattern of chromosomal aberration between the two [80, 81]. While acral melanomas have been shown to invariably harbor gene amplifications (defined as greater than a threefold increase in copy number), superficial spreading melanomas, of comparable tumor thickness and patient age, contain an amplicon in less than 15 % of cases. The amplified regions frequently consist of areas containing known oncogenes. Study of the amplicons not harboring obvious candidate genes may provide further insight into the mechanisms of melanomagenesis.
Isolated melanocytes located beyond the microscopically-designated free margins of resection have been shown to harbor these genetic amplifications as well [80, 81]. Researchers believe that these field cells represent unrecognized radial growth of the acral melanoma and are likely responsible for local recurrences. If further studies support such a notion, then CGH may play an instrumental role in shaping future guidelines pertaining to the attainment of clear margins of resection.
16.4.6 Fluorescent In Situ Hybridization
A multiprobe fluorescent in situ hybridization (FISH) assay against several of the aforementioned chromosomal abnormalities detected in CGH studies has been developed and tested in several studies with promising results [3, 82]. The assay identifies copy number alterations in chromosomes 6p25 (RREB1), 6q23 (MYB), and 11q13 (cyclin D1) as well as centromere 6. Results of these preliminary studies have demonstrated high sensitivity (~87 %) as well as specificity (~95 %) in distinguishing benign melanocytic nevi from melanomas using this particular panel of probes.
Therefore, this technique plays a potential role in classifying problematic melanocytic lesions. The area of most intense investigation of the applicability of this technique is in the distinction between spitzoid melanoma and benign Spitz nevus. Two recent studies have demonstrated sensitivity and specificity of 43–60 % and 50–80 % for determination of malignancy in ambiguous Spitzid lesions, using metastatic behavior as the gold standard [83, 84]. In another recent, albeit small, study evaluating the efficacy of FISH in distinguishing nodular nevoid melanomas from mitotically active melanocytic nevi with probes targeting these same for four loci, chromosomal aberrations were detected in each of the nevoid melanomas and in none of the melanocytic nevi [85]. Other scenarios for which FISH has shown potential benefit include distinguishing epithelioid blue nevus from blue nevus-like cutaneous melanoma metastasis [86], distinguishing intranodal nevus from metastatic melanoma [87], classifying superficial melanocytic neoplasms with pagetoid melanocytosis [88], and the microstaging of melanoma arising in association with a nevus [89]. Several commercial laboratories now offer this test for use in formalin-fixed, paraffin-embedded tissue using probes developed by Abbott Laboratories (Caris Life Sciences, Neogenomics Laboratories).
16.4.7 Immunohistochemical Staining for Prognostic Biomarkers
There has been abundant research aimed at identifying biomarkers for prognosis as well as treatment response. The widespread availability of immunohistochemical staining makes this technique one of the most widely investigated, because it can characterize patterns of protein expression while maintaining preservation of tissue and cellular architecture. There have been literally thousands of manuscripts devoted to potential molecular markers of melanoma that could identify patients at greatest risk for recurrence, or most likely to benefit from adjuvant therapy. Two recent systematic reviews of published data on immunohistochemistry-based prognostic molecular markers for cutaneous melanoma have been performed [90, 91]. The first of two reviews sought to report on associations between immunohistochemical expression of protein biomarkers and survival outcomes in cases of cutaneous melanoma. Molecular markers were then scored according to the protein’s major biological function, as categorized by a modified Hanahan-Weinberg functional capabilities scheme. This review identified effectors of DNA replication, cyclin-dependent kinase inhibitors, growth-promoting transcription factors, and regulators of tissue invasion and metastasis as having a statistically significant impact on patient outcome. Ki-67, proliferating cell nuclear antigen, metallothionein, Ku70, Ku80, and microtubule-associated protein-2 were consistently associated with disease-free as well as overall survival. Interestingly, cyclins and cyclin-dependent kinases were not associated with melanoma prognosis. More recently, a significant association between Ki-67 expression and melanoma-specific mortality—in addition to dermal mitoses, which remain a significant and independent prognostic factor—has been shown in a large and representative population of patients diagnosed with thin (≤ 1 mm in thickness) cutaneous melanomas [92]. With external validation in a similar population-based sample, it is possible that Ki-67 may be formally adopted into routine clinical practice, either as a prognostic factor for disease recurrence or a predictive factor of positive sentinel lymph node biopsy.
Expression of cyclin-dependent kinase inhibitors was significantly associated with mortality. For example, increased levels of p16 were associated with decreased all-cause and melanoma-specific mortalities, while increased levels of p27, which typically cause cells to arrest in the G1 phase of the cell cycle, are paradoxically associated with increased mortality. The rationale behind this observation lies in the activation of p27 through cytoplasmic sequestration, rather than protein degradation. Cytoplasmic accumulation of p27 has been associated with metastatic potential [93]. Among proteins involved in growth signaling, statistically significant associations were most frequent among transcription factors, ATF-2 and AP-2α, and transcriptional coactivators, NCOA3/AIB-1. This observation suggests altered transcriptional regulation as a crucial step in regulation of melanoma-specific survival. Increased expression of three cellular adhesion molecules was shown to be associated with statistically significant worsening of disease-free survival: the melanocyte-specific melanoma cell adhesion molecule (MCAM/MUC18), neuron-specific L1 cell adhesion molecule (L1-CAM), and glandular tissue-associated carcinoembryonic antigen-related cell adhesion molecule 1 (CEACAM-1). These molecules are thought to promote tumor infiltration and distant spread through abnormal tumor-stroma interactions [94–97]. Also, elevated levels of osteopontin and tenascin-C—members of the matricellular protein family, which consists of secreted molecules that interface between cell surface receptors and the extracellular matrix [98]—were associated with statistically significant worsening of melanoma-specific mortality and disease-free survival, respectively. Among proteases, increased levels of tissue plasminogen activator and matrix metalloproteinase-2 were statistically significant for mortality outcomes.
16.5 Basal Cell Carcinoma
Basal cell carcinoma (BCC) is the most common invasive cancer in the Western world, and it accounts for 75 % of skin malignancies overall with increasing rates worldwide [99–101]. Ultraviolet (UV) radiation, more specifically of the UV B-wavelength, is the dominant etiologic agent thought to be responsible for most skin carcinomas [99, 102]. It does so by genetically altering genes involved in initiation, promotion, and progression of both melanoma and non-melanoma skin cancers. Since the discovery of the hedgehog gene and its influence on the development of carcinoma in certain populations, great strides have been made in uncovering other genetic abnormalities and the possible use of specific inhibitors in treatment of the disease [103, 104]. These emerging genetic alterations, originally studied in inheritable disorders, such as xeroderma pigmentosum and nevoid basal cell carcinoma syndrome (also known as Gorlin syndrome), have offered new insight into its molecular basis.
16.5.1 Inherited Forms of Basal Cell Carcinoma
There are several major mechanisms involved in inherited forms of BCC: defects in detoxifying proteins, DNA repair, and embryonic signaling pathways. Defects in these pathways cause well-recognized skin cancer syndromes, including xeroderma pigmentosum, Gorlin syndrome, and familial cylindromatosis, and play a role in sporadic BCC as well. One of the most important components that protect the skin against oxidative stress after UV irradiation is the glutathione S-transferase (GST) enzyme system [105]. In humans, GST is expressed in melanomas, but only weakly in BCC [106]. Several GST polymorphisms have been identified in patients with high UV sensitivity and increased risk for BCC [105]. Impaired DNA repair, especially in patients with xeroderma pigmentosum, predisposes to non-melanoma skin cancer, preferentially of the squamous cell carcinoma (SCC) type (discussed later in this chapter). Of the embryonic signaling pathways, the most important is the hedgehog (Hh) signaling pathway.
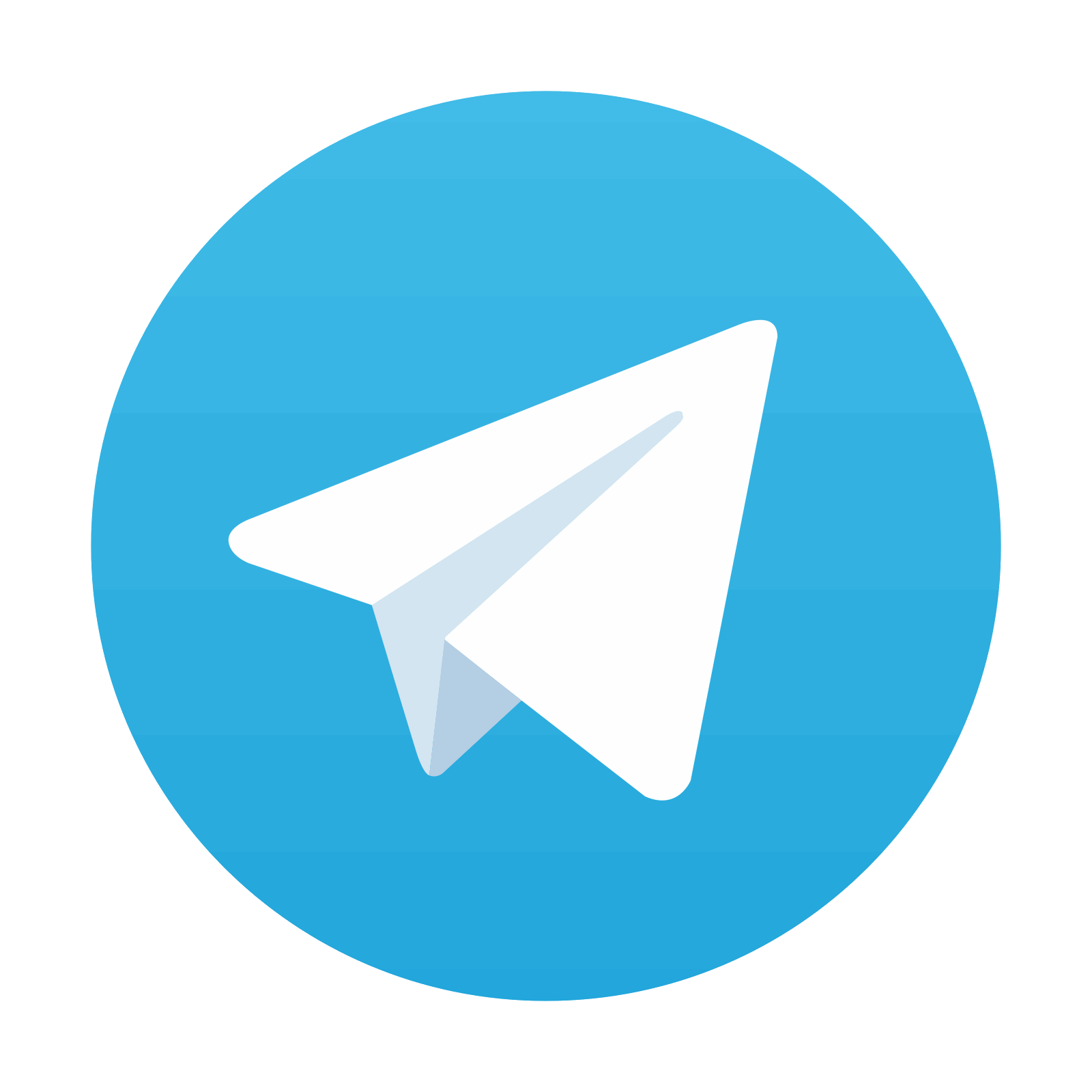
Stay updated, free articles. Join our Telegram channel

Full access? Get Clinical Tree
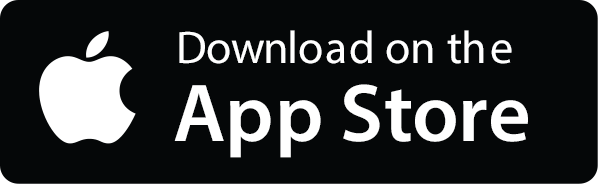
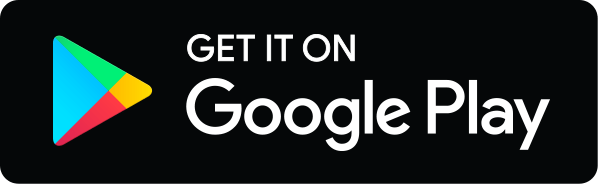