Fig. 8.1
Management of a thyroid nodule based on fine needle aspiration biopsy results. (a) Historical management decisions for thyroid nodules: fine needle aspiration (FNA) biopsy provides a thyroid tissue specimen for cytopathologic evaluation and placement into one of six categories according to the Bethesda system for reporting thyroid cytopathology, which imply specific risk of malignancy. Benign and malignant cytologies are considered sufficiently accurate to recommend nodule observation or thyroidectomy, in these two situations, respectively. For nodules that are indeterminate, considered to be Bethesda classes III (AUS/FLUS), IV (SFN/FN), and V (suspicious for malignancy), the optimal management remains uncertain. In the former two categories, malignancy risk is low but not excluded, and observation may miss a clinically relevant malignancy, while surgical resection may be unnecessary. For nodules with Bethesda V cytology, malignant risk is often sufficiently high to warrant surgical resection (60–70%), but the extent of surgery (lobectomy versus near-total thyroidectomy) is uncertain. Nodules with non-diagnostic (ND) cytology are low risk (1–4% cancer risk) and can typically be managed non-operatively, though a subset of repeatedly ND nodules will be removed. (b) Current recommended schema of thyroid nodule management using adjunct molecular testing: for nodules with indeterminate cytologies, further evaluation with molecular testing allows for classification as low or high risk for malignancy. When test performance has been shown in prospective, multicenter, blinded validation studies to have a sufficiently high negative predictive value (NPV), indicating a very low chance of a “false-negative” or missed cancer, nodule observation can be selected in lieu of diagnostic lobectomy avoiding an unnecessary surgery. Conversely, when a test with very high positive predictive value (PPV) indicates the presence of cancer, thyroidectomy is indicated, and near-total thyroidectomy as initial surgery rather than diagnostic lobectomy may be appropriately performed. The management of ND, benign, and malignant nodules is unchanged with current molecular testing. B = Bethesda classification. Dx = diagnostic. AUS/FLUS = atypia of undetermined significance/follicular lesion on undetermined significance. SFN = suspicious for follicular neoplasm/follicular neoplasm. SM = suspicious for malignancy
The Approach to Thyroid Nodules
Most thyroid nodules are asymptomatic except as a palpable mass detected by the patient or medical practitioner, and may be discovered incidentally by cross-section imaging [1, 2]. Most recent large series of consecutive nodules suggest that approximately 8–15% of thyroid nodules will prove to be malignant [3–5]. Clinical factors such as sex, age [6], childhood exposure to ionizing radiation [7], and rarely symptoms or palpable lymphadenopathy are present that increase the possibility that a thyroid nodule will be malignant. Thus, a full medical history and physical examination should be obtained on all patients who present for evaluation of a thyroid nodule.
Ultrasound is the optimal imaging technique for thyroid nodule evaluation. Beyond measurement of nodule size, sonographic features can assist with nodule risk assessment [8]. However, low to moderate inter-rater reproducibility of findings limits the precision and accuracy of interpretation [9]. For this reason, UG-FNA to obtain material for cytopathologic evaluation is recommended for most thyroid nodules larger than 1–2 cm [10]. Although there is high diagnostic accuracy of benign and malignant cytology, ~20–25% of aspirates will be cellularly sufficient but cytologically indeterminate. The Bethesda classification system effectively subdivides indeterminate cytologic findings into distinct categories associated with escalating risk of malignancy that improve the ability of clinicians to stratify the risk of thyroid malignancy [11–14], but inter-rater and intra-rater reliability of cytologic interpretation remains poor [15].
Because the risk of thyroid malignancy is generally low but not excluded in these cases, key considerations are whether such a nodule can be monitored conservatively or should be surgically removed and what extent of surgery (thyroid lobectomy or near-total thyroidectomy) is most appropriate [10, 16, 17]. These options carry risks and benefits, and the optimal management remains uncertain, in part, because of the limitations inherent to the clinical, sonographic, and cytologic assessments described above, indicating the need for synergistic forms of assessment, such as molecular testing, for such nodules.
Molecular analysis of nodular tissue has proved highly valuable. As price points for these diagnostic tests have fallen, cost-effectiveness analyses also suggest that the use of diagnostic molecular tests may reduce cost while improving quality-adjusted life years [18, 19]. Thus, the approach to cytologically indeterminate thyroid nodules is increasingly employing molecular diagnostic testing. Below, we provide a description of the molecular markers thus far evaluated for use in the care of patients with clinically relevant thyroid nodules.
Molecular Markers
A molecular marker assessed on fine needle aspiration material from a thyroid nodule biopsy may target one of many cellular constituents, such as expressed proteins, RNA, or DNA, measured either quantitatively or qualitatively using specific laboratory techniques. Some of the earliest investigations of molecular markers utilized IHC techniques on slides prepared from aspiration material to identify expressed proteins differentiating benign from malignant nodules. The identification of prevalent oncogenic mutations/translocations in thyroid cancer, including BRAF, RAS, and RET/PTC, led to intense focus on the DNA alterations in thyroid cancer, followed closely by advanced nucleic acid sequencing techniques allowing expression profiling of mRNA or microRNA, all of which have been investigated as molecular markers for thyroid nodule evaluation. Limitations inherent with the use of any single molecular marker have in some cases spawned the use of combinations of molecular tests improving test accuracy.
In each case, the process begins with identifying a possible molecular marker and creating a laboratory test to reliably detect it, which allows understanding of the test’s analytic validity. How well the marker discriminates benign from malignant nodules, especially in clinical practice, determines the test’s clinical validity. Whether or not knowing molecular marker status changes patient management when incorporated into clinical decision-making establishes its clinical utility and is important for determining the clinical value of the testing. Finally, the cost-effectiveness of using the molecular tests compared to other management requires evaluation within any large healthcare framework. Each of these aspects must be considered in the evaluation of a molecular marker as it is applied to clinical medicine. While the molecular markers described in this chapter (Table 8.1) have largely shown consistent analytic performance, the clinical validity, clinical utility and cost-effectiveness frequently remain unknown.
Table 8.1
Molecular markers
Immunohistochemistry |
Galectin-3 |
HBME-1 |
CK19 |
Mutations and gene rerrangements |
BRAF (V600E) |
RAS (H-RAS, K-RAS, N-RAS) |
RET/PTC (RET/PTC1, RET/PTC3) |
PAX8/PPARγ |
Asuragen miRInform™ thyroid panel |
ThyroSeq v2 panel |
Gene expression and microarray analysis |
MicroRNA expression |
Afirma® gene expression classifier |
Galectin-3
Galectin-3 is a lectin that binds to cell surface glycoproteins and interacts with intracellular proteins regulating cellular functioning including cell growth, apoptosis, and malignant transformation [20]. Its presence has been measured by IHC using a monoclonal antibody targeting galectin-3 on formalin-fixed and paraffin-embedded (FFPE) cellblock preparations from thyroid nodule aspires. Early investigations found that galectin-3 expression correlated strongly with thyroid cancers compared to benign specimens [21, 22].
Assessment of galectin-3 as a clinical test to improve diagnostic accuracy in cytologically indeterminate thyroid nodules has been performed. In a large multicenter prospective study, galectin-3 expression was assessed in 465 nodules with indeterminate cytology with histopathology available for blinded review and showed a sensitivity of 78% and specificity of 93%, yielding an 82% positive predictive value (PPV) and a 91% negative predictive value (NPV) in this population of patients [23]. While galectin-3 remains a marker of interest, there has not been more extensive validation of the test or robust data on how testing effects clinical care, and its initial performance has not been replicated. For these reasons, as well as the interpreter subjectivity surrounding its use, galectin-3 has shown greater promise in histopathologic assessment as opposed to cytologic assessment.
HBME-1
HBME-1 (Hector Battifora Mesothelial-1) is a monoclonal antibody targeting the microvilli of mesothelioma cells. However, the antigen recognized by HBME-1 has also been detected on differentiated thyroid cancer cells, leading to its development as a molecular marker for assessing malignancy risk. HBME-1 is measured by IHC on FFPE specimens. Preliminary studies evaluating HBME-1 on cytologically indeterminate thyroid nodules have shown moderate test performance, with sensitivity 79–94%, specificity 83–94%, PPV 84–94%, and NPV 80–94% [24, 25], but these have not been reproduced in prospective, multicenter, blinded investigations, and thus, validation of HBME-1 staining in such a population of indeterminate thyroid nodules remains to be seen.
CK19
Cytokeratin-19 (CK19) is a cytoskeleton component of epithelial cells that may be upregulated in well-differentiated thyroid cancer [26]. In one study of cytologically indeterminate nodules, most nodules ultimately proven to be papillary thyroid carcinoma stained positive for CK19; however, there was extensive overlap in CK19 expression between benign follicular adenomas and follicular thyroid carcinomas [27]. There are no high-quality data that support the sole use of CK19 as a single molecular marker at this time.
Combined Immunohistochemical Panels
Because of the limitations of individual protein markers, further studies have evaluated the potential for improved diagnostic accuracy when such markers are combined. An assessment of galectin-3 and HBME-1 together showed a PPV and NPV of 76.9% and 96.9% when the markers were concordant in their result [28]. Several studies have utilized the combinations of galectin-3, HBME-1, and CK19 [27, 29–31]. The largest of these evaluating 125 thyroid nodule FNA samples demonstrated an increase in sensitivity from 92% to 97% but a decrease in specificity from 96% to 80% compared to the performance of any marker in isolation [27]. Alternative targets have been included in separate panels of protein markers. One retrospective evaluation combining galectin-3, HBME-1, and CXCR4 showed little improvement compared to HBME-1 testing alone [25]. There have been no robust confirmatory data from large-scale clinical trials to validate these findings.
Mutations and Gene Rearrangements
The discovery of oncogenic mutations and gene rearrangements in thyroid cancers revealed that a significant percentage contain one of a small number of “driver” oncogenic events. Assessment of these mutations and translocations as a “rule-in” test for malignancy for indeterminate thyroid nodules soon followed. As our knowledge of the genetic landscape of thyroid cancer has grown, such molecular tests have evolved from detecting single mutations to assessing combinations of known genetic alterations.
BRAF
The BRAF gene encodes for the protein BRAF, a serine/threonine protein kinase in the MAPK pathway involved in many cellular processes including cell proliferation, differentiation, and apoptosis [32]. The most relevant BRAF mutation seen in thyroid cancers encodes for a mutated protein with a glutamic acid for valine substitution at position 600 (V600E) that causes unregulated kinase activation. BRAFV600E is the most common mutational event in classical PTC and is present in a significant minority of tumors classified as the follicular variant of papillary thyroid carcinoma (fvPTC) [33, 34]. Since this BRAF mutation is not present in benign thyroid disease, detection in FNA material essentially confirms the presence of thyroid cancer. BRAF mutations are less frequent in fvPTC and have a very low prevalence of 1–2% in follicular thyroid carcinoma (FTC). It lacks sufficient sensitivity to be a sole molecular marker for evaluating indeterminate nodules [35], especially because most BRAF-positive papillary thyroid carcinomas will demonstrate cytology that is “positive” or diagnostic of carcinoma (as opposed to cytologically indeterminate). Thyroid cancers harboring the BRAFV600E mutation are associated with a higher risk of extrathyroidal extension, lymph node metastasis, tumor recurrence, and patient mortality, and therefore, its presence may indicate the need for more aggressive initial therapy, though more data are needed to support specific treatment recommendations [35–37].
RAS
The RAS oncogene family is comprised of three genes (H-RAS, K-RAS, and N-RAS) that encode small GTPase proteins involved in signal transduction. Activating mutations of these genes stimulate the MAPK and phosphatidyl-3-inositol pathways that regulate cell growth, proliferation, differentiation, mobility, and survival [38]. RAS mutations are detected in up to 40% of differentiated thyroid cancers, occurring most often in fvPTC, noninvasive follicular thyroid neoplasms with papillary-like nuclear features (NIFT-P), and FTC [30], but are also present in benign thyroid lesions, such as follicular adenomas [39, 40] similarly making RAS status suboptimal as a single molecular marker. It may be that the presence of a RAS mutation portends a risk of malignant transformation, suggesting that resection of a thyroid nodule on the basis of a RAS mutation may still be appropriate [41]. However, data showing the stability and lack of malignant transformation of cytologically benign yet RAS mutation-positive thyroid nodules during long-term sonographic monitoring does not support this [42].
RET/PTC and PAX8/PPARγ Translocations
The RET proto-oncogene encodes a receptor tyrosine kinase that has been found to undergo intrachromosomal gene rearrangements forming fusion genes in differentiated thyroid cancer. The RET/PTC1 and RET/PTC3 gene rearrangements produce constitutively active kinase activity that stimulates the MAPK and phosphatidyl-3-inositol pathways [43]. Because of its relative rarity compared to BRAF or RAS mutations and the overlap with benign thyroid neoplasms, testing for these translocations has limited value in isolation [44].
Another interchromosomal gene rearrangement linking the PAX8 transcription factor gene with the nuclear hormone receptor gene PPARγ produces the PAX8/PPARγ fusion gene, possibly inhibiting the antiproliferative activity of the PPARγ receptor. This gene rearrangement has been detected in 20–40% of follicular thyroid carcinomas and a lower percentage of Hurthle cell carcinomas, as well as follicular adenomas, but has insufficient sensitive or specificity as a molecular marker [45].
Combination Assessment of Oncogenes
Each of these single mutations or translocations has limited diagnostic values, but together genetic alterations in these genes are found in up to 70% of histologically proven thyroid cancers, indicating that if assessed together, better diagnostic discrimination may be achieved. In an early study, BRAF, RAS, RET/PTC, and PAX8/PPARγ genes were assessed in 470 consecutive FNA samples with 55 of indeterminate cytology, showing that while nearly all nodules testing positive were malignant, in some thyroid cancers, no mutations were detected [46]. This indicates potential value as a “rule-in” test to identify cancers but insufficient sensitivity to “rule-out” thyroid cancer.
These and similar results led to continued development and commercialization of a diagnostic test measuring relevant genetic alterations (about 17 in total) in the BRAF, RAS, RET/PTC, and PAX8/PPARγ genes (marketed as miRInform Thyroid, Asuragen, Inc.). Independent investigation and use in the larger clinical context have demonstrated positive and negative predictive values that were more variable and less robust than the initial report [47–52].
The results of the integrated genomic characterization of papillary thyroid cancer as part of The Cancer Genome Atlas (TCGA) expanded our knowledge of the somatic genetic landscape present in PTC and identified the driver mutation/translocation in 96% of PTC tumors studied [53]. Inclusion of these additional oncogenes may help identify more thyroid cancers and improve molecular testing of thyroid nodules. Informed by these data, a diagnostic test was produced using next-generation sequencing techniques to assess point mutations in 13 genes and 42 gene fusions (marketed as ThyroSeq v2). This testing involves additional aspiration material that is separately rinsed in a specific processing solution. Two prospective single-center unblinded studies evaluated its performance on FNA material from cytologically indeterminate thyroid nodules. In 143 consecutive FNA samples with cytology of SFN/FN and available histopathology after resection, ThyroSeq v2 showed a high NPV of 96% (CI 92–100%) and moderate PPV of 83% (CI 72–95%) [54]. In a similar study assessing the performance of ThyroSeq v2 on 98 nodules with cytology of AUS/FLUS, the NPV was similar (97.2% [CI 79–100%]) with again moderate PPV (76.9% [CI 61–93%]) [55]. The demonstration of NPV comparable to benign cytology and PPV similar to that found in the Bethesda V category for which surgically is typically recommended suggests this test may provide both “rule-out” and “rule-in” capability; however, confirmatory data from multicenter, blinded studies or broader clinical use remain lacking. There presently are no published data from a prospective, multicenter, blinded validation of this broader panel, thus limiting any understanding of its clinical validation or clinical utility at this time.
Microarray Expression
Microarray platforms are a method to rapidly determine the expression of all transcribed RNA at relative low cost, allowing for the development of diagnostic microarray panels assessing hundreds of expressed genes. Importantly, computational algorithms are necessary to analyze the expression patterns seen. These techniques may be replaced with next-generation sequencing platforms, but it remains a robust form of expression analysis.
MicroRNA
MicroRNA (miRNA) are 21–22 nucleotide segments of noncoding RNA that play a key role in posttranscriptional gene regulation through complementary binding to messenger RNA that regulates translation and degradation [56]. Overexpression of specific miRNAs has been shown in FTC versus follicular adenoma and PTC versus normal thyroid tissues [57, 58], and initial studies using different miRNA expression profiles on tissue and/or aspiration samples showed overall accuracy from 76 to 90% [59–61]. Combining the relative strengths found in miRNA expression classification and oncogene determination, a two-step testing protocol has been developed and studied (marketed as ThyGenX® and ThyraMIRTM combination testing). 10-miRNA panel and 7-gene mutational panel were assessed on FNA biopsy material from 109 Bethesda III or IV nodules with available surgical pathology and demonstrated 94% [CI 85–98%]) NPV and 74% [CI 58–86%] PPV in a population with a 32% rate of malignancy [52]. Confirmation and validation in a large prospective sample representative of the general nodule population are needed.
RNA Gene Expression Classification
Since the majority of indeterminate nodules are referred for surgical resection and are ultimately proven benign, a novel paradigm was proposed to develop a test to identify benignity, thus effectively “ruling out” malignancy and preventing unnecessary surgery. The design of the Afirma® gene expression classifier (GEC) (marketed by Veracyte, Inc.) was based on this idea. This test measures 167 expressed RNAs from FNA tissue and uses trained computational algorithms to identify a profile highly correlated with a benign diagnosis when applied to indeterminate cytology nodules. After RNA expression profiling is completed, the result classifies a nodule as “benign” or “suspicious” [62]. A prospective, multicenter, blinded validation study evaluated the performance and clinical validity of the GEC in 265 indeterminate nodules greater than 1 cm with available histopathology [63]. In this study population with a rate of malignancy of 32%, NPV and PPV were 93% and 47%, respectively. The NPV for Bethesda V nodules was 85% and has generally been considered insufficient for “rule-out” testing. In contrast, NPV was 95% and 94% for nodules with AUS/FLUS and SFN/FN cytology, respectively, which is considered similar to the malignancy risk of a benign cytology and sufficient to recommend conservative management.
In a subsequent retrospective analysis of 339 indeterminate nodules from five academic centers, patients with an indeterminate nodule and “suspicious” GEC result underwent nodule resection in 121 of 148 (82%) cases, with malignancy confirmed in 53 (44%) nodules. By contrast, 4 of 174 patients with a “benign” GEC result were referred for surgery [64]. Taken together, these results show that when applied to clinical care, the Afirma GEC changes management decisions and reduces the need for diagnostic surgery.
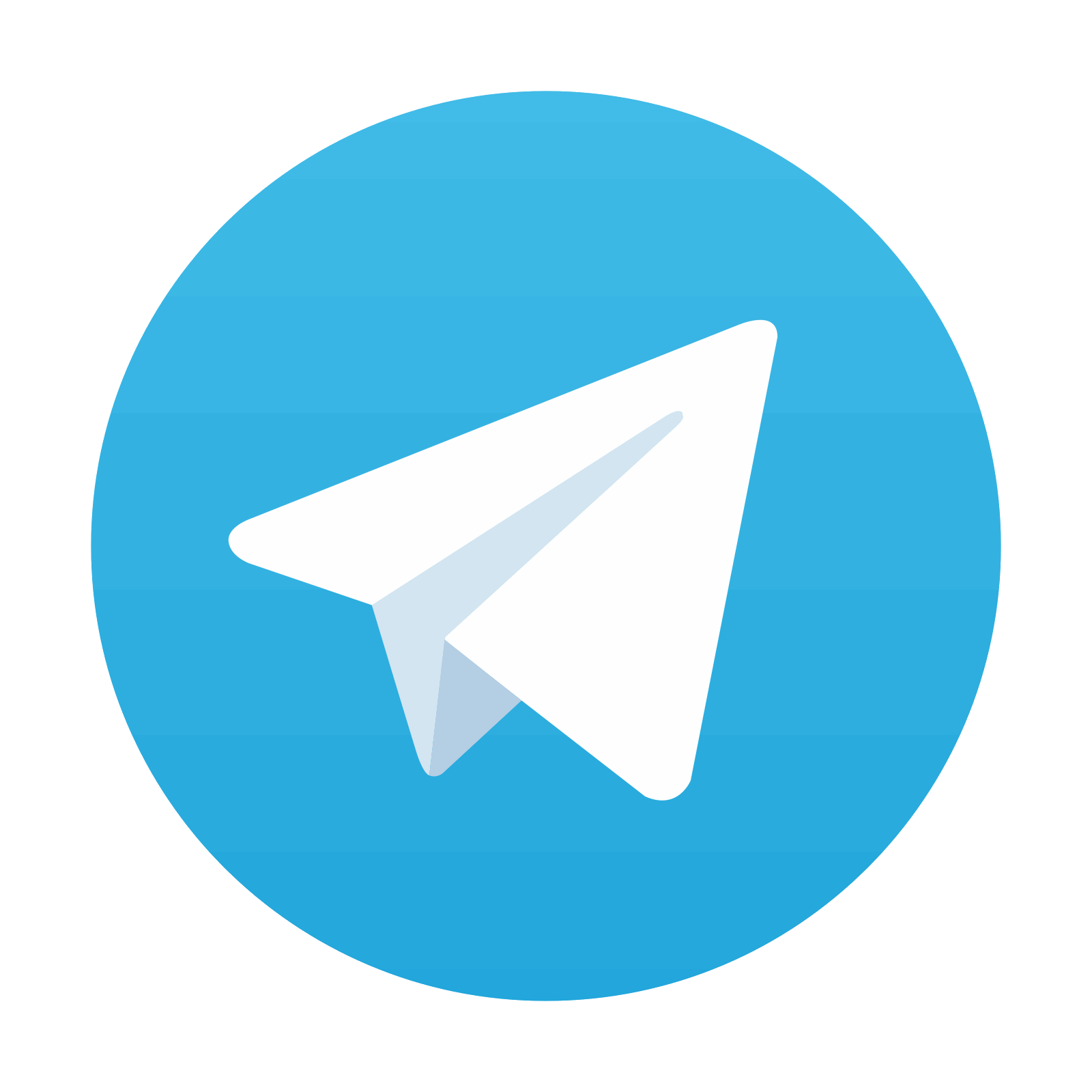
Stay updated, free articles. Join our Telegram channel

Full access? Get Clinical Tree
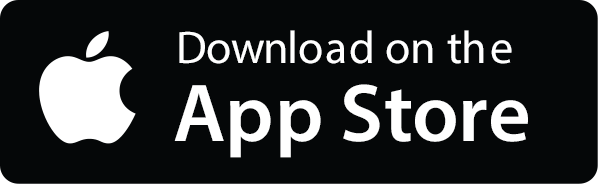
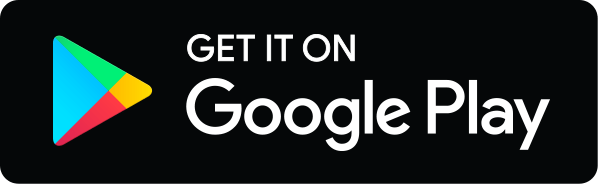