Measurement of Thyroglobulin
Carole Ann Spencer
Introduction
Thyroglobulin (Tg) is the large (660 kDa) dimeric glycoprotein that comprises 75% of the protein content of the thyroid gland and serves as the macromolecular precursor for thyroid hormonogenesis. Because some Tg protein is always released into the circulation as a consequence of thyroid hormone secretion, all subjects with an intact thyroid gland should have Tg detected in their serum. Tg plays a central role in a wide variety of pathophysiologic conditions affecting thyroid function and iodine metabolism. For example, the monoiodotyrosine (MIT) and diiodotyrosine (DIT) residues in the Tg protein backbone stored as colloid serve as iodine reservoirs to offset the effects of intermittent dietary iodine deficiency (1,2). Tg is a key autoantigen involved in thyroid autoimmunity (3), genetic defects in Tg biosynthesis can cause goiter and congenital hypothyroidism (4,5,6,7,8), and the thyroid-specific origin of circulating Tg has led to its use as a postoperative tumor marker for differentiated thyroid cancer (DTC) (9,10,11,12,13).
Over the last decade, Tg immunometric assay (IMA) methodology has improved with the development of more sensitive second-generation methods [functional sensitivities (FS) ≤0.1 ng/mL (μg/L) (see section on Assay Sensitivity) (14,15,16,17,18,19,20)]. Improved assay sensitivity has facilitated the use of basal (non-TSH stimulated) Tg trends for monitoring thyroidectomized DTC patients, thereby minimizing the need for recombinant human thyrotropin (rhTSH)-stimulated Tg measurements (14,15,16,17,18,19,21,22,23). Unfortunately, variability in the numeric values reported by different methods for the same serum remains high (>30%), precluding a change in Tg method during DTC monitoring (17,24,25,26,27). In addition, current Tg IMAs are prone to Tg autoantibody (TgAb) interference that limits the clinical utility of Tg testing for the approximate 20% of DTC patients with circulating TgAb (24,28,29,30). Tg IMAs are also prone to human anti-mouse antibody (HAMA) interferences
that can cause falsely elevated, and occasionally falsely low, Tg IMA values (19,31,32,33). Overcoming these interferences by measuring Tg protein by mass spectrometry, or by measuring Tg messenger RNA (mRNA) in peripheral blood (see section on “Tg mRNA Measured as a Surrogate Tumor Marker”), have not as yet proved useful as tumor marker substitutes for overcoming interferences (34,35,36,37). This chapter focuses on the pathophysiology, methodology, and clinical utility of Tg measurements.
that can cause falsely elevated, and occasionally falsely low, Tg IMA values (19,31,32,33). Overcoming these interferences by measuring Tg protein by mass spectrometry, or by measuring Tg messenger RNA (mRNA) in peripheral blood (see section on “Tg mRNA Measured as a Surrogate Tumor Marker”), have not as yet proved useful as tumor marker substitutes for overcoming interferences (34,35,36,37). This chapter focuses on the pathophysiology, methodology, and clinical utility of Tg measurements.
Factors that Influence Circulating Tg Concentrations
The circulating Tg concentration reflects the net sum of: (a) the mass of Tg-secreting thyroid tissue present (normal tissue and/or tumor), (b) any inflammation of, or injury to, thyroid tissue secondary to fine needle aspiration biopsy (FNAB), surgery, radioactive iodine (RAI) therapy or thyroiditis, and (c) the degree of TSH receptor stimulation (from endogenous TSH, rhTSH, high hCG, or thyroid stimulating immunoglobulins). In patients with DTC, thyroid mass and TSH status are the major factors that influence the interpretation of serum Tg concentrations, as schematically shown in Fig. 13B.1.
Thyroid Mass
Serum Tg concentrations are increased with: (a) simple or nodular goiter secondary to iodine deficiency (38,39), (b) goiter secondary to thyroid dyshormonogenesis (5,8,40), and (c) in many cases of thyroid neoplasia (9,41,42,43). Conversely, a low or undetectable serum Tg is expected with thyroid agenesis, thyroid atrophy, and after thyroidectomy (4,10,11,12,13,44,45). Changes in thyroid tissue mass are reflected by changes in the serum Tg concentration, as seen with relapse following resection of nontoxic goiter (39). Serum Tg also correlates with lymph node mass and number when recurrences are detected in DTC patients (9,14). However, lymph nodes may be inefficient Tg secretors such that Tg can be undetectable despite small lymph metastases (46,47,48,49). This is why current guidelines recommend a combination of Tg measurement and anatomic imaging for postoperative monitoring of DTC (10,11,12,13).
Thyroid Injury
Any source of thyroid injury – FNAB, surgery, RAI treatment, external beam radiation, thyroiditis, or hemorrhage – is expected to raise the circulating Tg concentration (50,51,52,53,54,55). The temporal pattern of the Tg elevation and recovery depends on the severity and cause of injury. Both thyroidectomy and FNAB produce an acute (hours) Tg rise followed by a rapid decline related to the half-life of circulating Tg (∼3 days) and the healing of surgical margins (56,57). RAI produces a slower (days–weeks) rise in Tg secondary to radiolytic tissue damage with a slower resolution (months) (50,54). The rise in serum Tg secondary to the inflammation associated with thyroiditis lasts the longest (∼2 years) (52).
TSH Receptor Stimulation
Retrospective studies report that serum TSH, even within the reference range, correlates with thyroid nodule malignancy risk and stage of disease (58,59). The serum Tg level is exquisitely sensitive to the degree and chronicity of TSH receptor stimulation by TSH, thyroid stimulating immunoglobulins (Graves’
hyperthyroidism), or high hCG (pregnancy). In patients with DTC, the administration of rhTSH, or the high endogenous TSH following thyroid hormone withdrawal, stimulates basal Tg (bTg) on average ten- to twenty-fold, respectively (10,16,19,60,61). In contrast, chronic thyroid hormone suppression of TSH reduces serum Tg approximately by 50% (62) (Fig. 13B.1). In Graves’ disease the stimulating immunoglobulins activate TSH receptors and cause markedly high serum Tg when measured by radioimmunoassay (RIA), but often paradoxically low Tg when measured by IMA because of TgAb interference (see section on “Tg Assay Interference by Tg Autoantibodies”) (63,64). Usually, hCG-related pregnancy changes in Tg are minimal, provided that iodine is adequate and TSH remains within normal limits (65,66,67,68,69). Pregnant patients with DTC may exhibit a small hCG-mediated Tg rise that typically returns to baseline postpartum (70).
hyperthyroidism), or high hCG (pregnancy). In patients with DTC, the administration of rhTSH, or the high endogenous TSH following thyroid hormone withdrawal, stimulates basal Tg (bTg) on average ten- to twenty-fold, respectively (10,16,19,60,61). In contrast, chronic thyroid hormone suppression of TSH reduces serum Tg approximately by 50% (62) (Fig. 13B.1). In Graves’ disease the stimulating immunoglobulins activate TSH receptors and cause markedly high serum Tg when measured by radioimmunoassay (RIA), but often paradoxically low Tg when measured by IMA because of TgAb interference (see section on “Tg Assay Interference by Tg Autoantibodies”) (63,64). Usually, hCG-related pregnancy changes in Tg are minimal, provided that iodine is adequate and TSH remains within normal limits (65,66,67,68,69). Pregnant patients with DTC may exhibit a small hCG-mediated Tg rise that typically returns to baseline postpartum (70).
Tg Measurement: Technical Issues
Quantitative Tg measurements are usually made in serum, cyst, or pleural fluid, or saline rinses of needles used to biopsy suspicious masses (see section on “Tg measured in Cyst Fluid and FNAB needle saline washouts”). Forty years after the first Tg RIA was developed, the assay remains technically challenging (71). Laboratories currently favor non-isotopic IMA methodology that requires shorter incubation times than RIA (hours versus days) and can be automated (Table 13B.1). Manufacturers generally use a two-step approach to overcome “hook” problems that can cause inappropriately low results when high antigen concentrations exceed the binding capacity of the monoclonal capture antibody reagent (72,73). Despite the practical advantages of IMA, technical problems persist, including: (a) wide between-method differences, likely reflecting differences in assay specificity for measuring Tg isoforms (see section on “Assay Standardization/Specificity”); (17,24,74,75), (b) suboptimal functional sensitivity (see section on Assay Sensitivity) (19,61,76), and (c) a high propensity for interferences from HAMA (see section on Human Anti-Mouse Antibody Interferences) (31,32,33,61) and TgAb (see section on Tg Assay Interference by Tg Autoantibodies) (24,28,29,30,72,77,78,79,80). In contrast, RIA methodology is not influenced by HAMA and appears more resistant to TgAb interference than IMA, although TgAb has the potential to cause falsely low or high Tg RIA values with some sera, depending on the specificity of the RIA reagents employed (24,28,29,30,72,77,79,81,82,83).
Assay Standardization/Specificity
As shown in Table 13B.1, some methods are still not directly standardized against the Certified Reference Material (CRM-457). Between-method variability remains high (∼30%)—two-fold higher than within-person variability or the coefficients of variation of measurements made with the same method in different laboratories (17,24,25,26,27). The between-method variability of IMA methods appears higher than for RIA (24). This likely reflects standardization and assay matrix issues, coupled with the employment of monoclonal antibody IMA reagents with narrow epitope specificity for different Tg isoforms, in contrast with the polyclonal antibodies with broader epitope specificities used for RIA (Table 13B.1) (72,84,85,86,87). Tg epitopes are conformational (88,89). There is potential for tumors to secrete abnormal immature Tg isoforms with low iodine and altered carbohydrate content resulting in an abnormal tertiary molecular structure that interacts differently with the antibody reagents of different assays (24,85,86,90,91,92,93,94,95). The wide between-method variability of current assays precludes changing the Tg method during long-term postoperative Tg monitoring of DTC patients. A change in method has the potential to result in a two-fold difference in Tg values that could be interpreted as a clinically significant change [more than 1.5 μg/L (ng/mL)] (76) and either mask disease or prompt unnecessary concern for recurrence. When practical, the archiving of unused specimens for concurrent re-measurement of a past with a current specimen could eliminate run-to-run variability and facilitate re-baselining, should a change in Tg method become necessary.
Reference Range Issues
As seen in Table 13B.1, methods have Tg reference ranges that vary and functional sensitivities (76) that are often close to the lower reference limit (17,24,75). The variability in upper reference limits likely reflects assay specificity differences coupled with the rigor of selecting subjects without thyroid pathology. The reference ranges established for normal control subjects with intact thyroid glands are not relevant to managing thyroidectomized DTC patients unless adjusted for the influence of TSH and the degree of thyroid surgery (76). Specifically, as shown in Fig. 13B.1, lobectomy plus TSH suppression each reduce serum Tg by approximately 50% (62,76). When iodine is sufficient, euthyroid control subjects with normal thyroid glands [∼10 to 15 g (96)] have serum Tg concentrations that average 10 to 13 ng/mL (μg/L) (Table 13B.1) (61,76). It follows that a gram of normal thyroid tissue gives rise to approximately 1.0 μg/L (ng/mL) Tg in the circulation at euthyroid TSH levels. Thus, 1 g of normal thyroid tissue would be expected to produce 1.0 ng/mL (μg/L) Tg in the circulation when TSH is normal. Given the ten- to twenty-fold stimulation expected during high TSH states, the 1 to 2 g thyroid remnant typically remaining following near-total thyroidectomy (97) would be expected to produce a serum Tg below ∼2.0 μg/L (ng/mL) in the absence of TSH stimulation, and below ∼20 μg/L (ng/mL) 72 hours after rhTSH administration or thyroid hormone withdrawal (Fig. 13B.1) (10).
Assay Sensitivity
The use of IMA methodology did not initially improve Tg assay sensitivity, as had been the case for TSH (17,24,75,98). Assay detection limits were difficult to compare before the protocol for determining a realistic estimate of the detection limit (functional sensitivity) was established (76). Functional sensitivity is now defined by guidelines as: “the serum Tg value that can be measured with 20 percent between-run precision over a period of more than 6 months using at least two different reagent lots and two different calibrator lots” (76). Most current assays remain first-generation, defined as having a functional sensitivity between 0.5 and 1.0 μg/L (ng/mL) (Table 13B.1) (17,24,71,75). The sensitivity limit of
such assays is only marginally below the reference range for euthyroid controls with intact thyroid glands and thereby suboptimal for detecting small amounts of persistent/recurrent tumor remaining after thyroidectomy for DTC (Fig. 13B.2). During the last decade, more sensitive second-generation Tg IMA methods [functional sensitivities ≤ 0.1μg/L (ng/mL)] have become available (14,15,16,17,19,24,61,75). Despite some controversy concerning how to interpret low but detectable Tg values in the 0.1 to 1.0 μg/L (ng/mL) range that were hitherto believed to be “undetectable” (10,11,12,13,18), there is growing recognition that the need for rTSH-stimulation is reduced by using a second-generation method to monitor bTg trends (14,15,16,17,19,20,22,23,61,75,99,100).
such assays is only marginally below the reference range for euthyroid controls with intact thyroid glands and thereby suboptimal for detecting small amounts of persistent/recurrent tumor remaining after thyroidectomy for DTC (Fig. 13B.2). During the last decade, more sensitive second-generation Tg IMA methods [functional sensitivities ≤ 0.1μg/L (ng/mL)] have become available (14,15,16,17,19,24,61,75). Despite some controversy concerning how to interpret low but detectable Tg values in the 0.1 to 1.0 μg/L (ng/mL) range that were hitherto believed to be “undetectable” (10,11,12,13,18), there is growing recognition that the need for rTSH-stimulation is reduced by using a second-generation method to monitor bTg trends (14,15,16,17,19,20,22,23,61,75,99,100).
Table 13B.1 Characteristics of Current Tg Assays | ||||||||||||||||||||||||||||||||||||||||||||||||||||||||||||||||||||||||||||||||||||||||||||||||||||||||||||||
---|---|---|---|---|---|---|---|---|---|---|---|---|---|---|---|---|---|---|---|---|---|---|---|---|---|---|---|---|---|---|---|---|---|---|---|---|---|---|---|---|---|---|---|---|---|---|---|---|---|---|---|---|---|---|---|---|---|---|---|---|---|---|---|---|---|---|---|---|---|---|---|---|---|---|---|---|---|---|---|---|---|---|---|---|---|---|---|---|---|---|---|---|---|---|---|---|---|---|---|---|---|---|---|---|---|---|---|---|---|---|
|
Interferences
An unintended consequence of adopting IMA methodology for Tg measurement has been an exacerbation of interference problems, involving both HAMA (31,32,33) and TgAb (24,28,29,30,101,102). Whereas HAMA interference usually results in a falsely high serum Tg that may prompt unnecessary investigations for disease (32,33), TgAb interference causes falsely low or undetectable Tg IMA measurements that have more serious consequences by masking the presence of disease (24,28,101,102,103,104). Whereas blocker reagents are added to IMA methods to minimize HAMA interference (19,31,105), there are still no reliable measures to overcome TgAb interference with Tg measurements.
Human Anti-Mouse Antibody Interferences
HAMA has the potential to interfere with noncompetitive IMA methodology that uses murine-derived monoclonal antibody (MAb) reagents (Table 13B.1) (31,32,33). Specifically, HAMA–MAb interactions can create a false signal that simulates, or in rare cases, masks, the presence of Tg antigen (31,32,33). This problem has the potential to negatively impact patient care by prompting unnecessary imaging or RAI treatment or masking the presence of persistent/recurrent disease (31,32,33). In contrast, competitive RIA methodology that uses high affinity polyclonal antibodies (typically raised in rabbits) is not influenced by HAMA (106). Patients handling animals or receiving MAbs for diagnostic imaging or cancer therapy are especially prone to develop HAMA (107). HAMA prevalence in general averages 5% to 10%, (108). However, the frequency of interference is generally much lower (0.05% to 3.0%) (109) because manufacturers add blockers to reduce HAMA interactions.
Currently, HAMA interference with Tg IMA measurements approximates 0.5% (19,105). HAMA interference should be suspected when Tg: (a) appears discordant with clinical status, (b) fails to rise with TSH stimulation or fall with TSH suppression, (c) is discordant when measured by different methods, or (d) is lowered when re-measured in a blocker (HBT) tube (Scantibodies, Santee, CA, USA) or after polyethylene glycol precipitation (19,31,110). There is no entirely reliable method for detecting or eliminating HAMA interference (111).
Currently, HAMA interference with Tg IMA measurements approximates 0.5% (19,105). HAMA interference should be suspected when Tg: (a) appears discordant with clinical status, (b) fails to rise with TSH stimulation or fall with TSH suppression, (c) is discordant when measured by different methods, or (d) is lowered when re-measured in a blocker (HBT) tube (Scantibodies, Santee, CA, USA) or after polyethylene glycol precipitation (19,31,110). There is no entirely reliable method for detecting or eliminating HAMA interference (111).
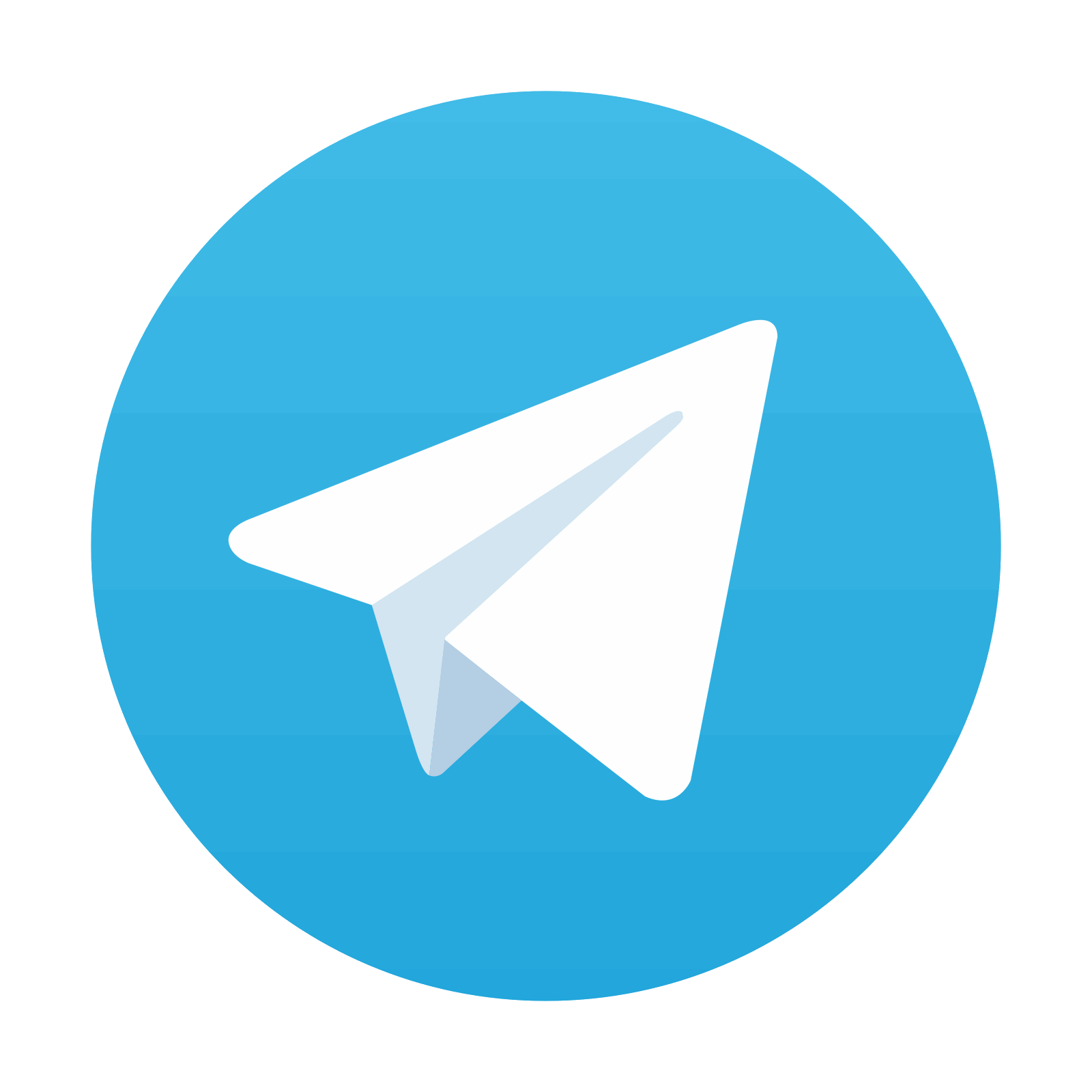
Stay updated, free articles. Join our Telegram channel

Full access? Get Clinical Tree
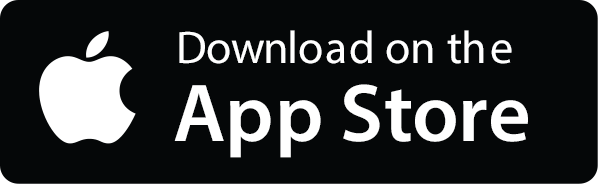
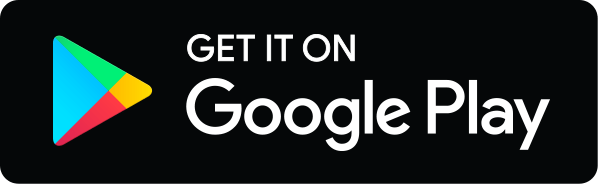
