Medical Management of Differentiated Epithelial Cell Thyroid Cancer
Sebastiano Filetti
R. Michael Tuttle
Steven I. Sherman
The medical management of patients with differentiated thyroid cancer (DTC) begins following completion of the primary surgical procedure, and continues for the remainder of the patient’s life. Although the basic concepts of management have not fundamentally changed in decades [radioactive iodine (RAI), thyroid hormone treatment, long-term monitoring for persistent or recurrent disease, and treatment of metastases], the options available and application of these management strategies continue to evolve. Whereas 20 years ago the primary focus was on near-universal application of RAI for both scanning for and treatment of disease, more recent paradigms have been based on more nuanced risk stratification, selective use of RAI as adjuvant therapy, increasingly sensitive detection of disease by serum thyroglobulin (Tg) measurements and neck ultrasound (US), and identification of patients with metastatic disease who might benefit from new systemic treatments and/or palliative interventions. The sequential changes in the treatment guidelines of the American Thyroid Association (ATA) since 1996 summarize well this evolution (1,2,3).
Radioactive Iodine Therapy
RAI is one of the earliest forms of targeted cancer therapy in the history of medicine. For over 50 years, 131I has been used to deliver therapeutic radioactivity to DTC tissue in the thyroid bed and elsewhere in the body. RAI therapy’s selectivity for DTC cells depends on the functional presence of a network of highly specialized proteins—including the sodium iodide (Na+/I–) symporter—that are involved in physiologic iodine metabolism (4). This complex machinery, which allows follicular cells to trap and retain iodine for use in hormonogenesis, remains functional to some extent in primary thyroid cancer cells, depending on their degree of dedifferentiation (5). Varying degrees of impairment occur but complete loss of this function is restricted to undifferentiated and anaplastic primary cancers. Dedifferentiation also explains why some (but not all) metastatic thyroid cancer cells exhibit even less efficient iodine uptake than their primary tumor counterparts, although evidence suggests the iodine-trapping capacity of metastatic thyroid cells might also be site-specific (i.e., lymph nodes, bone, lung, liver) (6). Despite its cornerstone role in the management of DTC, views on the use of RAI therapy have changed, and it is currently being recommended in more limited situations (3). These changes reflect in part our growing understanding of the natural history and biologic behavior of DTCs, and in part our increasing appreciation of the limitations and risks posed by the treatment. Although molecular as well as histologic differences separate papillary, follicular, and Hürthle cell carcinomas, the evidence that RAI treatment approach should differ among them is insufficient to provide separate recommendations.
Radioactive Iodine Remnant Ablation
The rationale for postoperative administration of 131I—commonly referred to as radioiodine remnant ablation (RRA)—is based on two assumptions. First, it can facilitate subsequent follow-up by eliminating residual traces of normal thyroid tissue, which reduce the specificity for detection of recurrent or residual tumor tissue by measurement of serum Tg and functional imaging studies using RAI (7). Second, the procedure is a form of adjuvant therapy, which could improve the long-term outcome by destroying any occult microscopic carcinoma within the thyroid remnant or elsewhere in the body. RRA has always been surrounded by some degree of controversy, and the debate shows no signs of subsiding (8,9,10).
Ablation of Residual Normal Thyroid Tissue
Measurement of basal and stimulated Tg levels has emerged as a cornerstone of postoperative surveillance programs for patients with DTC, as Tg is only produced by cells of thyroid follicular origin (11). In the absence of normal thyroid tissue, detectable Tg levels are a highly specific marker of residual or recurrent disease, although levels may take several years to decline to an undetectable nadir following primary therapy (12,13). But in patients who have not undergone RRA, the clinical significance a persistently detectable Tg is not always clear, as the Tg production may originate in the normal thyroid remnant (14,15). There are currently no reliable cutoffs for distinguishing Tg production stemming from persistent or recurrent cancer from that originating in normal residual thyroid tissue, and this limitation is particularly critical to interpret the results of the initial postoperative serum Tg assay. Thus, eliminating this nonneoplastic tissue should increase the specificity of serum Tg levels during follow-up.
This concept also applies to diagnostic whole-body RAI scans. These functional imaging studies may not detect ectopic tumor tissue when appreciable amounts of normal thyroid tissue remain after surgery. In vivo studies in patients with metastases have shown that 131I uptake in neoplastic tissue is relatively lower (and sometimes undetectable) when normal thyroid tissue is present because iodine metabolism in the
tumor cells is always impaired to some extent (4). One of the aims of RRA is to maximize the visualization of uptake by eliminating the competition for detection by nuclear cameras provided by the normal tissue.
tumor cells is always impaired to some extent (4). One of the aims of RRA is to maximize the visualization of uptake by eliminating the competition for detection by nuclear cameras provided by the normal tissue.
The arguments cited in support of RRA are now being challenged. The role of US in the follow-up of patients with DTCs has increased considerably over the last two decades (16). Aside from the obvious advantages of a method that is relatively inexpensive, widely available, and associated with zero risk in terms of radiation exposure, US offers diagnostic gains that are especially (though not exclusively) evident in low-risk patients, who represent an increasing proportion of the total DTC population. In this group, US has been shown to identify persistent or recurrent neck disease earlier and with higher sensitivity than serum Tg measurements or RAI scanning, independent of initial RRA (17,18). Its negative predictive value can be very high, and when positive US findings are confirmed by US-guided fine-needle aspiration biopsy, its specificity is also higher than that of the previously mentioned methods (15,17). A key caveat, however, is that US is highly operator dependent, and contemporary follow-up paradigms described below require access to high-quality US performance and interpretation (19). RAI scanning has been recognized to have poor sensitivity for detection of recurrent or metastatic disease, and thus the need to enhance its specificity through RRA carries considerably less value (17,20). As for monitoring Tg in the absence of RRA, use of serial measurements can reveal changes over time that may be sufficiently informative. Although this issue has never been specifically investigated in large series of unablated patients, studies of patients who have undergone postoperative RRA suggests that progressive increases in serum Tg values are highly predictive of persistent or recurrent neoplastic disease (13,18).
RAI Treatment as an Adjuvant Therapy
Post-thyroidectomy administration of 131I is also advocated as a means to destroy any microscopic nests of thyroid cancer cells that may have been left behind after surgery, within the residual glandular tissue or elsewhere in the body. In this case, RRA represents a form of adjuvant therapy aimed at reducing the risk of cancer recurrence and improving the long-term outcome by eliminating potential microfoci of malignancy, whose existence and location have not been ascertained.
Several retrospective studies have documented decreases in both disease recurrence (21,22,23) and disease-specific mortality rates (22) following RRA. One of the pioneer studies in this area reported on 1,355 patients with DTC followed for a median of nearly 16 years (23). The 30-year recurrence rate and likelihood of cancer-related death in individuals who had undergone RRA were approximately 3-fold lower than those observed in nonablated patients. However, other studies addressing this issue have failed to demonstrate that RRA confers any clinical benefits in terms on long-term outcomes (8,24).
The main reason for these conflicting results probably lies in the heterogeneous risks for recurrence and for disease-specific mortality in retrospective study cohorts. For example, the benefits suggested following RRA were not seen in patients with smaller primary tumors (i.e., measuring less than 1.5 cm) (21,23). As the proportion of patients regarded as low-risk increases in the study population, the impact of RRA on long-term outcomes is likely to become less evident. This issue has been further explored in more recent studies in which the effectiveness of RRA was assessed as a function of the disease stage at diagnosis (8,9,24,25). In one of the largest multicenter studies, the National Thyroid Cancer Treatment Cooperative Study Group (NTCTCSG) published its experience with 2,936 patients treated for DTC (25). In multivariate analysis of outcomes after a median follow-up of 3 years, independent benefits from RRA were observed only in patients with NTCTCSG stage III or IV tumors. In this subgroup, statistically significant improvements were seen in overall survival (35%), disease-free survival (32%) and disease-specific survival (46%). Stage II patients may also have had improved survival after RRA, although this was not significant independent of surgical extent. However, patients with NTCTCSG stage I tumors, which included nearly 50% of the cohort, showed no suggestion whatsoever of improved clinical outcomes, and further analysis extended this observation to all patients with subcentimeter PTC tumors regardless of nodal disease status (25,26,27). More selective, risk-adapted use of RRA is now inherent in multiple consensus guidelines statements for the management of DTC, including those of the ATA, National Comprehensive Cancer Network (NCCN), and European Thyroid Association (3,28,29).
The implications of this more conservative approach have been explored in a retrospective, Italian multicenter study, which examined long-term outcomes in 175 “very low-risk” cases of DTC (i.e., unifocal, intrathyroidal, subcentimeter PTC without known high-risk features) that were managed without postoperative RRA (15). During follow-ups ranging from 5 to 23 years (median 6.7 years), none of these patients experienced disease recurrence or died from thyroid cancer. These findings were confirmed in a single-institution study conducted at the Memorial Sloan-Kettering Cancer Center (30). Omission of RRA led to very-low rates of imaging-documented disease recurrence in 120 patients whose primary tumors measured 1 to 4 cm, had minimal or no extrathyroidal extension, and minimal or no involvement of cervical lymph nodes. Follow-ups ranging from 0.5 to 34 years (median: 5 years) showed signs of recurrence in only 5 patients (4.1%).
Careful assessment of benefit and risk from RAI is required to identify DTC patients who are likely to gain from RRA and those who can be managed without it (Table 50E.1). Randomized, controlled trials are needed for a more in-depth exploration of the effects of RRA on recurrence and disease-specific mortality, an essential step before we can draw firm conclusions on this issue, such as the European study that has recently been initiated (IoN, NCT01398085).
Table 50E.1 Rationale for radioactive iodine administration in patients with DTC. | ||||||||||||||||||||||||
---|---|---|---|---|---|---|---|---|---|---|---|---|---|---|---|---|---|---|---|---|---|---|---|---|
|
Patient Preparation
Traditionally, patients scheduled for RRA therapy were withdrawn for thyroid hormone treatment for at least 3 weeks, to permit elevation of the serum TSH concentration >30 mU/L for maximum uptake and organification of the 131I and optimal therapeutic effects. Recombinant human TSH (rhTSH) has also been approved for this purpose in the United States and in Europe. It stimulates thyroid 131I uptake and Tg release while the patient continues thyroid hormone therapy, thereby eliminating the unpleasant symptoms of hypothyroidism associated with hormone withdrawal (31). The U.S. Food and Drug Administration and the European Medicines Agency’s decisions to approve this indication for rhTSH were based on a small prospective, randomized trial, which suggested that
thyroid hormone withdrawal and exogenous TSH stimulation are equally effective in preparing patients for RRA with 3,700 MBq (100 mCi) (32,33). All of the patients in both groups achieved complete ablation, defined as less than 0.1% of uptake on a 150 MBq (4 mCi) 131I diagnostic whole-body scans performed 8 months, and there were similar rates of requiring retreatment within the next 3 to 4 years. The frequency of low stimulated Tg levels during follow-up were also similar. As expected, preparation with rhTSH was associated with significantly fewer symptoms than hormone withdrawal. Recent, large randomized trials have confirmed these findings of the ablation efficacy of RAI administered after rhTSH, and a retrospective study suggests that the adjuvant role of RRA may also be accomplished using rhTSH (34,35,36).
thyroid hormone withdrawal and exogenous TSH stimulation are equally effective in preparing patients for RRA with 3,700 MBq (100 mCi) (32,33). All of the patients in both groups achieved complete ablation, defined as less than 0.1% of uptake on a 150 MBq (4 mCi) 131I diagnostic whole-body scans performed 8 months, and there were similar rates of requiring retreatment within the next 3 to 4 years. The frequency of low stimulated Tg levels during follow-up were also similar. As expected, preparation with rhTSH was associated with significantly fewer symptoms than hormone withdrawal. Recent, large randomized trials have confirmed these findings of the ablation efficacy of RAI administered after rhTSH, and a retrospective study suggests that the adjuvant role of RRA may also be accomplished using rhTSH (34,35,36).
Selection of Radioactive Iodine Activity for Remnant Ablation
For many years, RRA was performed with relatively high administered activities (2,775–5,550 MBq [75–150 mCi]) but clinicians have now begun to use lower amounts of 131I. Several small studies demonstrated that rates of successful remnant ablation in patients who receive 1,110 MBq (30 mCi) are similar to those achieved with 3,700 MBq (100 mCi) but perhaps with lower adverse effects. When pooled data from these studies were subjected to a meta-analysis, successful RRA was not significantly more likely after higher activities (relative risk 1.15, 95% confidence interval 0.97 to 1.35) (37). In Europe, authorized use for stimulation of RRA is currently limited to 3,700 MBq (100 mCi) of 131I but lower activities of RAI (such as 1,110 MBq [30 mCi] or 1,850 MBq [50 mCi]) may be equally effective after rhTSH for eliminating remnant (34,35,38,39). Prospective outcomes data are still lacking, however, to assess whether recurrence rate or disease-free survival after rhTSH-stimulated lower administered activities are similar to those after more traditional higher activities.
For low-risk patients, guidelines recommend an activity range of 1,100–3,700 MBq (30–100 mCi) and advise use of the lowest activity capable of producing successful remnant ablation (3). Higher RAI activities [3,700–7,400 MBq (100–200 mCi)] are recommended when there is documented proof
or even a well-founded suspicion of residual microscopic disease. Large, adequately powered, controlled clinical trials are needed to define the relative efficacies of different RAI activities on cancer recurrence rates, disease-specific survival, and early and late complications.
or even a well-founded suspicion of residual microscopic disease. Large, adequately powered, controlled clinical trials are needed to define the relative efficacies of different RAI activities on cancer recurrence rates, disease-specific survival, and early and late complications.
Whole-body 131I Scans
Diagnostic whole-body 131I scanning with adequate TSH stimulation (i.e., hormone withdrawal or rhTSH stimulation) may be done before RRA therapy to verify the completeness of the surgical excision and to detect any foci of uptake outside the thyroid bed. These scan results may be useful for planning and tailoring subsequent management in terms of the need for repeat surgery, indications and 131I dosing for RRA (40). However, its use has been questioned by a number of researchers because in clinical practice it actually seems to have a low impact in on decisions on whether or not to use RRA (41).
Diagnostic 131I whole-body scans are usually performed with up to 185 MBq (5 mCi), and under these circumstances, sensitivity is low for detecting foci of neoplastic tissue. Scan sensitivity is enhanced by using higher activities of radioactivity but the outcome of the subsequent therapeutic procedure can be negatively influenced by the cells’ recent exposure to diagnostic doses of 131I. This exposure can reduce the iodine-trapping function of the thyroid cancer cells, an effect known as “stunning.” It becomes evident a few days after the diagnostic scan and can persist for several weeks, during which time RAI ablation therapy is less likely to be effective (42). Stunning can be minimized by performing diagnostic whole-body scans with doses within the 37–111 MBq (1–3 mCi) range, and radioiodine therapy within 24 to 48 hours following the diagnostic scan, before the iodine-uptake capacity of the tumor cells begins to decline but for practical reasons this is not always feasible. To avoid the risk of stunning, 123I (which does not yield significant tissue damaging β particles) may be used for whole-body imaging (43). Limiting more widespread use of 123I are problems related to the cost and availability in some parts of the world.
A clinician’s choice whether to perform diagnostic scans before RRA should be based on a careful evaluation of the effectiveness of this test in influencing the decision-making process. Further, 131I scintigraphy performed 2 to 10 days after the administration of a therapeutic dose of RAI (posttreatment scan) more effectively allows the identification of any neoplastic foci (44,45). The use of 131I single photon emission computed tomography and computed tomography fusion imaging for this purpose is also producing promising results (46), although further studies are needed before these methods can be considered real alternatives in clinical practice.
RAI Therapy for Local or Distant Metastatic Disease
Radioiodine is the most effective systemic therapy available for DTC patients with metastatic disease. Indeed, it is the only approach that has been demonstrated to significantly improve disease-free (22) and, most important, overall survival (47), which are the main goals of clinical oncology. However, there is also a clear trend toward a more selective use of 131I to treat distant metastatic lesions (10), and once again clinicians are being encouraged to base their decision on a careful risk/benefit analysis. First, not all patients with advanced disease are likely to benefit from RAI, in part due to inadequate or heterogeneous iodine uptake and retention in metastatic foci (47). Second, 131I administration is by no means a risk-free procedure: It can in fact cause important long-term side effects, including secondary malignancy (48). Third, RAI is no longer the only option: New therapeutic alternatives are emerging or undergoing clinical testing, broadening the range of potential choices for effective, individualized treatment of advanced DTC (49).
Indications for RAI Therapy
The question of which patients with advanced metastatic disease should be treated with RAI has not been specifically explored in prospective, randomized, clinical trials. Current recommendations are therefore based on retrospective analyses.
One of the first factors to consider is the RAI avidity of the tumor. In almost one-third of patients with metastatic DTC, no 131I uptake is observed in the metastases themselves. These are the cases with the worst prognosis: 10-year survival rates hover around 10% (see Fig. 50E.1) (47). If metastatic disease lacking RAI uptake is progressing, patients of this type should be considered for clinical trial enrollment or systemic therapy (3,49).
More difficult to define are those patients who are not responding to RAI therapy even though their tumors have retained RAI avidity. Such nonresponders represent about half of all patients with RAI-avid metastatic lesions and close to a third of those with distant metastases. Their 10-year survival rate of 30% is indisputably better than the 10% rate observed in patients whose tumors display no detectable uptake but dismally low when compared with the 90% rate in patients with 131I-avid lesions that disappear after repeated doses of RAI (see Fig. 50E.1) (47).
The likelihood that RAI-avid metastatic lesions will respond to 131I therapy can be anticipated on the basis of clinical and pathologic variables, including the patient’s age, the histology and degree of differentiation of the primary tumors, and site and extension of the metastatic lesions. RAI therapy is most likely to be beneficial for treating small-volume pulmonary metastases discovered in a relatively young patient whose DTC was well differentiated (47,50). The results of 2-[(18)F]fluoro-2-deoxyglucose (FDG) PET also seem to correlate with responsiveness to RAI therapy and, as a result, with the overall DTC prognosis. In particular, FDG uptake by the metastases—even if these lesions are also 131I-avid—is predictive of resistance to RAI therapy (51). Finally, responsiveness to RAI has also been linked to certain genetic and epigenetic events that have been shown to affect 131I uptake (52,53). One of the most important involves activating changes involving the gene that encodes B-type Raf kinase (BRAF), an important component of the mitogen-activated protein kinase pathway. Most studies indicate that BRAF mutations are associated with more aggressive tumors (54). Apart from its proliferation effects, BRAF kinase activation has also been linked to reduced expression of several genes that play key roles in iodine metabolism, with potentially negative repercussions on the tumor’s responsiveness to RAI therapy (54). However, more data are needed before the molecular markers are introduced into clinical practice to predict RAI responsiveness.
Patients with characteristics predictive of tumor response can be managed with repeated doses of RAI administered at 6- to 12-month intervals, as long as they continue to have objective responses. Large lung metastases and bone lesions of any size are less likely to respond to RAI regardless of RAI avidity on whole-body scanning, and enrollment in clinical trials should be considered whenever possible. RAI-avid cervical lymph nodes metastases can also be treated with 131I therapy but RAI is usually less effective when the nodes measure more than 1 cm (41). In these cases, neck surgery is the preferred option, followed by consideration of adjuvant RAI for possible microscopic residual disease. As for smaller, asymptomatic lymph-node metastases, the benefit of treating these lesions has yet to be proven. Finally, there are only limited data supporting the use of RAI therapy for brain metastases but anecdotal reports suggest increased risk for intracranial hemorrhage (55).
Greater than 95% of all remissions occur in patients who have received cumulative 131I doses that do not exceed 22 GBq (600 mCi) (47). Higher cumulative activities significantly increase the risk for secondary malignancies, salivary gland dysfunction, and other complications (see later), so decisions regarding further treatments should be made with caution given a diminishing benefit to risk ratio (48).
Doses and Methods of Administering RAI Therapy
RAI activities for locoregional or metastatic disease can be determined three ways: The empiric fixed-dose approach, quantitative tumor 131I dosimetry, and blood 131I dosimetry. Randomized prospective trials comparing these approaches are lacking.
The most widely used approach involves the empiric administration of fixed amounts of 131I, which usually range from 3,700 to 7,400 MBq (100–200 mCi), depending on the location and extent of distant metastases and the patients age and cardiovascular-renal function status (56). RAI-avid nodal disease may be treated with 5,550 MBq (150 mCi) as an initial activity. Similarly, 5,550 to 6,575 MBq (150–175 mCi) is advocated for initial treatment of pulmonary metastases and 5,550 to 7,400 MBq (150–200 mCi) for skeletal disease. An alternative approach is to administer serial 3,700 MBq (100 mCi) treatments every 3 to 6 months until posttreatment scans are negative or cumulative activity of 22 GBq (600 mCi) is reached (57). The percentage of 131I uptake observed in the metastatic lesions is not considered at all in this approach.
Radiation dosing to tumor from empirically fixed activities of 131I can vary considerably. In contrast, quantitative tumor 131I dosimetry involves estimating the amount of radiation delivered to the lesion per unit of 131I administered. Earlier studies suggested that RAI therapy is less likely to be successful if the radiation dose delivered to the cancerous tissue is less than 3,500 cGy, and that cures can be expected only if the neoplastic tissue receives more than 8,000 cGy (4). The absorbed radiation consists mainly of β emissions, and it depends on the effective half life in the tissue and the radioactive concentration —that is, the ratio of total uptake to the mass of thyroid tissue. Calculations of this sort require serial measurements of the radiation activity in the target lesions using a tracer dose and accurate estimates of tumor size (4).
A third option involves dose calculation based on quantitative blood and whole-body 131I dosimetry to estimate the dose-limiting toxicity to non-target tissues, such as bone marrow. Empirically administered activities of RAI frequently lead to radiation doses to blood that may exceed the maximum tolerated radiation dose (MTRD) of 200 cGy which can lead to bone marrow suppression (58,59). The aim of quantitative blood and whole-body 131I dosimetry is to avoid exceeding the MTRD and therefore prevent marrow suppression. One retrospective study suggested no difference in progression-free or overall survival in patients with distant metastases
treated with whole-body dosimetry compared with empirically selected activities; frequencies of leukopenia and thrombocytopenia did not differ between the two treatment groups but unexpectedly the most severe cytopenias were seen in patients treated dosimetrically (60). In patients whose iodine clearance capacity is reduced (e.g., secondary to renal insufficiency) dosimetry is probably more appropriate. Multiple approaches to estimating the radiation dose to either tumor or marrow have been described, and superiority of one over another has not been demonstrated. Dosimetric methods are available in a limited number of referral centers since they require the skills of a dedicated team of physicists and nuclear medicine technicians and should be ordered only by clinicians who have adequate training and experience in their use.
treated with whole-body dosimetry compared with empirically selected activities; frequencies of leukopenia and thrombocytopenia did not differ between the two treatment groups but unexpectedly the most severe cytopenias were seen in patients treated dosimetrically (60). In patients whose iodine clearance capacity is reduced (e.g., secondary to renal insufficiency) dosimetry is probably more appropriate. Multiple approaches to estimating the radiation dose to either tumor or marrow have been described, and superiority of one over another has not been demonstrated. Dosimetric methods are available in a limited number of referral centers since they require the skills of a dedicated team of physicists and nuclear medicine technicians and should be ordered only by clinicians who have adequate training and experience in their use.
Whatever method is used for dose calculation, RAI therapy requires TSH stimulation to ensure effective uptake of 131I by neoplastic foci. To reduce the unpleasant symptoms of hypothyroidism caused by thyroid hormone withdrawal, placing the patient on liothyronine therapy for 2 to 4 weeks and then withdrawing it for the next 2 weeks has been a traditional approach but a recent randomized study has challenged this assumption (61). The use of rhTSH stimulation instead of hormone withdrawal eliminates the untoward effects of iatrogenic hypothyroidism altogether. This approach has been approved for the preparation of patients undergoing RRA, but its effectiveness in the treatment of metastatic lesions has yet to be demonstrated in a randomized trial and therefore has not been approved for use in the therapy of distant metastases either in the United States or Europe, and has not been recommended in clinical practice guidelines (3). One recent prospective nonrandomized trial suggested that radioiodine scans as well as 124I-PET after preparation with thyroid hormone withdrawal detected more metastatic foci than after rhTSH injections (62). Nonetheless, several other retrospective series point to the potential value of rhTSH preparation for 131I therapy (63,64,65). In a recent study involving 175 patients with RAI-avid metastatic disease followed at the Memorial Sloan-Kettering Cancer Center, similar overall survival rates were observed at 5 years when either thyroid hormone withdrawal or rhTSH was used to stimulate 131I therapy administered following whole-body and blood dosimetry performed (64). Use of rhTSH prior to RAI therapy of metastatic disease is particularly appropriate in those patients with comorbidities that would make iatrogenic hypothyroidism potentially deleterious or those who are unable to produce adequate TSH owing to pituitary disease.
Treatment of Patients with High Serum Thyroglobulin Levels and Negative Imaging Studies
It is widely recognized that some patients who undergo total thyroidectomy and RRA therapy for DTC will subsequently present with exclusively biochemical evidence of persistent or recurrent disease. In these cases, there is no evidence of disease on cross-sectional imaging (e.g., neck ultrasound, computed tomography scan, or magnetic resonance imaging) or functional imaging studies (e.g., diagnostic and/or post-therapeutic RAI scan, or 18-FDG-PET scan) but the patient has detectable Tg levels during LT4 suppression of TSH and/or an increase in Tg levels after TSH stimulation. A recent report indicates that around 20% of all patients present findings of this type on the initial assessment after primary treatment (66).
The question is: Do these findings truly reflect the presence of persistent disease? False positives are usually related to residual Tg production by the remnants of normal thyroid tissue left after primary treatment (i.e., surgery and RRA therapy), but in rare cases they can be caused by heterophile antibody interference with Tg assays (67). The boundaries between normal and neoplastic production are by no means clear although several reports suggest normal cut-off values of 10 ng/mL after LT4 withdrawal or 5 ng/mL after rhTSH stimulation (7,13,17,18). More than absolute values perhaps, increases over time in Tg levels are a highly specific marker of residual/recurrent disease in patients who have undergone postoperative RRA: The positive predictive value in these cases is almost 100% (13,18). Further investigation is needed, however, to identify the rate of change that can be considered predictive of malignancy (16). On the other hand, several studies have found that DTC patients may have detectable Tg values that decrease progressively during the first 2 to 3 years after primary treatment, suggesting a more gradual response to therapy of either the normal remnant or microscopic DTC (13,14,15,17,18). The temporal characteristics of this decline in production probably depend on the volume of these remnants and delayed response to RRA.
In this context, some physicians suggest that RAI therapy be used empirically both to locate and to treat persistent tumor tissue (68). This approach might be considered in patients with no structural evidence of disease if the stimulated Tg values exceed an arbitrary cut-off level (10 ng/mL after thyroid hormone withdrawal and 5 ng/ml after rhTSH stimulation) or rising Tg levels have been documented. If the post-therapy whole-body scan is negative, further RAI administration should not be administered. Using this strategy, serum Tg levels may decline following RAI therapy, but there remains no evidence of improved survival outcomes (12,69) and in studies with appropriate control groups, Tg levels often decline over time even without additional RAI therapies (12). Patients with elevated Tg levels, negative diagnostic or post-therapeutic RAI scans, and evidence of distant metastatic disease on conventional imaging studies or 18-FDG-PET have tumors that have lost their 131I-concentrating ability and therefore also do not benefit from further RAI therapy (51).
Complications of RAI Therapy
Although RAI therapy is reasonably safe, especially when compared with other commonly used adjuvant cancer treatment modalities (e.g., external-beam radiation, cytotoxic chemotherapy), it has become increasingly evident that the procedure is associated with potential complications. They usually involve organs known to concentrate iodine and include both early- and late-onset effects (70). The most commonly observed adverse events affect the salivary glands (sialoadenitis, loss of or changes in taste, xerostomia), eyes (xerophthalmia or epiphora), and gonads (transient reductions in fertility). Recent investigations are challenging traditional concepts of how to minimize these risks; i.e., prophylactic use of lemon juice or lemon candies may not protect against sialoadenitis (71). Fortunately, serious, life-threatening complications, such as acute bone marrow failure or reduced pulmonary function, are rare.
Long-term survivors previously treated with RAI may have a higher incidence of second primary malignancies (72,73). A meta-analysis of these two North American and European studies found that thyroid cancer survivors who had received repeated doses of 131I had a relative risk (RR) of second primary malignancies of 1.19 (95% CI 1.04–1.36; p = 0.010) compared with a healthy control population who had not received RAI, and the likelihood of hematologic malignancies was even higher (RR 2.5; 95% confidence interval 1.13–5.53; p = 0.024) (48). Risk significantly increases when cumulative RAI activities exceed 22 GBq (600 mCi) (48,70). RAI treatments in this cumulative range are usually reserved for patients with locally advanced and/or metastatic disease but therapeutic utility also appears to be limited above this cutoff (47). Although the risk for second primary malignancies is generally felt to be radiation dose dependent, a recent study suggested that the overall risk, while remaining exceptionally low, was increased by 21% in a low-risk DTC population that had been treated only for normal remnant ablation (and presumably with cumulative activities of RAI that were probably in a much lower range) (74).
These data suggest that there is no administered activity of RAI that can be regarded as 100% safe, and in the absence of data demonstrating that adjuvant RAI actually improves survival outcomes in low-risk patients; this finding highlights the need for greater caution in prescribing this treatment.
Follow-Up Paradigms
Just as the initial therapeutic recommendations for surgery and RAI are increasingly tailored to the risk of recurrence and disease-specific mortality, a risk adapted, individualized approach should dictate patient follow-up. In recent consensus guidelines, the most important management issues with regard to the clinical follow-up of DTC patients are based on the risk of recurrence and disease-specific mortality for individual patients (3,29). These issues range from the need for stimulated thyroglobulin (Tg) during follow-up to the role of diagnostic whole-body scans, neck ultrasonography, TSH suppression, and additional therapies.
From a practical management standpoint, the early follow-up (first 2 years after initial therapy) of patients with DTC is based on initial estimates of risk for disease-specific mortality (e.g., AJCC/TNM, AGES, AMES, MACIS, MSKCC, or NTCTCS) (75) and the recently proposed ATA risk of recurrence system. However, long-term management paradigms (more than 2 years after initial therapy) should depend on revised risk estimates that are developed with data obtained during the early follow-up period (response to therapy assessment) (66).
A dynamic risk assessment system has been proposed in which early follow-up recommendations based on initial risk assessments (ATA low, intermediate or high risk of recurrence) are modified with clinical data obtained during the first 2 years of follow-up that provide a response to therapy evaluation (excellent, acceptable, or incomplete response) (66,76). Furthermore, ongoing clinical follow-up is based on an active reassessment of risk in which an individual patient’s response to therapy (excellent, acceptable, or incomplete) may change based on additional data obtained during long-term follow-up.
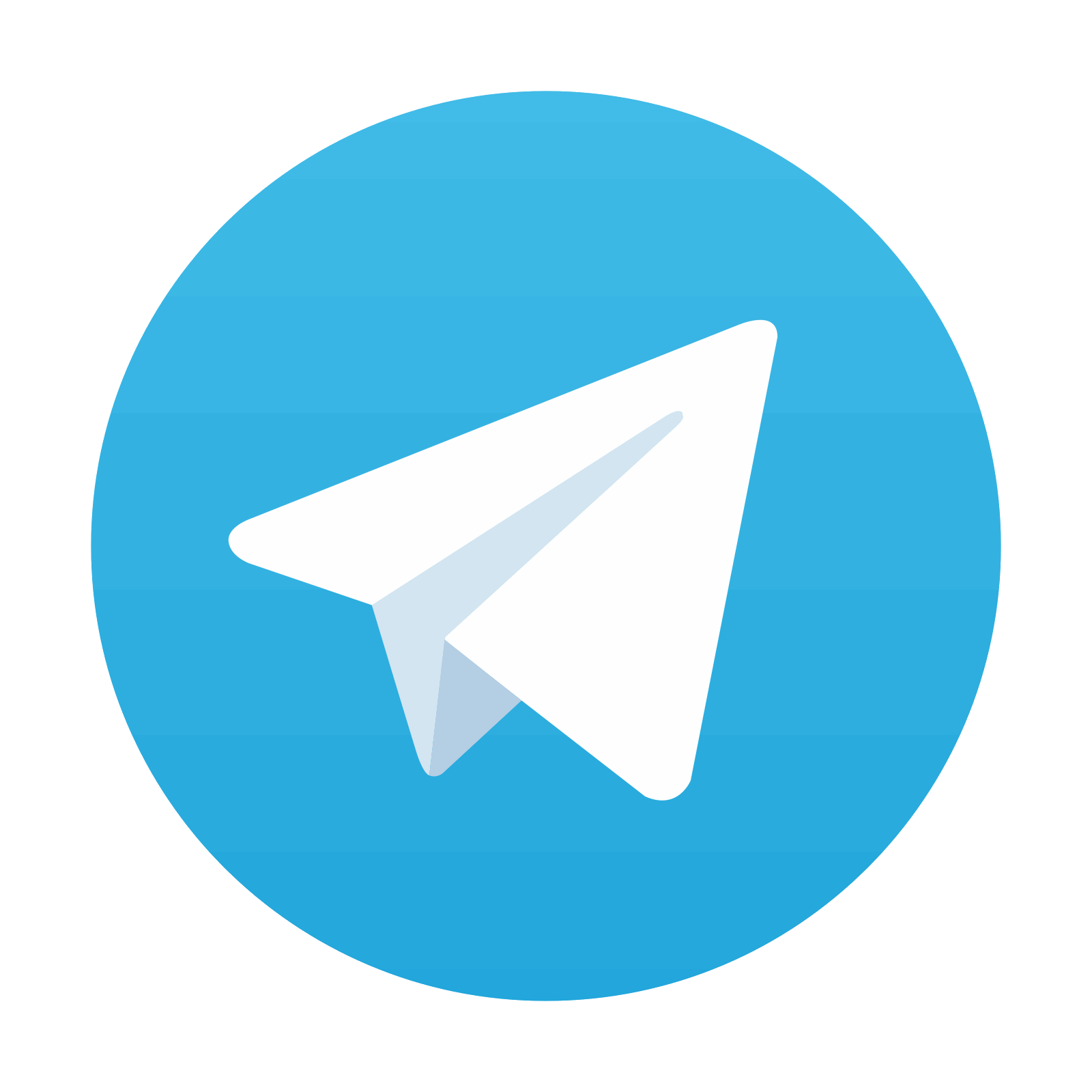
Stay updated, free articles. Join our Telegram channel

Full access? Get Clinical Tree
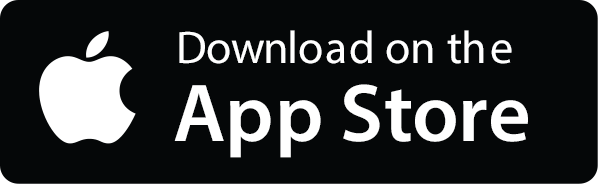
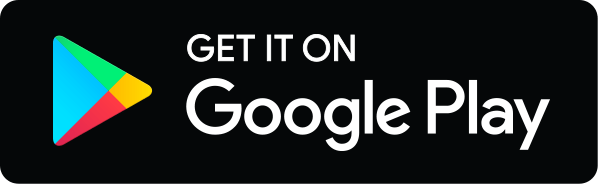