Methods of Immobilization and Stabilization
OVERVIEW OF EXTERNAL BEAM IMMOBILIZATION AND STABILIZATION
Rationale for Patient Immobilization and Stabilization
Accurate delivery of the prescribed treatment is critical to achieve a successful outcome in radiotherapy. There are many sources of uncertainty in the radiation therapy delivery process that can result in a geometric miss of the intended target volume. A geometric miss of the intended target volume will not only decrease the probability of tumor control, but will also increase the volume of normal tissue that is irradiated, which will increase the probability of a treatment-related complication. Geometric uncertainties may be broadly classified as mechanical inaccuracies, localization inaccuracies, and positioning inaccuracies. Mechanical inaccuracies include the coincidence of the light and radiation fields, mechanical stability of the couch, laser alignment, and correspondence of the simulation and treatment isocenters. Localization error relates to the difficult nature of defining the location and extent of the target volume during both planning and treatment delivery. Positioning uncertainties relates to errors in reproducing the patient’s positioning from simulation to treatment delivery, the most undesirable result of which is the target volume moving out of and critical normal tissues moving into the treatment field.
The goal of daily patient setup is to reproduce the position at the time of simulation as best as possible; however, interfractional setup variations from day to day will inevitably occur. Intrafractional positioning uncertainty can also arise from motion of the patient during treatment. Patients may fidget from being uncomfortable or anxious during the treatment delivery. Furthermore, organs can move internally with respect to bony anatomy, skin marks, and other soft tissues due to normal and unavoidable physiologic processes such as respiration, swallowing, and peristalsis. An early study by Haus and Marks1 reported that approximately 30% of the localization errors they observed in their clinic were caused by patient motion. Dunscombe et al.2 also concluded that observed field placement errors were largely due to patient motion.
The focus of this chapter is to describe devices and methods for patient immobilization and stabilization in radiotherapy. The goal of immobilization and stabilization techniques is to reduce positioning uncertainties from patient motion during each fraction (intrafraction error) and to also increase the reproducibility of the patient setup for each fraction (interfraction error). Immobilization devices can decrease the time needed for daily setup and target localization, thus increasing a clinic’s throughput. Certain immobilization devices may also allow setup marks to be made directly on the device instead of the patient’s skin, which can improve the patient’s psychological well-being while under treatment. The immobilization system should be lightweight for ease of setup and transport, yet strong and durable so that the device does not break during the patient’s course of treatment. Furthermore, the device should be made of materials that minimally affect the megavoltage treatment beam and do not cause computed tomography (CT) imaging artifacts that could impact three-dimensional (3D) visualization of the patient’s anatomy.
The devices and techniques for patient immobilization and stabilization described in this chapter have been shown to improve interfraction setup reproducibility, as well as reduce intrafraction uncertainties. The overall accuracy of any immobilization system, however, is dependent on the skill and patience of the therapists forming the device at the time of simulation and setting the patient up on the treatment table for each fraction. Adequate training should be provided for each immobilization system employed in a given clinic, and adequate time should be scheduled for the therapist to properly set up and immobilize the patient. Furthermore, some patients will be more challenging to reproducibly align than others. A variety of devices and positions should be available for each treatment indication to help create a treatment position that maximizes both interfraction and intrafraction reproducibility for each patient. Finally, it must be noted that in some cases, even the most robust techniques for patient immobilization do not guarantee accurate daily localization of the tumor volume and should not be a substitute for image guidance when indicated. Tumors are well known to change position relative to other soft tissue anatomy, external marks, and bony anatomy. Thus, strategies for daily target localization, described elsewhere in this textbook, are also important and are intimately tied to patient immobilization.
FIGURE 21.1. Simple devices are cost-effective and can reduce intrafraction positioning errors by increasing patient comfort. Shown here are rolls and wedges that can be placed under the knees (A) and wedges to support the arms and shoulders (B). A simple strap (C) can be used to suppress the shoulders in head and neck patients, and grip rings (D) can be used for the patient to hold on to. Headrests (E) are available in a wide range of shapes and sizes.
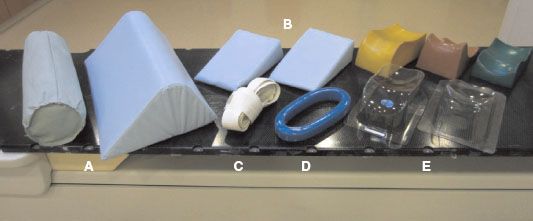
Simple Immobilization Devices
Simple methods to reduce intrafraction positioning uncertainty due to patient motion have been in use for decades to ensure that the patient is comfortable. Figure 21.1 illustrates a variety of simple devices available that can be used to enhance the patient’s comfort. For patients treated in a supine position, a wedge or roll underneath the knees can help to reduce stress on the lower back. For arms-down positioning, a ring for the patient to grip can increase comfort. For lung and liver treatments, it may be preferable to position the patient with the arms above the head. In these cases, the arms may be supported under the shoulder by foam wedges to help the patient attain a restful position. For some head and neck patients, the patient’s shoulders may block the inferior portion of lateral treatment fields. In this case, a simple strap can be used to pull the shoulders down and out of the treatment fields. A headrest is almost always used with supine positioning to elevate the head and reduce strain on the neck. Standard sets of Timo and Silverman headrests come in a variety of shapes, sizes, and materials. For those not adequately accommodated by the standard set of headrests, several manufacturers offer customizable head cushions that can be molded to the patient’s head contour. All of these devices are relatively inexpensive and reusable, and they can be covered and/or cleaned between uses.
Immobilization Features of a Dedicated Radiation Oncology CT Simulator
Simple devices incorporated during initial simulation can improve patient comfort and reduce intrafraction motion due to patient movement. However, they may not address day-to-day variations of overall patient setup and positioning accuracy. A major objective of a robust immobilization system is to reproduce the patient’s anatomic geometry at the time of simulation for all subsequent treatment fractions. An example of a robust immobilization system to treat a patient with breast cancer is shown in Figure 21.2 to illustrate some of the desired features of a dedicated radiation oncology CT simulator. With this immobilization system, the patient lies supine with an ergonomic wedge under the knees. The arms are positioned above the head and out of the tangential treatment fields. A custom-formed, reusable vacuum-lock bag, described in more detail in the following body conformal section, helps to support the patient’s arms, and a T-bar may be used for the patient to grip. The angled baseboard on which the vacuum-lock bag rests uses gravity to help the breasts fall into a more reproducible position and can be adjusted to minimize skin folds. This example of a breast setup, where one or both arms may be positioned above the head, highlights the advantage of a wide bore (e.g., >85-cm diameter) CT scanner for radiation oncology treatment simulation, as opposed to the more common 70-cm bore diameter, which may limit the options for positioning certain patients.
Flat couches are favored for radiation oncology treatments to accommodate a wide range of patient sizes, setup positions, and immobilization devices. Diagnostic CT scanners feature a curved couch for patient comfort; the geometry of a patient simulated on a curved diagnostic couch top will be challenging to reproduce on a flat treatment couch. Flat couch-top inserts are offered by numerous vendors to reproduce the couch geometry of the treatment unit. An important feature of a couch-top insert is the indexing system, which allows an assortment of immobilization devices to be rigidly affixed in concert to both the simulator and treatment tables with high accuracy. The indexing system improves the interfraction reproducibility of the patient setup and can also decrease the amount of time to set up a patient.
External marks placed either on the patient’s skin or the immobilization devices are used for initial laser alignment on the treatment machine as a surrogate for the internal target anatomy. An in-room laser system in the CT simulator suite is a necessary feature to provide external references points that correlate to the internal reference point location. After the simulation CT scan is acquired, the isocenter is defined and the coordinates are transferred to the in-room laser system. The laser system and CT table are then moved to the defined setup point, and external setup marks can be made, thus correlating the position of an internal setup point to the external markings.
FIGURE 21.2. Immobilization system for a breast cancer patient in the simulator suite at the University of Virginia. Some key features of a dedicated radiation oncology CT simulator are an in-room laser system to mark the reference point position (A), a wide patient bore (85-cm diameter) to allow more flexibility in patient positioning (B), and an indexed flat couch-top overlay (C) to reproduce the geometry of the treatment table. An angled breast board (D) can improve patient comfort and provide optimal positioning of the breast for radiotherapy. An indexing bar is used to attach the breast board to the flat couch-top overlay. A vacuum lock mattress (D) and a T-bar (B) support the patient’s arms above the head. An ergonomic knee support (E) can reduce stress on the lower back.
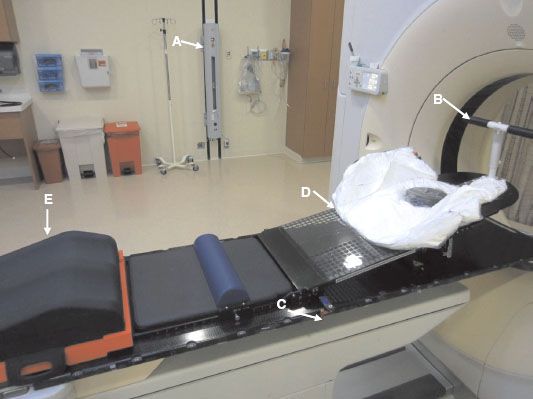
Head and Neck Immobilization Devices
Adequate immobilization is particularly critical in the treatment of head and neck (H&N) cancers, as the target is frequently located in close proximity to critical structures. Thermoplastic masks have replaced traditional plaster-casting methods in most clinics. Thermoplastic masks are heated to approximately 70°C in a water bath. At this temperature, the thermoplastic material becomes malleable and can be stretched and shaped to conform to the patient’s face, head, and neck. The patient’s head and neck is supported by a simple cushion like those previously described and illustrated in Figure 21.1. The mask is connected to a base plate, which is attached to the couch via the indexing system. Setup marks can be made directly onto the thermoplastic mask, eliminating the need for unsightly marks to be placed on the patient’s face for the duration of the treatment.
Early work with thermoplastic mask immobilization for H&N cancer by Bentel et al.3 showcased the efficacy of the thermoplastic mask in comparison to the traditional plaster three-strip immobilization technique. In terms of the rate of physician-requested shifts from port films, the thermoplastic mask had a frequency of 6.2%, compared to 16.1% for the plaster-casting method. However, this advantage was only observed when the mask was rigidly affixed to the couch, highlighting the importance of the couch indexing system.3 Since then, numerous studies quantifying the accuracy of patient setup for H&N cancer have appeared in the literature. The bulk of these studies used two-dimensional portal imaging to quantify setup displacements, although studies using 3D cone-beam CT data are appearing more frequently as this technology becomes adopted by more clinics. Generally, systematic and random errors have been reported to have standard deviations ranging from 1 to 4 mm.4–8 Based on a review of studies assessing setup errors using portal imaging, Hurkmans et al.6 suggested that random and systematic errors of 2 mm or less should be a practically achievable goal for H&N patients, given current technology and methods.
Figure 21.3 illustrates the flexibility of patient positioning options with thermoplastic masks. Masks can encompass the head only or can extend inferiorly to cover the shoulders (Fig. 21.3A). Gilbeau et al.5 compared the setup accuracy of the short and long thermoplastic masks in the treatment of head and neck cancer. Portal images were acquired for isocenters placed at the level of the head, neck, and shoulders. Their results show similar setup accuracy with the long and the short mask for the isocenters in the head and neck. For isocenter placement at the shoulder level, the use of the long mask resulted in a random setup error of approximately 1.0 mm, which was significantly less than the 2.3-mm error of the short mask (p = 0.01).5 Thus, a long mask to immobilize the shoulders is recommended for patients receiving supraclavicular treatment in which the isocenter will be placed at the level of the shoulders. Thermoplastic systems can also accommodate prone or lateral setups when these may be indicated (Fig. 21.3B). Some commercially available base plates can be angled to allow for the patient to be set up with the neck in an extended or flexed position, which may be used to avoid critical structures such as the eyes. Neck extension can be used with prone positioning for craniospinal irradiation. For prone setup, a donut-shaped cushion supports the patient’s face in the base plate, and the thermoplastic mask is formed around the posterior aspect of the head to hold the patient’s face in the donut. A potential disadvantage of treatment with a flexed or extended neck position is the need for registration with different imaging modalities (e.g., positron emission tomography [PET]-CT or magnetic resonance [MR]-CT) in which the PET or MR were not performed in the patient treatment position.
Every clinic must consider space limitations when selecting particular immobilization and stabilization systems. Thermoplastic H&N systems necessitate more equipment and thus more space in the simulation suite. The water bath to heat the thermoplastic sheets for the long H&N masks can be large (Fig. 21.3C). For clinics with insufficient space to house a large water bath, smaller water baths are commercially available that will fit the short head-only masks. Furthermore, some commercially available base plates (e.g., VersaBoard, Bionix Radiation Therapy, Toledo, OH) incorporate shoulder suppression systems, which consist of rigid plastic panels to exert downward pressure on the shoulders. When a long thermoplastic mask is not indicated or not available, a base plate with shoulder suppression panels can help to keep the shoulders out of the beam path for lateral head and neck beams (Fig. 21.3A). In lieu of long thermoplastic masks or base plates with shoulder suppression panels, the patient can pull on a simple shoulder strap (see Fig. 21.1) to move the shoulders inferior and avoid unnecessary irradiation of the shoulders when opposed lateral beams are used to treat the neck.
FIGURE 21.3. For treatment of targets in the head and neck, thermoplastic masks are routinely used for custom immobilization. A: Thermoplastic sheets are commercially available in short versions that cover only the head, as well as in longer versions that extend over the patient’s shoulders. B: They can also be used to treat patients in the prone and lateral positions. C: Additional space is needed to accommodate the water bath used to warm the thermoplastic sheets. (A and B courtesy of Bionix Radiation Therapy, Toledo, OH.)
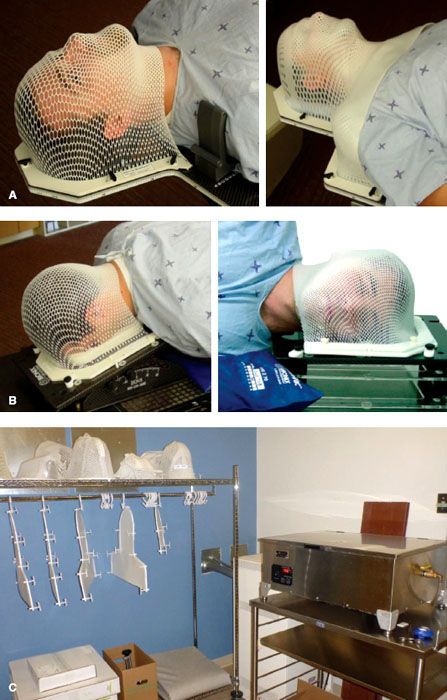
FIGURE 21.4. A: The Elekta HeadFIX is an example of a bite-block system that can provide an alternative method for immobilizing head and neck patients who are not able to tolerate a thermoplastic mask due to anxiety or claustrophobia. B: A custom dental mold is formed for each patient and is suctioned to the patient’s hard palate. The dental mold is attached to a carbon fiber frame featuring a quick-release handle. (Courtesy of Elekta, Norcross, GA.)
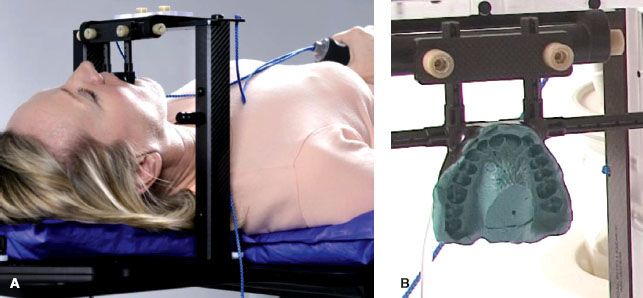
Thermoplastic masks have been observed to shrink as they cool in the 24-hour period after formation, and this has been shown to lead to systematic setup errors if not taken into consideration.9 Furthermore, a mask that has shrunk after the simulation process may be uncomfortable for the patient and may require resimulation and another treatment plan to be developed. Bionix Radiation Therapy attempted to address this problem with the Klarity Green line of thermoplastic material, which is designed to have reduced shrinkage coupled with increased rigidity. As with any immobilization system, the thermoplastic manufacturer’s recommendations should be carefully followed, and adequate time should be allowed during simulation for the mask to harden properly to minimize the effect of shrinkage.
Thermoplastic masks are widely used for H&N treatment indications; however, they are not well tolerated by all patients, particularly patients with claustrophobia. For these patients, bite block systems that do not incorporate a mask may be an acceptable alternative. Elekta (Norcross, GA) offers a bite-block system, the HeadFIX, which is illustrated in Figure 21.4. A custom dental mold is formed to the patient’s maxillary teeth, and a vacuum system creates suction of the mold to the hard palate. A custom cushion is placed under the patient’s head, neck, and shoulders. The mouthpiece is attached to a carbon fiber frame, which is fixed to the treatment table’s indexing system. The head is thus rigidly immobilized via the connection of the mouthpiece to the head frame. The carbon fiber design of the HeadFIX product allows for the use of in-room kilovoltage (kV) image guidance systems for target localization and the validation of setup accuracy. The mold can be quickly released from the frame if the patient feels discomfort. Setup accuracy has been shown to be similar to that of thermoplastic masks, on the order of 1 to 3 mm.10,11 These stand-alone bite-block systems, however, require a high level of patient compliance and may not be suitable for patients with poor dentition or who are endentulous. Use of bite blocks formed with a patient’s false teeth is highly discouraged and may result in inaccurate setups or breakage of the dentures. Custom-formed dental molds can also be incorporated in the conventional thermoplastic mask systems described earlier to provide an additional point of support for the patient. A custom bite block may also be formed with primary goals other than immobilization—for example, to separate the tongue from the roof of the mouth. This can be useful in treatment of sinus/nasal cavity tumors to decrease dose to the tongue and vice versa.
Body Conformal Immobilization Devices
Numerous commercial body conformal immobilization devices are available for a variety of treatment indications. One type of body conformal system uses a two-part foaming agent to form a permanent mold of the patient’s body. One such system, Alpha Cradle (Smithers Medical Products, North Canton, OH), is illustrated in Figure 21.5A,B. Forms are available for treatment of patients with a variety of disease sites, including the head and neck, breast, thorax, abdomen, pelvis, and extremities. At the time of simulation the appropriate body form is chosen and is placed in a polyvinyl bag. The two-part chemical foaming agents are mixed together, initiating the chemical reaction that causes the foam to expand. The mixed foaming agent is then distributed evenly throughout the body form and sealed inside the polyvinyl bag. The patient is positioned inside the form as the foaming agent expands and conforms to the patient’s body contour. The foam will continue to expand for approximately 10 to 15 minutes. During the foam expansion, the therapists will need to vigilantly guide the foam around the patient to prevent the foam from escaping the bag and moving into locations of least resistance that may contribute little to immobilization of the patient. As with any immobilization device, adequate training is needed to form a high-quality customized patient mold, and the manufacturer’s instructions should be carefully followed. Chemical foaming agent systems generally require two therapists to form at the time of simulation, and the process can require approximately 30 minutes. Obviously, great care is needed to prevent any of the chemicals from having direct contact on the skin, as this may cause irritation.
Another class of body conformal systems, termed here “vacuum-lock bags,” comprise a bag filled with plastic minispheres. These bags are available in a variety of sizes to accommodate different treatment sites and patient positioning. At the time of simulation, the bag is first conformed to the patient’s body contour by pushing the minispheres to fill in around the patient. A vacuum pump is then connected to the bag, and the air is evacuated from the bag, causing the plastic minispheres to lock together to rigidly retain the device’s shape. During the evacuation, the therapists maneuver the minispheres within the immobilization device to provide optimal fit and stabilization of the patient’s body contour. These vacuum-lock bags are made of a durable nylon material to resist tears or punctures and can be expected to retain their shape for 6 weeks or more. They are reusable and may require less time to generate a customized mold than the expanding foam systems. Another advantage of the vacuum-lock bag is that if during the simulation process the device is found not to provide the intended level of immobilization or is found to be uncomfortable for the patient, it can be reinflated and remolded to achieve the desired shape as many times as is necessary. The vacuum-lock systems, however, do require a time commitment to clean and remove any setup marks prior to use with subsequent patients. In addition, if a tear or puncture occurs during the course of treatment, a new vacuum-lock bag would need to be formed, and the simulation and treatment planning process may need to be repeated to deliver the remaining fractions. Each clinic should consider the benefits and drawbacks of each system. It is not uncommon to see both types of body conformal systems used within one institution.
Because body conformal devices are used to treat a wide range of sites throughout the entire body, careful thought should be given to each specific case to ensure that the treatment site is properly immobilized, the setup is reproducible from day to day, and the patient is able to maintain the position for the duration of each fraction. An immobilization device that extends well beyond the treatment site to encompass adjacent anatomy can be more effective than a more local device that focuses only on the treatment region. For example, Bentel et al.12 showed that for Hodgkin lymphoma, a cradle that extends below the pelvis is more effective than a cradle that only includes the upper torso. Devices that extend around the patient also give the ability to make setup marks directly on the immobilization device for which to align the radiation isocenter. Aligning the beam to setup marks on the immobilization device can increase the daily setup reproducibility13 by reducing the reliance on skin marks, which can be especially troublesome for larger or older patients, in whom the skin can be more mobile overtop the underlying anatomy. Marks can then be made on the patient to help align the patient within the immobilization device. Longer devices also allow for longer setup marks to be made in the superior–inferior direction, which can help to detect small rotational setup errors.
FIGURE 21.5. Body-conformal systems are used for treatment indications throughout the body. A, B: Two patients in treatment position with the Alpha Cradle, which uses a two-part foaming agent to create customized conformal body immobilization. (A and B courtesy of Smithers Medical Products, North Canton, OH; Alpha Cradle is a registered trademark of Smithers Medical Products.) C: In Elekta’s BodyFIX system, a cover sheet [1] is placed over the patient after being positioned inside of the vacuum-lock bag [2] that was formed at the time of simulation. A separate vacuum system [3] evacuates the air between the cover sheet and the patient. The pressure from the cover sheet can help to reduce patient motion and improve setup reproducibility. (C courtesy of Elekta, Norcross, GA.) D: Room used for storing vacuum-lock bags in the simulation suite at the University of Virginia. The simulation suite services multiple treatment machines at satellite locations. After simulation, the immobilization devices are transported to the satellite treatment sites in body bags (pictured on the right) via a courier service.
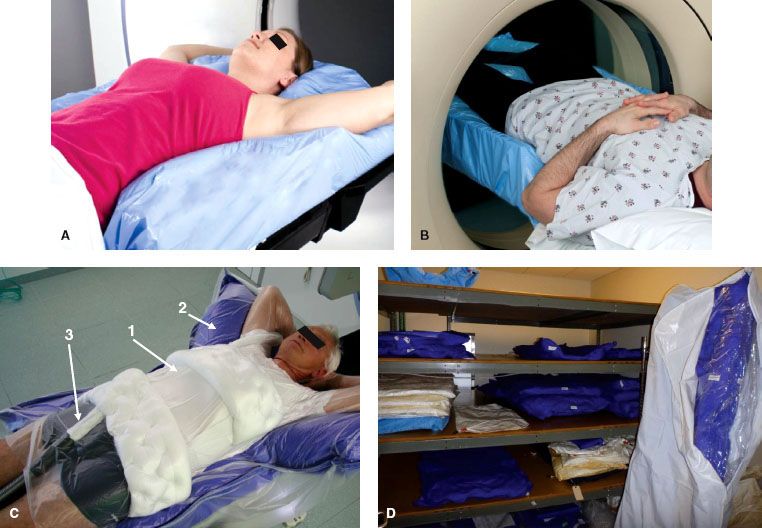
Early work by Bentel and colleagues at Duke University showed that immobilization with the Alpha Cradle expanding-foam system reduced the frequency of physician-requested setup corrections based on portal images for a variety of treatment indications, including Hodgkin lymphoma12 and lung,14 breast,15 and prostate cancer.13 It should be noted, however, that not all studies show a clear-cut advantage in setup accuracy with body conformal immobilization devices. For example, an early study by Song et al.16 showed no statistical advantage of using immobilization in the treatment of prostate cancer in terms of the number of deviations greater than 5 mm as quantified on portal images. They did show, however, that obese patients tend to have larger setup errors,16 highlighting the fact that certain subpopulations of patients may be more difficult to set up than others. The review of setup accuracy by Hurkmans et al.,6 as assessed with portal imaging, provides a good review of the data up to 2001. This work concluded that with current patient setup, portal imaging, and immobilization techniques, systematic and random setup errors on the order of 2.5 to 3.5 mm should be achievable for treatments of the thorax, abdomen, and pelvis. The integration of cone-beam CT units into treatment machines is an excellent tool for assessing setup accuracy and is being used in an increasing number of studies comparing and evaluating various immobilization systems. This technology has been especially leveraged to study the setup accuracy of stereotactic immobilization devices, the subject of the next section in this chapter. It should be emphasized that the degree of interfraction setup accuracy achievable by a particular institution will depend on the skill and knowledge of the personnel involved in forming patient immobilization devices, setting up patients for daily treatments, making adjustments based on verification imaging, and the understanding of the limitations of each step of the process.
The Elekta BodyFIX is a unique immobilization system that uses a dual-vacuum system as shown in Figure 21.5C. For simulation, the patient is first placed in a vacuum-lock bag system. A thin plastic cover sheet is then placed over the patient, which is attached to the vacuum-lock bag via special adhesive strips. A vacuum pump is then used to evacuate the air under the cover sheet, creating a continuous pressure of up to 600 mbar. The additional pressure created by the cover-sheet vacuum system helps the patient settle into the vacuum-lock bag, which is molded to the patient and then evacuated with a second vacuum system to create the body-conformal device. For all subsequent treatment fractions, the pressure from the cover-sheet vacuum system ensures that the patient is reproducibly located in the vacuum-lock bag. The BodyFIX can provide a degree of abdominal compression on the vacuum sheet that is placed over the abdomen, which may help to decrease the respiratory excursion of certain tumors. Internal target motion management is discussed further in the section of this chapter on stereotactic immobilization. Patients in our clinic tolerate the BodyFIX dual-vacuum system very well, with infrequent complaints of claustrophobia or discomfort. If the patient feels that the pressure from the cover sheet is uncomfortable, the vacuum for the cover sheet can be turned down, or the vacuum-lock bag can be used by itself for certain indications.
Whole-body expanding-foam or vacuum-lock bag systems can provide excellent patient comfort and immobilization, but they are large devices, making the issue of space very relevant. Adequate space is required to store each patient’s custom-formed device for up to 6 weeks or more while a patient is under treatment. At the Emily Couric Clinical Cancer Center at the University of Virginia (Charlottesville, VA), a dedicated room next to the simulation suite and large closets in each treatment room are used for storing patient body conformal immobilization devices (Fig. 21.5C). For clinics where one simulation suite is used to support multiple satellite clinics, such as at the University of Virginia, the logistics of transporting the immobilization device must also be considered. At the University of Virginia, a courier service is used to transport the large body-conformal devices in body bags from the simulation site to satellite treatment facilities. Each clinic must assess space availability when deciding which system to adopt in patient care.
FIGURE 21.6. Immobilization devices specifically designed for prone positioning. A: A prone belly board can be used in treating targets in the pelvis. Gravity assists small bowel migration out of the treatment fields, which can reduce treatment-related toxicity. B, C: A prone breast board offered by Bionix Radiation Therapy can be advantageous for patients with large, pendulous breasts. The board features a cutout that is easily reversible for treatment of either breast. (Courtesy of Bionix Radiation Therapy, Toledo, OH.)
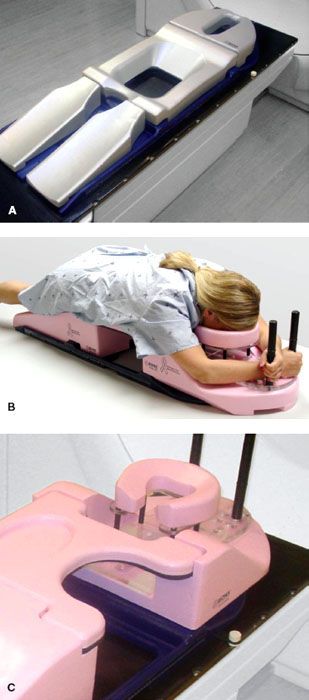
PRONE POSITIONING AND IMMOBILIZATION
The body-conformal devices just described can help to provide reproducible patient setup from day to day for a wide range of disease sites. The patient is most often positioned supine in these devices. For certain treatment indications, a prone setup may provide a dosimetric advantage. In pelvic and abdominal irradiation, the small bowel is an important organ at risk, and acute toxicity is correlated with the amount of small bowel that receives a high dose. For patients receiving pelvic irradiation, a popular method is to use a belly board (Fig. 21.6A). The patient lies prone on the board with the stomach positioned over the cutout region. With the aid of gravity, the small bowel will fall into the board’s cutout and can reduce the amount of bowel in the treatment fields. The use of a prone belly board has been demonstrated to reduce the volume of small bowel at all dose levels.17–20 Even with intensity-modulated radiation therapy, the use of a belly-board technique has been shown to further reduce the small bowel dose.18
The magnitude of the small-bowel reduction with prone belly board positioning can be highly patient-dependent. Kim et al.18 correlated the amount of small bowel at the 50% isodose level with a patient’s age, weight, and gender. For example, this work showed a larger amount of small bowel (total volume in cubic centimeters) at the 50% isodose level for the subgroups of patients greater than 56 years old, female patients, and patients weighing less than 65 kg (143 lb). In contrast, Das et al. did not find any correlation with patient weight, age, gender, or whether radiation was delivered presurgery or postsurgery. It must also be noted that some investigators reported that the use of a belly board worsened the reproducibility of the patient setup.17 For example, Martin et al.19 reported having to reposition the patient based on portal imaging 68% of the time with a prone belly-board system, compared to 23% of the time with supine positioning. In contrast, Olofsen-Acht et al.20 reported similar setup reproducibility between prone positioning with a belly board and patients in the supine position. Each patient should be assessed at the time of simulation for the efficacy of the belly-board method to displace small bowel, as well as the patient’s ability to tolerate lying on the belly board itself.
Another site where prone positioning has been used is in the treatment of breast cancer patients. Figure 21.6B shows a patient positioned on a prone breast board offered by Bionix Radiation Therapy. The patient is positioned on the cushioned platform with her face resting in a donut-shaped cushion. The patient can hold the hand posts to help improve reproducibility and comfort. The prone positioning is most efficacious for patients with large, pendulous breasts, although it may also be an effective option for patients with severe arthritis that may be unable to hold their arms over their head.21 Patients with large breasts tend to have larger tissue separation, larger treatment volumes, worse surface irregularity, and an accentuated inframammary fold. These characteristics can cause patients with large breasts to have higher reported rates of both acute and late toxicities such as high-grade dermatitis and fibrosis.22,23
The prone position has been shown to improve the dose homogeneity throughout the breast volume since the lateral tissue separation is minimized in the pendant geometry.24,25 This improved dose homogeneity may decrease the rate of late toxicities such as fibrosis, which can lead to undesirable cosmetic consequences. Decreased tissue separation can decrease the entrance dose needed to provide target coverage and may decrease the overall hot spot for the treatment plan, particularly at the skin surface.21 Improved dose homogeneity and decreased skin dose from prone positioning may also prove to be advantageous for hypofractionated breast regimens, where larger doses per fraction are delivered. Five-year follow-up data from Stegman et al.21 showed that the prone position offers comparable local control to traditional supine positioning for whole-breast irradiation, with lower levels of toxicity. With the breast hanging in the prone setup, it may also be possible to reduce the dose to nearby normal tissues. The increased separation of the breast tissue from the chest wall has been shown to decrease the amount of irradiated lung in whole-breast irradiation.26 Dose to the heart is more variable; for left-breast patients, most heart doses (20 to 30 Gy) are decreased in the prone position, although some patients did exhibit higher heart doses when prone.26
Large-breasted patients can also be very troublesome to reproducibly set up with supine positioning because the breast can lie in different positions from day to day. In the supine position, a ring or tube can be used to keep the pendulous breast from folding over. With the aid of gravity, daily setup can be more reproducible with the breast hanging in the prone position. There are, however, potential disadvantages to the prone setup for breast patients. The therapists have reduced visibility of the breast with which to align the treatment fields on a daily basis. Furthermore, the prone positioning may not be tolerable for elderly or obese patients. Finally, the prone position may not be logistically suitable for patients requiring regional nodal irradiation because the prone position would severely limit visual alignment of an anterior supraclavicular field.
Total Body Irradiation Immobilization
Several devices are available for clinical programs that include total body irradiation (TBI) with photons, generally in preparation for bone marrow transplantation. These devices can also be designed to rotate and also provide total skin irradiation for treatment of mycosis fungoides. Features of these systems generally include a beam spoiler, because a maximum dose at the skin entrance is preferred for TBI; strategies for applying partial lung blocks; and methods to keep the patient stable while standing for the treatments, which may take from 10 to 40 minutes, depending upon the treatment protocol. The major disadvantage for these systems is identifying storage in the treatment room; they are large and often take up vital space in the treatment area. It is important to note that these systems usually operate at a distance of about 5 m from the target, with the gantry at a horizontal angle (90 or 270 degrees). Figure 21.7 shows two representative TBI devices from Radiation Products Design (Albertville, MN) and Mick Radio-Nuclear Instruments (Mount Vernon, NY). Features such as a bicycle seat, handgrips, and shoulder stabilizers are used to help support the patient. Some TBI stands offer a harness that will provide an additional measure of security to prevent the patient from falling over in case the patient succumbs to fatigue and faints.
Dosimetric Effects of Immobilization Devices
Any material placed between the patient and the radiation source can modify characteristics of the treatment beam. Modern immobilization devices made of low-density materials have little impact on the depth–dose characteristics of megavoltage energy treatment beams. Electrons liberated within the immobilization device can, however, lead to a measurable increase in the patient’s surface dose, that is, a bolusing effect. Clinical experience supports this effect and has shown that skin reactions are more frequent, and tend to occur earlier in the treatment course, when beams are delivered through an immobilization device.27,28 The magnitude of the bolus effect depends on the composition, density, and thickness of the immobilization device.
For thermoplastic head and neck masks, the bolus effect is reduced when the masks are stretched more as the thickness of material in the beam’s path is subsequently reduced. The clinician may also cut out parts of the mask to remove material in the beam path to reduce skin reactions. Care must be taken, however, to ensure that the integrity of the mask and its ability to provide rigid immobilization are not compromised. With proper skill and attention, thermoplastic masks with cutouts to improve skin sparing have been shown to achieve similar setup accuracy as unmodified masks.7,29 For custom-made foam body-conformal systems, such as the Alpha Cradle, foam lying in the beam path can be shaved away to reduce the thickness along the beam path. Reusable vacuum-lock bags are commercially available with treatment beam portals for standard beam arrangements to avoid bolusing.
FIGURE 21.7. A stand is commonly used to help position patients for total body irradiation (TBI) with photons and electrons. A: Common features of TBI stands include hand grips and a seat to help support the patient. B: Harness used to prevent the patient from falling in the case of fatigue and/or fainting. (B courtesy of RPD, Albertville, MN.)
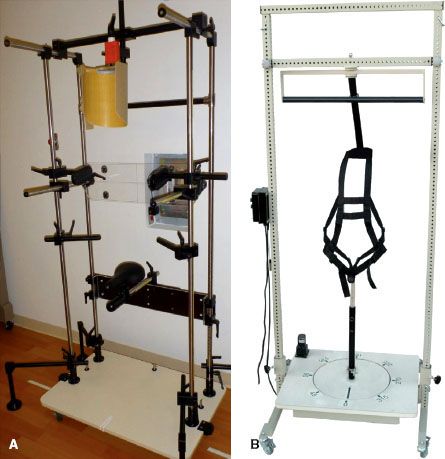
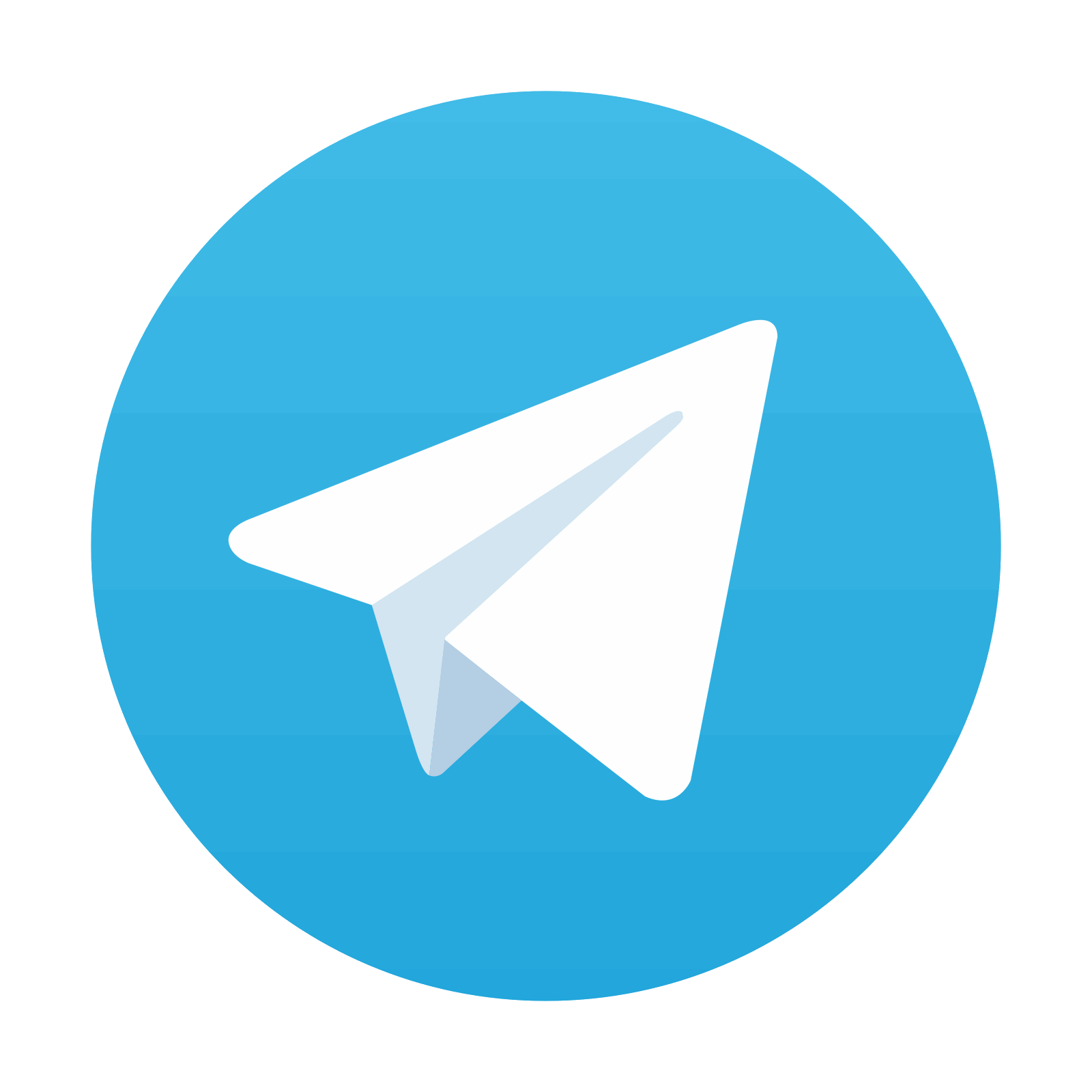