Radiation is a frequent adjunct for cancer treatment. Over 1.5 million persons have been diagnosed with cancer in the United States and of these, over two-thirds require radiation treatment.1 Radiation is frequently combined with surgery to assist with local control. Furthermore, it can reduce the dosage of chemotherapy required through a synergistic effect. It is more commonly used in breast and lung tumors due to contiguous spread of disease or inability to achieve completely negative margins. It also plays a role in the treatment of recurrent and metastatic tumors of other cell-line etiologies including lymphomas. Physiologically, radiation induces damage to the double-stranded DNA through the generation of free radicals.
While radiation therapy techniques have advanced over the 100 years of its existence, it continues to impact normal tissue in its path. Radiation injures small vessels thereby decreasing oxygen delivery to normal tissues. It also causes fibrosis of surrounding tissue, further diminishing oxygen delivery.2 This directly damages the quality of the tissue present in an irradiated area and worsens its ability to respond to environmental or physiologic stresses. Complications associated with radiation to the chest wall include ulceration of the skin and soft tissue with resultant infection, rib fracture, osteonecrosis, recurrent tumor, and pulmonary fibrosis to name a few.
Chest wall tumors frequently require a multidisciplinary approach. Because local soft tissues become fibrotic following irradiation, these tissues are often not amenable to primary closure or local tissue rearrangements to achieve a closed wound. Irradiated chest wall defects following tumor resection frequently require the incorporation of well vascularized, nonirradiated tissue in the form of flaps. Despite the delivery of well-vascularized tissue, chest wall reconstruction in the setting of radiation remains a complex proposition with higher than normal complication rates.3
The chest wall provides protection to intrathoracic and intra-abdominal organs, supports shoulder and upper extremity function, and provides a platform for respiratory muscles to increase or decrease intrathoracic pressure to stimulate air exchange.4 Chest wall reconstruction involves combining two goals: restoration of functional integrity and aesthetics.
Prior to any reconstructive effort in an irradiated field, it is important to establish clear margins. In addition, any infection must be cleared with serial debridement and IV antibiotics. Inadequate debridement may lead to persistent drainage.5 Once the final defect is established, it is important to assess the size and location of defect, depth of defect, quality of surrounding tissues, and patient prognosis.6 These factors will guide the selection of an optimal reconstructive method while also providing for potential contingencies. Knowledge of a wide variety of reconstructive options allows for adaptation to potential intraoperative developments.
The size and location of the defect will help to determine what will likely be affected as part of the resection plan. Based on excision location, the ideal method to achieve closure can be determined. Especially in the setting of radiation where local tissue is damaged, the transfer of well-vascularized regional or distant tissue is paramount to achieve proper coverage of vital structures that may be exposed. Defect thickness can be divided into partial versus full thickness. Partial-thickness defects involve soft tissue alone while full-thickness defects involve both soft tissue and bone. While some superficial and partial thickness wounds may be successfully treated with skin grafts or local tissue rearrangements, radiation damage to these wound beds may impair the circulation and subsequent graft take or limit the mobility and perfusion of local flaps. The presence of a bony defect adds to the complexity of the reconstruction, requiring this component to also be addressed.
The surrounding tissue quality will help to determine which tissues are available for reconstruction. Prior scars may preclude the use of certain vascularized flaps. Extensive radiation fibrosis will necessitate the use of regional or distant tissue for reconstruction while mild changes may allow for use of local tissue rearrangements. Strong consideration should also be given to resecting this fibrotic tissue, thus creating an even larger defect for reconstruction. If an infection is present, the patient may be better served with control of the infection with IV antibiotics and serial debridement of the soft tissue. Vacuum-assisted closure (VAC) therapy may serve as a bridge to definitive reconstruction.2
It is important to consider the overall patient prognosis. The need for postoperative chemotherapy or radiation treatment may influence what type of reconstruction is chosen. A skin graft does not provide as robust coverage as a flap when subjected to postoperative radiation. Additionally, if a patient is being seen for palliation, consideration should be given to the simplest and quickest reconstructive option that will provide the patient the greatest degree of comfort, not necessarily the most durable solution.
Skeletal defects occur when resection is full-thickness and involves excision of sternum or ribs. The impact on respiratory physiology increases with increased sternal loss. If greater than four ribs are resected anteriorly or 5 cm of lateral chest wall is resected, it would frequently require bony stabilization to support respiratory function.
Skeletal reconstruction plays a profound effect on improving respiratory physiology. Studies demonstrate reduced ventilator dependence and overall hospital stay with adequate rigid fixation of the chest wall.7 It is important to balance the benefits of rigid chest wall reconstruction with the risk of infection and other patient comorbidities. Anterior and lateral chest wall defects typically require stabilization of the chest wall. Posterior and apical chest wall defects do not typically require reconstruction because of the natural parietal suspension by the scapula or clavicle, respectively. Skeletal stabilization can be accomplished through a variety of techniques using mesh, methyl methacrylate, and osteosynthesis systems.
Mesh, whether biologic or synthetic, is commonly used as a component of full-thickness chest wall reconstruction involving the resection of bone. It provides a physical barrier to fluid and air when stretched over a defect. It can also serve to provide stability of the thoracic cavity following bony excision. Typical synthetic nonabsorbable products such as polypropylene, polyester, polytetrafluoroethylene, or knitted meshes are easy to use, but require adequate soft tissue coverage to be successful. Being synthetic, they are more prone to infection due to the ability of bacteria to form adherent biofilms. They do not allow local soft tissue in-growth and are not incorporated into the native tissue. For this reason, they are not used in contaminated fields and have less of a role in reconstruction of irradiated wounds.
Biologic meshes, whether xenograft or allograft, regardless of source (porcine, bovine, or human) and the propriety process used to produce them, act as scaffolds for tissue ingrowth.4 This is the primary advantage of biologic meshes over synthetic nonabsorbable products, which are gradually incorporated into the host tissue and are thus rendered more resistant to infection. In addition to greater cost of biologic meshes compared to synthetics, their main disadvantage is the risk of material laxity in the future which may require intervention. More highly cross-linked and thicker biologic products have less stretch, and also require longer times to revascularize. As of this time, there is no randomized, head-to-head comparison of synthetic versus biologic meshes for chest wall reconstruction in humans.
Methyl methacrylate may be sandwiched between mesh, typically Marlex, to provide greater stability to larger bony chest wall defects.8 It can be shaped to conform to any chest wall skeletal defect and uses the mesh as points of fixation to the surrounding tissue. Because of associated seromas, its use is limited by higher rates of infection.
There are a variety of osteosynthesis systems available using shaped plates secured with screws to provide rigid bony fixation.9 They are best for sternal defects or bridging rib segments. The systems are typically made of titanium which resists corrosion and metal fatigue. Rib-plating systems have also been used to improve the aesthetic appearance of the chest wall in cases in which rib resection has been performed for access to the internal mammary vessels during microsurgical breast reconstruction.10
At this time, there is no clear evidence which skeletal fixation (mesh, methyl methacrylate, osteosynthesis) method is best to achieve skeletal stability. However, the main source of morbidity with full-thickness chest wall resection remains pulmonary complications; thus, it remains paramount to focus on good pulmonary toilet postoperatively.11 The second source of complications is wound related, such as hematoma, seroma, infection, and delayed wound healing. This can lead to prosthesis removal in a small percentage of cases.
Large soft tissue deficits can occur with chest wall resection. Due to exposure of critical structures, primary closure or split-thickness skin grafting is not a viable option. In addition, the poor vascular bed created due to radiation requires healthy, vascularized tissue to be imported into the field. The pectoralis major, latissimus dorsi, rectus abdominis, and great omentum are the four workhorse flaps to provide soft tissue coverage.12 These flaps are popular for their reliability, consistent anatomy, ease of harvest, and versatility. The muscle flaps may be used alone and covered with a split-thickness skin graft or as a composite musculocutaneous flap. If postoperative radiation is planned, musculocutaneous flaps are preferred.
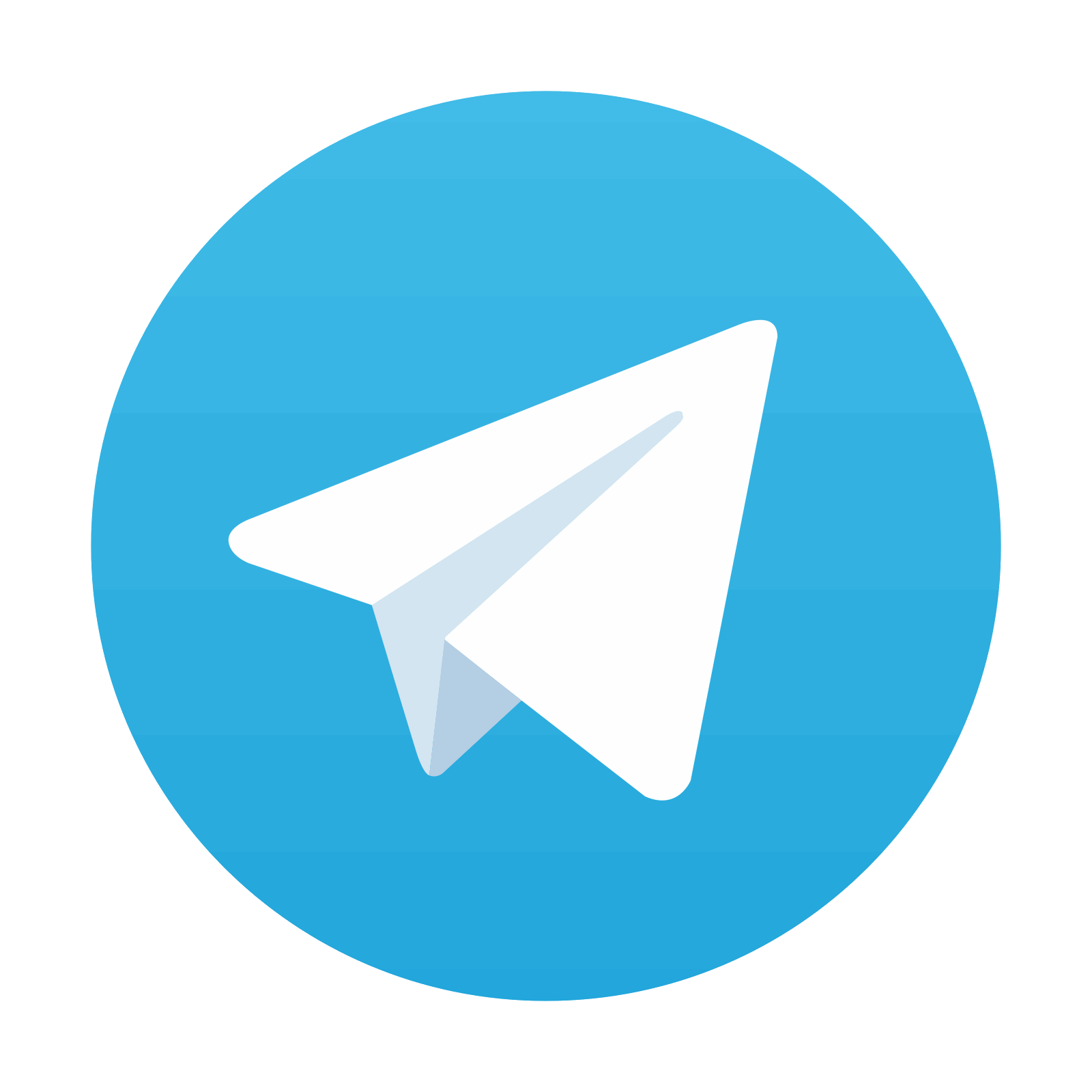
Stay updated, free articles. Join our Telegram channel

Full access? Get Clinical Tree
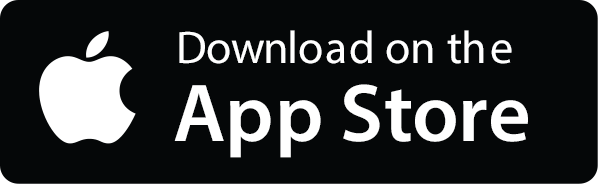
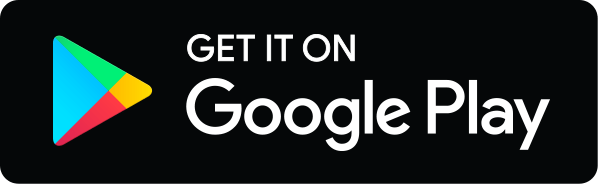