Tumor type
miRNAs
Comments
Reference(s)
Brain, GBM
miR-21, miR-221, miR-222, (up)
miR-21 upregulated in all high-grade tumors
Breast cancer
miR-21, miR-155b (up)
miR-125b, miR-145 (down)
Expression levels correlate with biopathological features and proliferation index
[59]
Cervical cancer
miR-21 (up)
miR-143 (down)
[103]
Cholangiocarcinoma
miR-21, miR-141, miR-200b (up)
[103]
Chronic lymphocytic leukemia (CLL)
miR-15a, miR-16-1 (down)
miR-29a-2, miR-29b-2, miR-29c (down)
Downregulated in 68 % of CLL patients
[59]
Colorectal cancer
miR-143, miR-145, miR133b (down)
miR-31, miR-135b, miR-96, miR-183 (up)
miR-31 expression level correlates with cancer stage
[68]
Hepatocellular cancer
miR-18, miR-224 (up)
miR-199a, miR-195, miR-200a, miR-125a, miR-122 (down)
Higher miR-18 expression level is inversely correlated with tumor differentiation
[104]
Lung, NSCLC
let-7 (down)
miR-21, miR-155 (up)
Low let-7 and high miR-155 expression levels correlate with poor survival
Lymphoma (B cells)
miR-155 (up)
Pancreatic cancer
miR-21, miR-107 (up)
miR-155, miR-103 (down)
Overexpression of miR-21 correlates with high proliferation index
[71]
Prostate cancer
let-7, miR-125b, miR-143, miR-145 (down)
Profile can separate between androgen positive versus androgen negative tumor
[72]
Testis, germ cell tumors
miR-372, miR-373 (up)
[105]
Thyroid, papillary ca (PTC)
miR-221, miR-222, miR-146b (up)
[41]
Thyroid, anaplastic ca (ATC)
miR-125b, miR-26a (down)
miRNA profile can separate ATC from normal and (PTC)
[67]
For years researchers were perplexed as to how the BIC RNA could consistently produce lymphomas and induce the overexpression of the MYC oncogene [39]. After analyzing the phylogenetically conserved region in BIC, Metzler et al. found that a high homology region in fact codes for the pri-miRNA of miR-155 [40]. Further studies by this group showed that miR-155 expression is upregulated 100-fold in pediatric Burkitt lymphoma, in addition to Hodgkin’s lymphoma and primary mediastinal and certain subsets of large B-cell lymphoma [41, 42]. The etiological role of miR-155 in lymphoma development was further established by Costinean et al. from their work with transgenic mice carrying a miR-155 transgene with B-cell targeted expression [43]. The transgenic mice from this experiment developed preleukemic pre-B-cell proliferation at as early as 3 weeks of age and developed high-grade B-cell malignancies at 6 months of age. However, the oncogenic role of miR-155 is not limited to B-cell lymphoma as other studies have reported its upregulation in breast, lung, colon, and thyroid cancers [44, 45].
Another potentially oncogenic cluster of miRNAs is the polycistron miR-17-92, containing seven miRNAs: miR-17-5p, miR-17-3p, miR18a, miR-19a, miR-19b-1, and miR-92-1. This cluster was found to be overexpressed in samples of many kinds of lymphoma samples compared with normal tissues [46]. Using a mouse B-cell lymphoma model, He et al. found that the miR-17-92 cluster in conjunction with MYC accelerated tumor development [46]. At the same time, O’Donnell et al. independently identified the mir-17-92 cluster as a group of potentially cancer-related genes [47]. The group found that MYC activates the expression of this cluster and two miRNAs in this cluster, miR-17-5p and miR-20a, act to downregulate the transcription factor E2F1, which is also a MYC transcriptional target, promoting cell cycle progression. This feedback cycle allows MYC to control the accumulation of E2F1 and drive the cell toward proliferation, since E2F1 is known to sensitize cells to apoptosis at high concentrations.
Various studies repo rted that miRNAs are also aberrantly expressed in brain tumors. During the analysis of a global expression level of 245 miRNAs in glioblastoma multiforme (GBM), Ciafre et al. observed that miR-221 was highly upregulated [48]. In recent work, Gillies et al. reported that miR-221 and miR-222 directly target p27Kip1, a key negative regulator of the cell cycle, in glioblastoma [49]. In another study, Chan et al. also observed that miR-21 was strongly overexpressed (5-fold to 100-fold) in highly malignant glioblastoma tumor tissues [50]. The investigators also found that knockdown of miR-21 in cultured glioblastoma cells activated caspases and resulted in more cell death by an apoptotic pathway. In a comprehensive analysis of miRNA expression signature of human solid tumors, Volinia et al. found that overexpression of miR-21 is shared by all six solid tumors studied, including breast, colon, lung, pancreas, prostate, and stomach [45]. This finding suggests that miR-21 plays an important regulatory role in a pathway shared by all of these cancer types. Despite the evidence that mutant miRNAs cause cancer, little is known about the mechanism of these miRNAs and how they affect cancer progression.
17.2.2 let-7 Regulates and Suppresses Activated RAS in C. elegans
Our lab recently showed that let-7 regulates let-60, the C. elegans RAS oncogene homologue [18, 19]. let-60/RAS is best understood for its role in C. elegans vulval development [51]. During vulval development, a LIN-3/EGF signal from the anchor cell is received by the LET-23/EGFR receptor on the vulval precursor cells (VPCs), P5.p, P6.p, and P7.p. The P6.p cell receives the most LIN-3 and activates a RAS/MAPK signal transduction pathway to adopt the primary induced (1°) fate. P5.p and P7.p receive less LIN-3 and also receive a second, lateral signal (involving LIN-12/Notch) from the 1° cell that induces them to the 2° fate. let-60/RAS contains multiple let-7 complementary sites in its 3′UTR, and let-60(lf) suppresses let-7 mutants. We found that the let-60/RAS 3′UTR is sufficient to restrict let-60/RAS expression only to the P6.p. In a normal animal, a let-7 family member, mir-84, is expressed in all the VPCs except the primary induced cell, and represses let-60/ras expression in these cells. In C. elegans animals carrying activating let-60/RAS mutations more than one VPC is induced to differentiate into the 1° cell fate, leading to excess vulvae (thought of as vulval tumors), called a multivulva phenotype (Muv). We found that overexpression of mir-84 suppres sed the Muv phenotype of activating mutations in let-60/RAS.
17.2.3 let-7 Negatively Regulates Human RAS and Other Oncogenes
Many activating mutations in the human NRAS, KRAS, and HRAS genes alter the same amino acid affected by the C. elegans let-60/RAS activating mutation. Since C. elegans RAS is a target of let-7, and both human RAS [36, 52, 53] and let-7 [18, 54] are implicated in lung cancer, our lab oratory tested the hypothesis that human RAS is also a target of let-7. Our results show that all three RAS oncogenes have let-7 complementary sites in their 3′UTRs and that let-7 can reduce their expression in a 3′UTR-dependent manner [18] in vitro. We next evaluated the relationship between let-7 and RAS in lung tumors and found that let-7 was consistently downregulated in lung cancers relative to normal adjacent tissue (NAT), mirroring a recently published report about let-7 [54]. We also found that in lung cancer tissue, where let-7 levels are low, RAS protein levels are elevated relative to the NAT. Supporting the significance of our findings, a correlation was found where lung cancer patients with the least let-7 expression were most likely to die from lung cancer [54, 55]. A similar correlation was seen when just the lung adenocarcinoma patients were followed [54].
let-7 has also been recently reported to be involved in the regulation of two other oncogenes, MYC and HMGA2. Sampson et al. showed that overexpression of pre-let-7a in a Burkitt lymphoma cell line led to downregulation of MYC expression and reverted MYC-induced growth in this cell line [56]. Interestingly, let-7a overexpression not only resulted in a decrease in MYC protein (75 %), but also its mRNA level (70 %), suggesting that let-7-mediated repression of MYC may act through the mRNA degradation pathway and not the translational inhibition pathway.
HMGA2 encodes a small, nonhistone chromatin-associated protein that plays a critical role in growth during embryonic development by altering chromatin architecture [57]. It is normally expressed at low levels in adult, but disruption of the gene by chromosomal translocation at 12q15 results in benign and malignant transformation in various tissues [58]. Several groups reported that this chromosomal translocation event removes the 3′UTR of HMG2A and results in the deletion of miRNA-binding sites [59–61]. Mayr et al. [59] and Lee et al. [60] showed that the HMG2A 3′UTR contains seven predicted conserved let-7 binding sites, and a luciferase reporter assay using this 3′UTR confers repression by let-7. The two groups also demonstrated that overexpression of let-7 inhibited proliferation in H1299 lung cancer cells and disruption of the pairing between let-7 and HMGA2 led to enhanced tumor formation in a mouse xenograft model [59, 60].
These findings support the role of let-7 as a tumor suppressor. It has been hypothesized that similar to its role in C. elegans, let-7 functions during late stage embryonic development to maintain differentiated states by suppressing expression of embryonic genes (such as HMGA2) that are expressed in de-differentiated tissues [61]. When let-7 repression is lost, embryonic genes are upregulated and trigger neoplastic transformation with characteristic of embryonic cells. Taken together, these studies suggest that let-7 replacement may be a potentially useful cancer treatment, especially in lung cancer.
17.2.4 Mir-34 and the p53 Network
The mir-34 family is an evolutionary conserved miRNA family first identified in C. elegans. It is located at a fragile site region at 1p36 and has been found to be frequently deleted in various cancers including breast and lung cancer [62]. Recently, numerous independent reports showed that miR-34 is a part of the p53 network and is a direct transcriptional target of p53 [63–67]. miR-34 is directly induced by p53 in response to DNA damage and oncogenic stress. miR-34 by itself has been shown to mimic p53 action to a certain extent, as evident by its anti-proliferation and apoptosis-inducing properties when overexpressed in primary fibroblast and some tumor cell lines [63, 65]. Microarray analysis showed that the induction of the miR-34 family led to the downregulation of hundreds of mRNAs. Some validated targets of miR-34 include CDK4, CDK6, cyclin E2, and E2F3. This was the first time that a miRNA was found to be an integral part of an established oncogene and tumor suppressor network, and suggests that there are other miRNAs belonging to these networks as well. These findings could partially explain why alterations affecting miRNAs are so prevalent in cancers.
17.3 miRNA Profiling: Diagnostics, Classification, Prognosis, and Treatment
miRNA expression profiling stu dies using microarray and other methods can differentiate normal from cancer tissues via a unique miRNA signature. These differences can classify different cancer types and cancer grades. Certain miRNA signatures are correlated with prognosis and could potentially be used someday to determine the specific course of treatment [44, 54, 55, 68–70].
Using a novel bead-based miRNA microarray assay, Lu et al. examined the expression profile of 217 miRNAs in a panel of 334 samples that included primary tumors, tumor-derived cell lines, and normal tissues [68]. The investigators found that miRNA profile can discern between normal and cancer tissues, separate different cancer types, stratify cancer differentiation state, and cluster sample groups according to their embryonic lineage. Moreover, miRNA profiles were more accurate than mRNA profiles in classifying cancer types and were able to categorize 17 poorly differentiated tumors of cancers of unknown primaries where histological appearance was not diagnostic, into their specific tissue lineages.
Studying colorectal cancer, Michael et al. were the first to recognize aberrant miRNA expression in solid tumors as the investigators identified 28 different miRNAs in colonic adenocarcinoma and normal mucosa, and found that miR-143 and miR145 were consistently downregulated in colon cancer [71].
Analyzing the miRNA expression in 104 pairs of primary lung cancers and corresponding noncancerous tissues, Yanaihara et al. found that a set of 43 unique miRNAs were able to discriminate normal from tumor tissues [55]. Among the miRNAs from the signature, high miR-155 and low let-7a-2 expression independently correlated with poor survival for adenocarcinoma patients. In a comparable study, Takamizawa et al. [54] found that let-7 miRNA expression can classify 143 postoperative lung cancer patients into two prognosticator groups. Those with low levels of let-7a had significantly shorter survival after surgery [54].
In breast cancer, the first miRNA profiling study showed that a set of 15 miRNAs correctly predicted normal versus cancer tissues with 100 % accuracy, with the most significantly deregulated miRNAs being miR-125a, miR-125b, miR-145, miR-21, and miR-155 [72]. miR-21 and miR-155 were upregulated and the others were downregulated. miR-125a and miR-125b regulate the expression of the receptor tyrosine kinases ERBB2 and ERBB3 and their overexpression in SK-BR3 cells induced impaired anchorage-dependent growth and reduced invasion capacities [73]. Furthermore, miRNA expression was correlated with specific breast cancer pathological features, such as estrogen and progesterone receptor expression, tumor stage, and vascular invasion.
Papillary thyroid carcinoma (PTC) is the most common malignancy in thyroid tissue. Profiling of PTC by He et al. yielded a signature of five miRNAs (which included miR-221, miR222, and miR-146) that could separate PTC and normal thyroid tissue [44]. The investigators found that upregulation of miR-221, miR-222, and miR-146 corresponded to a dramatic decrease in KIT mRNA and protein levels. This suggests that these three miRNAs negatively regulate KIT and may contribute to PTC. Interestingly, in a separate study, Visone et al. [70] showed that another type of thyroid cancer, thyroid anaplastic carcinomas (ATC), has a completely different miRNA signature from PTC that clearly differentiates ATC from normal tissues and from PTC.
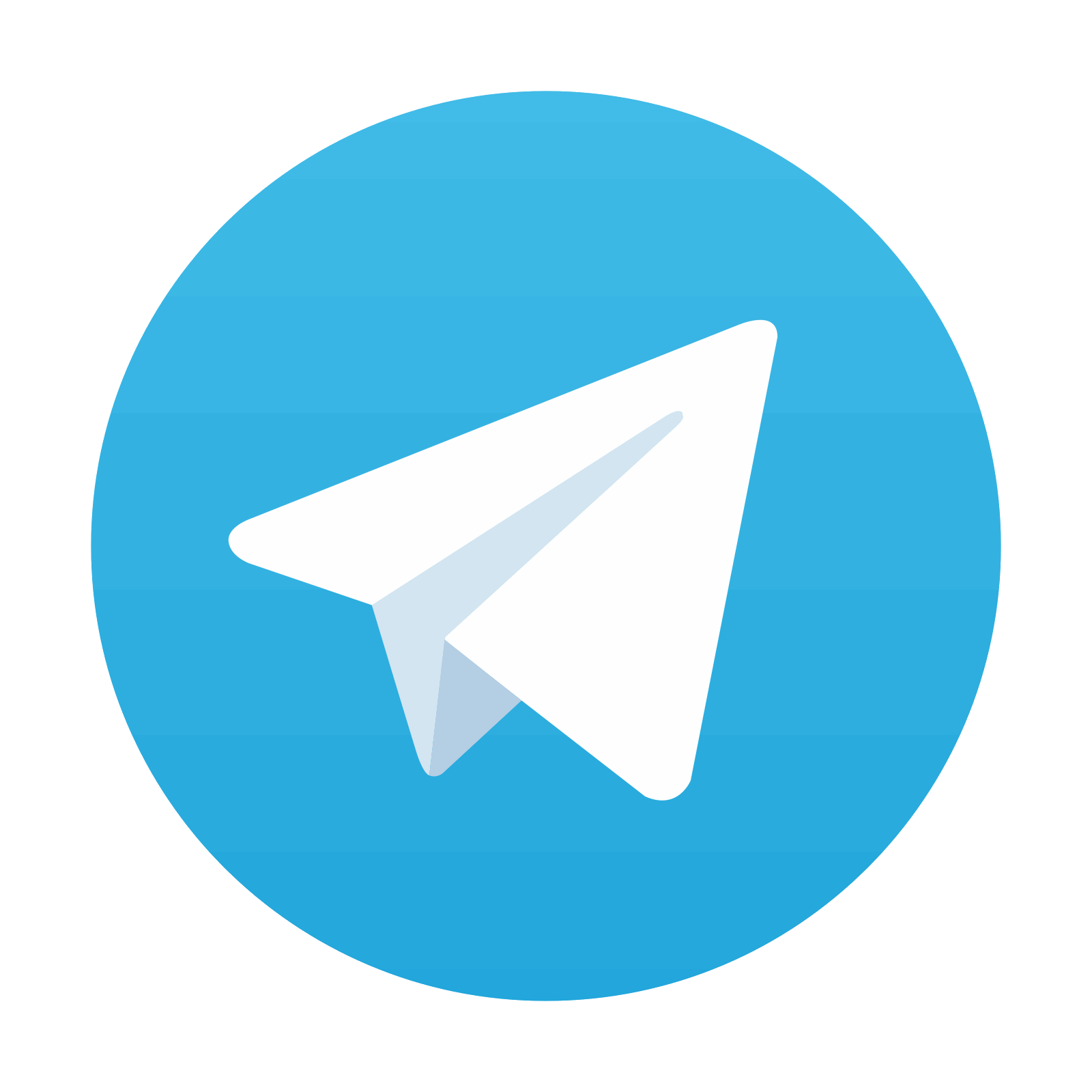
Stay updated, free articles. Join our Telegram channel

Full access? Get Clinical Tree
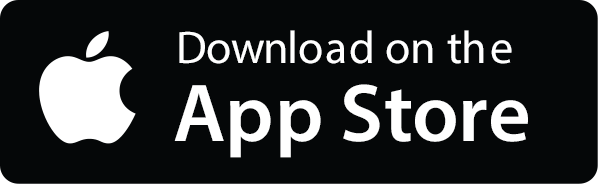
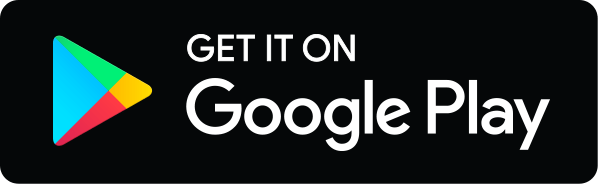