Malformation
Chr region (locus)
Gene
Inheritance pattern(s)
Mutation type(s)
Arterial and capillary anomalies
Arteriovenous malformations (AVM)
?
?
Sporadic
?
Ataxia-telangiectasia (AT)
11q23 (AT1)
ATM
Autosomal recessive
Germline
Capillary malformation-arteriovenous malformation (CM-AVM)
5q14.3 (CMC1)
RASA1
Autosomal dominant
Germline, somatic 2nd hit
Cerebral cavernous malformation (CCM)
7q21.2 (CCM1)
KRIT1
Autosomal dominant
Germline, somatic 2nd hit
7p13 (CCM2)
CCM2 / malcavernin
Autosomal dominant
Germline, somatic 2nd hit
3q26.1 (CCM3)
PDCD10
Autosomal dominant
Germline, somatic 2nd hit
3q26.3–27.2 (CCM4)
?
Autosomal dominant
?
Hereditary hemorrhagic telangiectasia or Rendu-Osler-Weber (HHT/ROW)
9q33–34 (HHT1)
ENG
Autosomal dominant
Germline
12q11–14 (HHT2)
ALK1
Autosomal dominant
Germline
5q31.3–32 (HHT3)
?
Autosomal dominant
?
7p14 (HHT4)
?
Autosomal dominant
?
Juvenile polyposis-HHT (JPHT)
18q21.1 (JPHT)
SMAD4 / MADH4
Autosomal dominant
Germline
HHT-like
10q11.22
GDF2 / BMP9
?
Germline
Isolated capillary malformation (CM)
1q21.2
GNAQ
Sporadic
Somatic mosaic
Sturge-Weber syndrome (SWS)
1q21.2
GNAQ
Sporadic
Somatic mosaic
Microcephaly-capillary malformation syndrome (MIC CAP)
2p13.1
STAMBP
Autosomal recessive
Germline
Venous anomalies
Glomuvenous malformation (GVM)
1p22.1 (VMGLOM)
GLMN
Autosomal dominant
Germline, somatic 2nd hit
Cutaneomucosal venous malformation (VMCM)
9p21 (VMCM1)
TEK / TIE2
Autosomal dominant
Germline, somatic 2nd hit
Unifocal sporadic venous malformation (VM)
9p21
TEK / TIE2
Sporadic
Somatic mosaic
Blue rubber bleb nevus syndrome (BRBN)
9p21
TEK / TIE2
Sporadic
Somatic mosaic
Lymphatic anomalies
Lymphatic malformation (LM)
?
?
Sporadic
?
Primary lymphedema (LE)
Primary congenital LE (Nonne-Milroy disease)
5q35.3 (PCL1)
FLT4 / VEGFR3
Autosomal dominant
Germline
5q35.3
FLT4 / VEGFR3
Sporadic
De novo germline
5q35.3
FLT4 / VEGFR3
Autosomal recessive
Germline
4q34.3
VEGFC
Autosomal dominant
Germline
LE-choanal atresia
1q32.2
PTPN14
Autosomal recessive
Germline
LE-lymphangiectasia-mental retardation (Hennekam syndrome)
18q21.32
CCBE1
Autosomal recessive
Germline
LE-distichiasis/ptosis/yellow nail
16q24.3 (LD)
FOXC2
Autosomal dominant
Germline
Hypotrichosis-LE-telangiectasia (HLT)
20q13.33 (HLT)
SOX18
Autosomal dominant
Germline
20q13.33
SOX18
Autosomal recessive
Germline
LE-myelodysplasia (Emberger syndrome)
3q21.3
GATA2
Autosomal dominant
Germline
Four-limb LE
1q42.13
GJC2 / CX47
Autosomal dominant
Germline
Oculodentodigital syndrome-LE
6q22.31
GJA1 / CX43
Autosomal dominant
Germline
LE-microcephaly-chorioretinopathy (MLCRD)
10q24.1
KIF11 / EG5
Autosomal dominant
Germline
Osteoporosis-LE-anhidrotic ectodermal-dysplasia-immunodeficiency (OLEDAID)
Xq28 (IP2)
NEMO / IKBKG
X-linked recessive
Germline
LE-hereditary cholestasis (Aagenaes syndrome)
15q (LCS1)
?
Autosomal recessive
?
Combined vascular malformations
CLOVES
3q26.3
PIK3CA
Sporadic
Somatic mosaic
Klippel-Trenaunay syndrome (KTS)
5q13.3
AGGF1 / VG5Q
Sporadic
Germline
PTEN hamartoma tumor syndrome (PHTS) (including Cowden, Bannayan-Riley-Ruvalcaba, and “Proteus” syndromes)
10q23.3 (PHTS)
PTEN
Autosomal dominant
Germline
Cowden, Cowden-like syndrome
3q26.3
PIK3CA
Autosomal dominant
Germline
14q32.3
AKT1
Autosomal dominant
Germline
“Proteus” syndrome
14q32.3
AKT1
Sporadic
Somatic mosaic
Congenital lipomatous overgrowth with vascular, epidermal, and skeletal anomalies (CLOVES)
3q26.3
PIK3CA
Sporadic
Somatic mosaic
Major advances have been made in identifying the genetic bases of several vascular malformations (Table 2.1; reviewed in [8]). This has allowed for more accurate “molecular” diagnosis and therefore appropriate treatment of diseases with overlapping symptoms. In addition, knowledge of the genetic bases of pathology has led to insights into the pathways and mechanisms dysregulated downstream, which may be targeted for therapy.
Capillary Malformation (CM)
CMs (OMIM 163000) are the most frequently occurring vascular malformations, with an incidence of about 0.3 % in the population. They appear flat, reddish to purple, and irregular, giving them the name “port-wine stain.” Sometimes quite extensive, they are most frequently found in the face and neck regions but can also occur on the trunk and limbs. Histologically, they consist of dilated capillary-like channels, usually within the dermis. CMs are predominantly sporadic in nature, although rare autosomal-dominant hereditary forms, such as capillary malformation-arteriovenous malformation (CM-AVMs), have been observed in some families.
Capillary Malformation-Arteriovenous Malformation (CM-AVM)
CM-AVM (OMIM 608354) is characterized by small, multifocal, pink-red CMs, often with a pale halo. In about a third of cases, these are accompanied by AVMs, AVFs, or Parkes Weber syndrome (PWS; OMIM 608355), characterized by large cutaneous stains on an extremity, which also shows soft tissue and skeletal hypertrophy, and AV micro-fistulas. Mutations in p120RasGAP (RASA1), which encodes a Ras GTPase-activating protein (RasGAP), have been identified to cause the autosomal-dominant disorder [9–11]. The changes primarily cause premature truncation and loss of function of the protein. The reduced penetrance and phenotypic heterogeneity observed in affected families suggest that a somatic second hit may be required to locally inactivate the second allele of the gene. In support of this hypothesis, Revencu et al. identified a somatic loss of chromosome 5q that includes RASA1, in a neurofibroma that developed (during adulthood) on a congenital Parkes Weber lesion, in one individual with a germ line RASA1 mutation. Two somatic hits to NF1 were in addition identified in the tissue [11]. Loss of p120RasGAP would be expected to lead to chronic Ras activation. RASA1 may also be able to act in a Ras-independent manner through its interaction with p190RhoGAP, which seems to play a role in cell movement [12, 13], or through its ability to promote pro-survival Akt activation [14]. While mice that are heterozygous for a deletion of Rasa1 appear normal, homozygous deletion of the gene is embryonic lethal (at E10.5), due to defects in the development of the blood vasculature and increased apoptosis [15]. In support of the second hit hypothesis, mice that are mosaic for Rasa1+/− and Rasa1−/− cells develop localized vascular defects [15]. Homozygous deletion of Rasa1, whether ubiquitous or lymphatic endothelial cell (LEC)-specific, results in defects in the lymphatic rather than the blood vasculature when induced in adult mice; lymphatic defects have also been observed in certain patients with PWS and CM-AVM [16].
Isolated and Syndromic Sporadic Capillary Malformations
The presence of deeper vascular anomalies in association with CMs is also observed in sporadic syndromes, such as Sturge-Weber syndrome (SWS, OMIM 185300; characterized in addition by leptomeningeal and ocular vascular malformations) and Klippel-Trenaunay syndrome (KTS, OMIM 149000; in which an affected extremity shows, in addition, hypertrophy of soft tissue and bone, varicose veins, deep venous defects, and venolymphatic malformation). While its symptoms overlap with those of PWS, KTS does not appear to be part of the spectrum of phenotypes mediated by germline mutations in RASA1 [17]. The genetic basis of KTS is not known, although a balanced translocation ((5;11)(q13.3;p15.1)) with a breakpoint in the AGGF1 (angiogenic factor with G patch and FHA, previously VG5Q) gene promoter has been identified in one patient [18, 19]. AGGF1, upregulation of which is associated with KT, plays a role in endothelial cell proliferation and in the establishment of venous identity in zebra fish, which may account for the occurrence of venous malformations in KT [20]. The somewhat overlapping CLOVES syndrome, characterized by congenital lipomatous overgrowth, vascular malformations, and epidermal nevi (OMIM 612918), is caused by somatic activating mutations in PIK3CA, the p110 subunit of PI3kinase [21]; some proportion of Klippel-Trenaunay syndrome likely has a similar basis.
The use of whole-genome sequencing comparing paired affected and normal tissues from patients with SWS recently resulted in the identification of a somatic mutation in GNAQ (guanine nucleotide-binding protein G (q) subunit alpha; p.Arg183Gln) [22]. Targeted deep sequencing revealed that this change was present in affected (skin or brain) tissues from 88 % of SWS patients and 92 % of patients with isolated CM. Mutant allele frequency ranged from 1 to 18 %, highlighting the importance of high depth of coverage in the discovery of mosaic mutations. The change was also present at a frequency of 1–1.5 % in 5 of 669 control blood samples, hypothesized to correlate with the incidence of CM in the general population. The pathogenic effects of this mutation, which induces mild activation of p42/44 MAPK but not JNK, p38, or AKT in vitro, remain to be elucidated [22].
Whole-exome sequencing revealed recessive germline mutations in STAMBP in microcephaly-capillary malformation syndrome (OMIM 614261), in which cortical atrophy, epilepsy, and profound developmental delay are accompanied by multiple cutaneous capillary malformations [23]. STAMBP is a deubiquitinating isopeptidase that plays a role in endosomal sorting and trafficking of ubiquitinated proteins. Reduced levels of STAMBP in patient cells are associated with an accumulation of ubiquitinated proteins and activation of the RAS-MAPK and PI3K-Akt pathways [23].
Cerebral Cavernous Malformation (CCM)
CCM (OMIM 116860) occurs as a sporadic or autosomal-dominantly inherited cerebral vascular anomaly with incomplete penetrance. It is characterized by enlarged capillary-like channels lined with a single layer of endothelial cells lacking tight junctions, within a dense collagenous matrix with no intervening brain parenchyma. Lesions occur predominantly in the brain but have also been observed in the retina, spinal cord, and skin, as crimson, irregular hyperkeratotic cutaneous capillary-venous malformations (HCCVMs). Symptoms of CCM include headaches, seizures, and cerebral hemorrhage, but while the anomaly has an estimated prevalence of 0.5 % based on large-scale magnetic resonance scanning (MRI) and autopsies, the clinical incidence is much lower, since it is often asymptomatic. Truncating mutations and/or large genomic deletions in three different genes have so far been identified to cause the disease, CCM1 (KRIT1 [24, 25]), CCM2 (malcavernin or MGC4607; [26]), and CCM3 (PDCD10; [27]), with a fourth locus suggested on 3q26.3–27.2 [28]. Cutaneous HCCVMs are mostly due to mutations in CCM1/KRIT1 [29, 30]. It has become evident that somatic “2nd-hit” mutations that are allelic to the inherited change and cause complete localized loss of function cause lesion formation [31, 32].
CCM 1, CCM2, and CCM3 have been found to interact with each other [33, 34] and form a complex with the kinase MEKK3, the small GTPase RAC1, and integrin cytoplasmic domain-associated protein-1 α (ICAP-1α), which function in integrin and the MAP-kinase pathway [35, 36]. CCM1 also interacts with Rap1, which stabilizes EC junctions [37, 38] and promotes DLL4-Notch signaling through Akt while inhibiting ERK [39]. In vitro, knockdown of any of the three CCM proteins decreases tube formation and invasion by ECs [40, 41]. In vivo, CCM1 −/− mice die at mid-gestation with abnormal differentiation of arteries. This raises the possibility that it is important in arterial development, failing which, a default venous phenotype results in the CCM sinusoids [42]. The absence of the tumor suppressor in Trp53−/− Ccm1+/− mice results in the formation of vascular lesions in the brain [43]. CCM1 +/− mice, on the other hand, fail to show any CCM-like lesions. Similarly, homozygous CCM2 deletion causes embryonic lethality at mid-gestation, while heterozygous mice appear healthy [44]. Morpholino-mediated inactivation of the CCM genes results in vessel dilation and embryonic death in zebra fish [45, 46].
Venous Anomalies
Venous anomalies [6] are a group of slow-flow malformations, with an estimated incidence of about 1 in 10,000, primarily comprising of venous malformations (VMs), which account for about 95 % of patients. These are primarily sporadic in nature (sporadic venous malformation or VM) but can also occur in the autosomal-dominantly inherited form, cutaneomucosal venous malformation (VMCM; about 1 %). About 5 % of venous anomalies are glomuvenous malformations (GVM), typically dominantly inherited.
Glomuvenous Malformation (GVM)
GVMs (OMIM 138000) consist of raised, nodular, pink to blue-purple cutaneous lesions, often multifocal and hyperkeratotic. Histologically, they appear as distended venous channels, their hallmark being the presence of rounded “glomus” mural cells, lacking the spindle shape of normal vSMCs as well as certain developmental markers. Unlike VMs, these malformations do not infiltrate deeply, are often painful upon palpation, cannot be completely emptied upon compression, and do not cause the coagulation abnormalities that can be observed in VMs [47, 48].
Most GVMs (about 70 %) are autosomal-dominantly inherited, showing incomplete penetrance that increases with age and wide heterogeneity even among members of the same family. Mutations in the glomulin (GLMN) gene mediate the anomaly [49], with >30 loss-of-function changes identified in >100 families. 11 of these mutations account for >80 % of families, allowing for relatively efficient screening of the gene [50, 51]. The mutations occur across the entire gene, with no genotype-phenotype correlation. Glomus cells in GVMs lack expression of GLMN, explained by the fact that somatic 2nd hits to gene have been identified in the majority of tissues [49, 52]. Interestingly, uniparental isodisomy of the entire 1p arm, which results in the replacement of the normal allele with a second defective copy of GLMN, is the predominant somatic change [52].
GLMN is observed primarily in vascular SMCs, which fail to differentiate appropriately in its absence [53]. GLMN has been found to be able to bind FKBP12, which in turn impairs TGF-β signaling by binding TGF-β RI and inhibiting its phosphorylation by TGF-β RII [54, 55]. Thus, by competing for binding with FKBP12, GLMN could play a role in promoting TGF-β signaling, known to be important in SMC development. GLMN can also bind the inactive form of c-MET [56], the tyrosine kinase receptor for hepatocyte growth factor (HGF), which participates in cell proliferation, tube formation by ECs, and SMC migration [57]. Upon ligand binding and phosphorylation of c-MET by HGF, GLMN undergoes phosphorylation and is released, accompanied by activation of the kinase p70S6K and its downstream target PI3-K [56]. TGF-β regulates the expression of HGF as well as c-MET [58, 59], and thus, GLMN may act in a coordinated manner in the two pathways.
GLMN also binds Cul7 and Rbx1, subunits of the cullin RING ligase 1 (CRL1) complex [60]. It inhibits the E3 ubiquitin ligase activity of the complex, which is responsible for the recruitment and transfer of ubiquitin to protein substrates destined for ubiquitination and degradation [61]. Lack of GLMN results in a decrease in Fbw7, a substrate receptor for CRL1 that targets cyclin E and c-Myc for degradation, therefore leading to increased levels of these pro-oncogenic proteins [62]. This would suggest that their increase contributes to GVM, possibly accounting for the accumulation of large numbers of rounded GLMN-negative glomus cells.
Venous Malformation (VM)
VMs consist of expanded venous-like channels, with a thin, flat endothelial cell layer, and sparse, patchy areas of vascular smooth muscle cells (vSMCs). They are usually located in the skin or mucosa but can infiltrate deeper into the muscles, nerves, bones, and viscera [47, 48]. VMs are congenital and appear flat or raised and bluish purple, ranging from small blebs to extensive lesions. Localized intravascular coagulation (LIC) in VMs causes increased D-dimer products in 40 % of patients, and soluble fibrin and decreased fibrinogen are associated with more extreme lesions [63, 64]. VMs can be part of combined lesions in the context of more complex syndromes such as KTS, described earlier, or occur along the GI tract, as seen in the blue rubber bleb nevus syndrome.
Cutaneomucosal Venous Malformation (VMCM)
The autosomal-dominant VMCM (OMIM 600195) is characterized by multiple small, raised cutaneous lesions that seldom infiltrate deeply. The presence of oral mucosal lesions along with family history is typical, although the anomaly can often be asymptomatic. Activating mutations in TIE2 (TEK), the endothelial cell-specific tyrosine kinase receptor for the angiopoietins (ANG–1, ANG–2, and ANG–4 in human or ANG–3 in mouse; [65–67]), have been found to mediate the disease [68–70].
TIE2 plays an important role in microvascular sprouting, maturity, stability, and integrity (reviewed in [71]). Upon ligand binding with multimeric forms of its activating ligand ANG–1, TIE2 forms homodimers and undergoes phosphorylation. ANG-2, on the other hand, is generally considered to be an antagonist; its effects however seem to be strongly context dependent. TIE2 has also been found to heterodimerize with TIE1, a close paralogue, with the latter being able to modulate TIE2 signaling [72, 73].
Deletions of the TIE1, TIE2, or ANG–1 or ANG–2 genes all result in severe defects in vascular development and embryonic lethality in mice. Tie2 −/− mice have defects in primary capillary plexus formation due to a deficiency of ECs, as well as incomplete cardiac development, and hemorrhage [74, 75]. While Ang1 −/− mice show a similar, overlapping phenotype [65], Ang2-overexpressing transgenics are similar to TIE2-defective mice [66].
TIE2 activation results in the phosphorylation and activation of a variety of downstream signaling intermediates, including PI3K and Akt, which have an anti-apoptotic effect [76]. PI3K can also activate focal adhesion kinase (FAK) and the endothelial-specific nitric oxide synthase (eNOS), which participate in cell migration and sprouting. Other signaling molecules that can interact with TIE2 include Dok-R, which can affect cell migration, Grb2, 7, and 14, and the protein tyrosine phosphatase Shp2, which can in turn associate with FAK and activate the mitogen activated protein kinase (MAPK) pathway, influencing EC migration and survival [77, 78]. By interacting with ABIN-2, an inhibitor of NFkB, TIE2 may exert anti-inflammatory effects on ECs, reducing leukocyte extravasation and promoting vessel stability and integrity [79].
Eight TIE2 mutations have been identified in VMCM. Of these, p.Arg849Trp (R849W) is recurrent, having been reported in 10/17 families [68–70, 80]. All VMCM-associated mutations are intracellular, located in the 1st tyrosine kinase, kinase insert, and C-terminal tail domains of the receptor. They cause ligand-independent TIE2 hyperphosphorylation when overexpressed in vitro [68–70]. The R849W mutation has been found to have a strong Akt and ShcA-dependent anti-apoptotic effect [81, 82] but does not cause proliferation. It also causes hyperphosphorylation of the transcription factor STAT1 in human umbilical vein endothelial cells (HUVECs) in vitro, inhibiting VEGF-A-mediated angiogenesis by competing with STAT3 for its promoter targets [83, 84]. A somatic 2nd-hit mutation has been identified in one patient with the inherited R849W change, indicating that VMCM, like CCM and GVM, has a paradominant mode of inheritance [85]. The somatic mutation deletes most of the extracellular Ig2 ligand-binding domain of the wild-type allele, resulting in a loss of its function. This would suggest that the presence of the wild-type receptor exerts an as-yet-undefined protective or competitive effect on the mutant receptor.
Sporadic Venous Malformation (VM)
Somatic mutations in TIE2 also cause common sporadic VM, characterized by the presence of single large lesions, although multifocal lesions can in rare cases occur [85, 86]. A recurrent p.Leu914Phe (L914F) has been identified in affected tissues from >75 % of patients. Almost all of the remainder carry double mutations in cis (on the same allele), commonly combining modifications of p.Tyr897 (to Cys, His, Phe, or Ser) with a second change in the kinase insert or C-terminal tail domains [85, 86]. All of the mutations cause ligand-independent receptor phosphorylation when overexpressed. This is also true for certain mutations that cause premature truncation of TIE2 in the C-terminal tail region, which is otherwise held in an inhibitory conformation. The relatively strong phosphorylation caused by L914F as compared to R849W is echoed by a stronger effect on downstream AKT and STAT1 phosphorylation [87].
In addition to a pro-survival effect on ECs, Akt phosphorylation causes phosphorylation and inactivation of transcription factors FOXO1 and 4. The major vascular smooth muscle cell-attractant PDGFB is among the FOXO1 target genes downregulated as a result in vitro as well as in vivo around malformed veins in patient tissues [87]. This is hypothesized to account for the paucity and irregularity of the mural cell layer observed in VM. Angpt1 ligand activation of TIE2 similarly results in downregulation of PDGFB, suggesting that it does not play a role in the recruitment of vSMCs to nascent vessels, but in the stabilization and homeostasis of established ones [87].
Blue Rubber Bleb Nevus Syndrome (BRBN)
Blue rubber bleb nevus syndrome (OMIM 112200) is a rare congenital disorder characterized by the presence of a few to hundreds of cutaneous and internal VMs, with gastrointestinal lesions being pathognomonic. Somatic activating mutations in the intracellular region of the TIE2 receptor have been identified in the lesions from a large (>80 %) percentage of patients with BRBN [88]. Double (cis) mutations seem to predispose to multifocal lesions and are significantly enriched in BRBN as compared to common unifocal VM. As in rare multifocal sporadic VM, multiple lesions from the same patient bear the same mutations, undetected in blood, suggesting they arise from common cellular progenitors [88].
Lymphatic Malformations (LM)
LMs are defects in lymphatic structure characterized by no-flow microcystic or macrocystic lesions, lacking connections with the lymphatic vessel system. They consist of dilated channels lined by a flat endothelium, filled with a clear fluid, but no blood except when intracystic bleeding occurs. This can cause the malformations, which otherwise appear to be of normal skin color, to appear bluish purple to red. LMs can be secondary, due to infection or trauma, or primary, in which case they are usually congenital or appear in infancy, growing with the individual. The etiology of these sporadic malformations remains unknown, with major controllers of lymphatic development, such as those implicated in lymphedema, remaining good candidates.
Lymphedema
Lymphedema is a diffuse lymphatic anomaly, characterized by swelling caused by fluid accumulation due to defective lymphatic drainage, usually of the lower extremities. Lymphedema can be primary or secondary due to infection or trauma. Primary lymphedema, which can occur in sporadic as well as incompletely penetrant, inherited forms, can either be isolated or form part of syndromes, such as Turner syndrome (short stature, estrogen deficiency, and cardiac and other problems caused by partial or complete chromosome X-monosomy) and Noonan syndrome (short stature, facial dysmorphism, and cardiac defects, for which gain-of-function mutations in genes in the MAPK kinase pathway including PTPN11, KRAS, SOS1, and RAF1 have been identified). Primary lymphedema is further subdivided according to age at onset of the disease into: type I congenital lymphedema, usually present at birth, which can sometimes cause prenatal pleural effusion and hydrops fetalis; type II praecox lymphedema or Meige disease (OMIM 153200), which occurs between puberty and 35 years of age; and lymphedema tarda, which occurs later in life.
Eleven genes have been identified to cause different forms of lymphedema, several of which modulate, or respond to, VEGFR3 signaling [89]. These account for only a small proportion of the disease, however, an indication of the high level of genetic heterogeneity underlying the phenotype when defined broadly. An autosomal-dominant inherited form of congenital primary lymphedema called Nonne-Milroy disease (OMIM 153100) can be caused by phosphorylation-inhibitory mutations in the tyrosine kinase domain of the vascular endothelial growth factor receptor 3 (VEGFR–3 or FLT–4) [90, 91]. De novo mutations in the gene have been identified in sporadic cases [92], and a homozygous change creating a hypomorphic allele was identified in a recessive case [93]. VEGFR3 expression is found to be elevated in lymphatic ECs; Vegfr3 mutant (Chy) and receptor-deficient mice show a very similar phenotype to the human, supporting its role in lymphangiogenesis and lymphedema pathogenesis [94, 95].
Exome sequencing identified a small frame-shifting deletion in the VEGFR3 ligand VEGFC in one family with a phenotype similar to that associated with VEGFR3 mutations [96]. Chy–3 mice, which are lack one copy of the VEGFC gene, have chylous ascites and lymphedema. Homozygous knockout mice are unable to form lymphatics, while heterozygotes show lymphatic hypoplasia [97, 98].
A homozygous frame-shifting deletion of exon 7 has been identified in PTPN14, a non-receptor type protein tyrosine phosphatase, in a family with lymphedema with choanal atresia (OMIM 608911) [99]. PTPN14 was shown to interact with VEGFR3, and complex formation was increased by stimulation with VEGFC. Mice lacking the phosphatase develop lymphatic hyperplasia and lymphedema by about 5 months of age [99].
Homozygous and compound-heterozygous mutations in collagen and calcium-binding protein with EGF domain 1 (CCBE1) cause Hennekam syndrome (lymphedema, lymphangiectasia-mental retardation; OMIM 235510) [100–102]. CCBE1 has been found to amplify VEGFC-mediated lymphangiogenesis [103]. Both mouse and zebra fish embryos lacking the protein develop edema due to the failure to develop lymphatic vessels [103, 104].
Mutations in the transcription factors FOXC2, SOX18, and GATA2 have been implicated in forms of primary lymphedema. Loss of function and missense mutations in FOXC2 can cause inherited lymphedema associated with distichiasis (OMIM 153400), ptosis (OMIM 153000), and yellow nail syndrome (OMIM 153300) [105–107]. Foxc2-deficient mice have abnormally patterned lymphatics with increased pericyte coverage and fail to develop lymphatic valves [108].
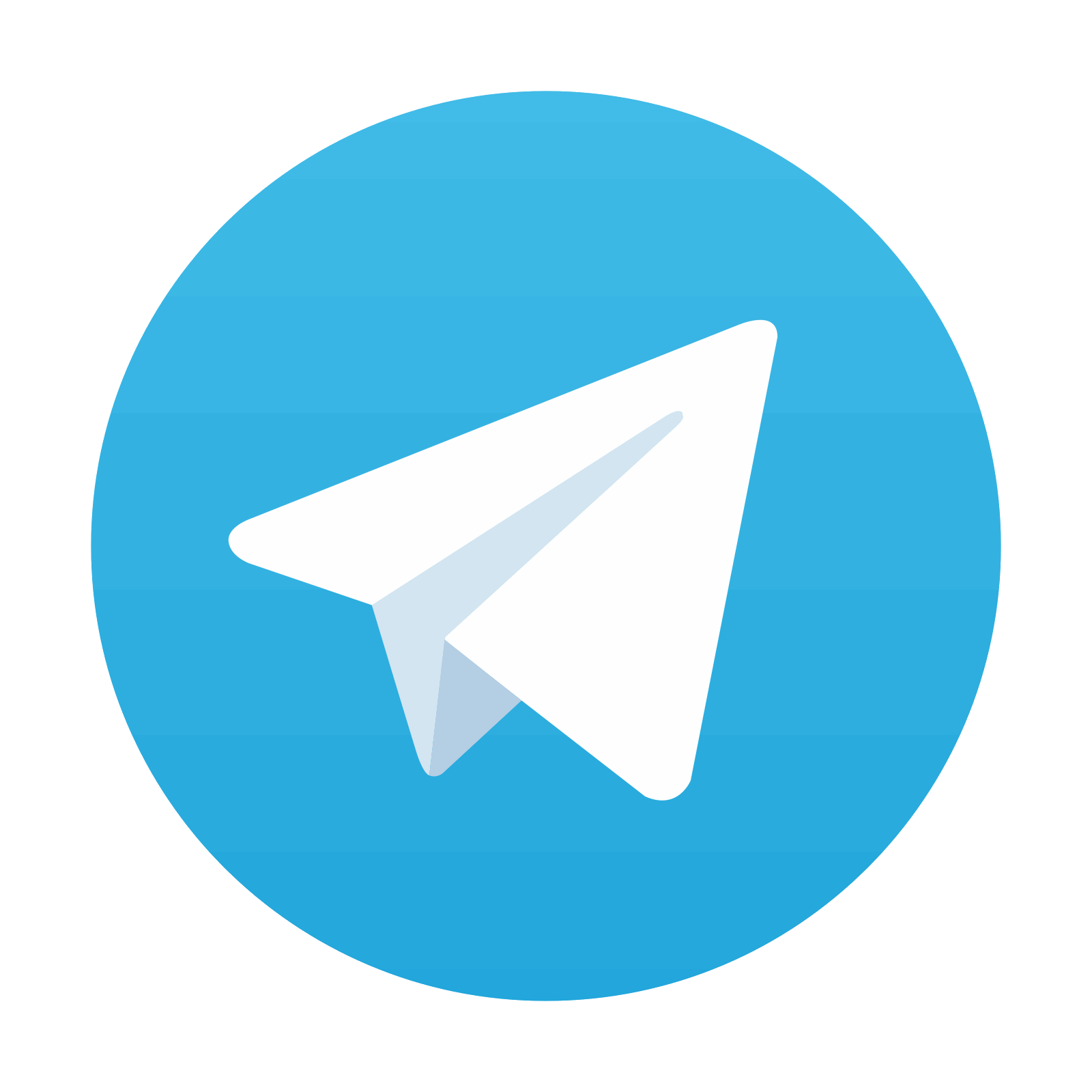
Stay updated, free articles. Join our Telegram channel

Full access? Get Clinical Tree
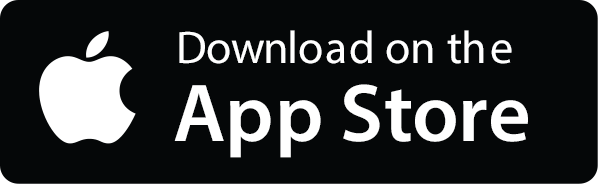
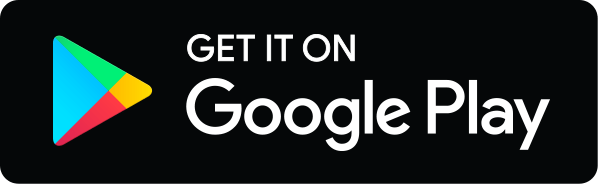