Management of Other High Risk Patients
Seema A. Khan
INTRODUCTION
Breast cancer risk can be attributed to three major sources: increasing age, lifetime estrogen exposure (endogenous and exogenous), and genetic susceptibility. These major factors play a critical role in breast epithelial transformation and appear to converge in the phenotypic expression of proliferative precursor lesions of the breast epithelium, as observed in the unaffected breasts of women who are genetically susceptible (e.g., BRCA mutation carriers) as well as women at risk for the so-called “sporadic” breast cancer. This chapter will focus on the identification and management of women at higher than average risk for sporadic breast cancer; management of women who are genetically susceptible and those with lobular carcinoma in situ is covered in Chapters 12 and 16. The following discussions of breast cancer “risk” will apply to women who do not carry mutations of known breast cancer susceptibility genes. Additionally, a full discussion of the epidemiology of sporadic breast cancer can be found in Chapter 18, along with pertinent references; these risk factors are reviewed here only from the perspective of identification and management of individuals (rather than populations) at risk for breast cancer.
The management of women at increased risk for breast cancer includes: a) identification of high risk women (or risk estimation); b) recommendations for life-style modifications that may reduce risk; c) a plan for breast surveillance; d) discussion of pharmacologic prevention; and e) discussion of prophylactic mastectomy (if appropriate and sought by the patient).
IDENTIFICATION OF HIGH RISK WOMEN
Age: The relation of age to breast cancer risk is discussed fully in Chapter 18 but it is worthwhile noting here that breast cancer risk estimation for individuals is heavily influenced by age. Incidence rates rise sharply with age, starting in the mid-to-late 30s, and all currently used statistical risk estimation models relate the known relative risk (RR) for a particular risk factor to the age-specific breast cancer frequency in that population. This is important to recognize in terms of breast cancer risk management, as a 5-year risk estimate of 1.7% using the Gail model-2 (National Cancer Institute (NCI) Breast Cancer Risk Assessment Tool) has been widely used as a benchmark measure of breast cancer risk. The 5-year breast cancer Gail model probability for an average 45-year-old woman is 1%, while the similar estimate for an average 65-year-old woman is 2%. Thus a 45-year-old woman who has a 5-year breast cancer probability of 1.7% is at higher life-time risk than her 65-year-old counterpart. However, as the same 45-year-old woman ages, if she does not acquire any new risk factors and does not develop breast cancer, her estimated risk declines and approaches the average risk for her age group. Thus longer-term risk estimates (e.g., 10-or 20-year estimates) may be a more useful framework for risk counseling, and life-time risk estimates (calculated to age 85 or 90) have become a commonly used benchmark for comparison of risk groups, most useful for younger women.
Risk Factors Related to Reproductive Hormones: A wide array of established risk factors for breast cancer relates to endogenous life-time estrogen exposure. These include young age at menarche, late age at first full-term pregnancy,
no exposure to lactation, and late age at menopause. Among postmenopausal women, higher serum estradiol levels are robustly linked to breast cancer risk, and among premenopausal women there is a suggestion of higher levels of the androgenic estradiol precursors in breast cancer cases but these differences are not sufficient to utilize serum hormone levels for risk estimation in clinical practice. Although the risk associated with reproductive variables has been generally attributed to estrogen exposure, the hormones progesterone and prolactin also deserve consideration. Thus, the number of ovulatory cycles during a woman’s reproductive life-span (with their luteal phase progesterone surge) is a stronger determinant of breast cancer risk than the length of time from menarche to menopause, and the short-term increase in breast cancer risk following pregnancy is at least partially attributed to the high progesterone exposure of pregnancy. Furthermore, exogenous progestin exposure in the form of combination postmenopausal hormone therapy (CMHT) carries a larger breast cancer risk than therapy with estrogen alone. In premenopausal women, current oral contraceptive use has long been associated with a modest increase in breast cancer risk, but recent data on the use of depo-medroxyprogesterone acetate (DMPA) shows a twofold increase in breast cancer risk, with a trend toward higher grade and triple negative tumors (1). Prolactin exposure, too, is now clearly implicated in breast cancer causation, and the protective effect of pregnancy is mediated, at least partially, through a long-term lowering of serum prolactin. Epidemiological studies have shown that breast cancer cases demonstrate higher serum prolactin levels than controls, prior to the onset of breast cancer.
no exposure to lactation, and late age at menopause. Among postmenopausal women, higher serum estradiol levels are robustly linked to breast cancer risk, and among premenopausal women there is a suggestion of higher levels of the androgenic estradiol precursors in breast cancer cases but these differences are not sufficient to utilize serum hormone levels for risk estimation in clinical practice. Although the risk associated with reproductive variables has been generally attributed to estrogen exposure, the hormones progesterone and prolactin also deserve consideration. Thus, the number of ovulatory cycles during a woman’s reproductive life-span (with their luteal phase progesterone surge) is a stronger determinant of breast cancer risk than the length of time from menarche to menopause, and the short-term increase in breast cancer risk following pregnancy is at least partially attributed to the high progesterone exposure of pregnancy. Furthermore, exogenous progestin exposure in the form of combination postmenopausal hormone therapy (CMHT) carries a larger breast cancer risk than therapy with estrogen alone. In premenopausal women, current oral contraceptive use has long been associated with a modest increase in breast cancer risk, but recent data on the use of depo-medroxyprogesterone acetate (DMPA) shows a twofold increase in breast cancer risk, with a trend toward higher grade and triple negative tumors (1). Prolactin exposure, too, is now clearly implicated in breast cancer causation, and the protective effect of pregnancy is mediated, at least partially, through a long-term lowering of serum prolactin. Epidemiological studies have shown that breast cancer cases demonstrate higher serum prolactin levels than controls, prior to the onset of breast cancer.
Other well-established risk factors also appear to operate through the endocrine axis: postmenopausal obesity is associated with increased aromatization of androgenic precursors in adipose tissue, lower sex hormone-binding globulin, and higher free estradiol. Physical activity in adolescence delays menarche and the onset of ovulatory cycles; later in life, it protects against obesity and may operate through other mechanisms as well. Moderate to heavy alcohol consumption is associated with higher circulating sex steroid levels and may retard the hepatic metabolism of hormones.
In general, the contribution of these individual risk factors to overall risk is modest, and relative risk estimates for each of these factors from most studies are in the range of 1.5-2.0. It is therefore difficult to apply this information to individual risk estimation unless it is incorporated into multifactorial statistical models, the prototype of which is the Gail model (2), validated in the Breast Cancer Prevention Trial (3). More recently developed models (Tyrer-Cusick) do include additional endocrine risk factors (age at menarche, use of postmenopausal hormone therapy, height, weight) and family history (4). Breast cancer risk estimation is discussed more fully below.
Mammographic Density
The radiographic appearance of the breast varies according to differences in the relative distributions of fat and fibroglandular tissues, where fat appears dark and radiographically dense areas appear light. It is reported on mammography reports using the standard reporting lexicon as BIRADS 1 through 4 (mostly fatty, scattered densities, heterogeneously dense, or very dense). These categories are intended to correspond to the percent dense area (<25%, 25%-50%, 51%-75%, and >75%) measured by application of a computer algorithm to digitized film screen images. Much of the published literature is based on a semiautomated algorithm (the CUMULUS software, developed by Byng et al.) but additional algorithms based on publically available software are being developed as shown in Figure 19-1. Volumetric, three-dimensional measurements are also being tested and may be more closely related to breast cancer risk. Mammographic density is at least partially genetically determined and a recent genome-wide association study has shown that polymorphisms associated with mammographic density are also associated with breast cancer risk (5).
There is now a substantial body of evidence showing that extensive mammographic density is strongly associated with an increased risk of breast cancer. The relative risk for the highest category of breast density (>75% of breast area is dense) has been reproducible across several large studies, and, on meta-analysis of these, the RR for the highest density category ranges from 3.25 to 6.49 (6). Additionally, a combined analysis of three nested case-control studies which included 1,112 participants of breast cancer screening programs (7) showed that women with mammographic density of more than 75% had a breast cancer RR of 4.7 (95% CI 3.0,7.4) compared to women with mammographic density less than 10%. In particular, women with the highest density category had a very high risk of being diagnosed with breast cancer within 12 months of the screening mammogram (RR = 17), suggesting that high mammographic density might mask the cancer. Among postmenopausal women, the association of percent dense area with breast cancer risk is stronger for those using hormone therapy; other data suggest an interaction between alcohol use of more than 1 drink daily and mammographic density. Based on these results, it is important to identify women with high percent density to counsel them about modifiable risk factors. Research on alternative imaging techniques for earlier detection is also needed.
The importance of mammographic density as a strong and independent risk factor for breast cancer is amplified by its high prevalence, with about one-third of the general population of women displaying dense areas of 50% or greater on mammography. Because of this prevalence, the fraction of breast cancer cases attributable to high mammographic density is in the range of 16% to 32% and higher in premenopausal women (7). Thus, the impact of breast density on cancer risk is far stronger than any of the known endocrinerelated risk factors and in the same range as the risk associated with atypical proliferative lesions of the breast.
Studies examining the correlation between serum sexsteroid levels and mammographic density have not shown any consistent associations, with one large study by Verheus et al. in 2007 showing no relationship between circulating sex steroid levels and mammographic density but a more recent examination of hormonal variation in premenopausal women showing a positive association with mean estrogen level through the menstrual cycle. It would be of interest to determine if local breast estradiol levels (e.g., those in nipple aspiration fluid) are related more closely to breast density than circulating levels; these studies are ongoing. On the other hand, mammographic density and serum sex steroids (estradiol and testosterone) may have independent and additive effects on breast cancer risk as suggested by a recently reported case-control study nested within the Harvard Nurses’ Health Study. Other hints that mammographic density is modulated by the endocrine environment come from the significant correlation between mammographic density and serum insulin-like growth factor-1 (IGF-1) that has been observed in premenopausal women (8). Prolactin is a potentially important hormone in breast carcinogenesis—higher levels in the serum are associated with increased breast cancer risk in both pre- and postmenopausal women—and several studies now suggest an association between serum prolactin levels and mammographic density.
![]() FIGURE 19-1 Mammographic density measurement. Examples of processed images. (A) An example of a digitized mammogram before and after thresholding and application of the watershed algorithm (one image, one particular thresholding algorithm, Moments), and the same image thresholded by using Cumulus (B). Discriminatory powers of Cumulus and ImageJ for predicting breast cancer risk, as measured by area under curve (AUC). Legend on the top-left corner summarizes P values for the Delong test between two receiver operating characteristic (ROC) curves. Legend on the bottom-right corner summarizes the AUC for each model with corresponding 95% confidence intervals. There were 3,593 participants with eligible film mammograms (1,784 cases and 1,809 controls). (From Li et al. High-throughput mammographic-density measurement: a tool for risk prediction of breast cancer. Breast Cancer Res 2012;14(4):R114.) |
Studies relating breast density to histological findings have shown that high mammographic density is associated with an increased risk for atypical hyperplasia. However, in breast epithelial samples obtained from high risk women using random fine needle aspiration, no correlation was seen between cytologic atypia or cell proliferation (measured by Ki-67 labeling) and mammographic density. Quantitative microscopy of the autopsied breast shows relationships between mammographic density and total nuclear area, epithelial and nonepithelial nuclear area, glandular structures, and amount of collagen. Similarly, in reduction mammoplasty samples, the epithelial cell volume was concentrated in areas of high density connective tissue, and was significantly related to the mammographic density. This increased epithelial and stromal area in dense tissue does not translate into increased cell proliferation or steroid receptor expression in epithelial cells. Thus, there is no clear biological explanation for the association of breast density with cancer risk, and much remains to be done in order to incorporate the measurement and modulation of mammographic density into algorithms for breast cancer risk assessment.
An important, and potentially the most useful, aspect of breast density is the possibility that it is modifiable. This is most evident by the fact that the use of combination
postmenopausal hormone therapy (PMHT) increases percent breast density whereas tamoxifen therapy not only reduces it, but a decrease in mammographic density appears to predict preventive benefit. In a post-hoc sub-study of the International Breast Cancer Intervention Study (IBIS) trial, women who demonstrated a 10% or greater reduction in density following 12 months of tamoxifen use demonstrated a two-thirds reduction in breast cancer risk, whereas no significant risk reduction was seen in women whose mammographic density did not change (9). Small studies of ovarian suppression with a gonadotrophin-releasing hormone agonist in premenopausal women, and in BRCA1 mutation carriers, suggest another avenue of breast density reduction. Other data are more mixed; a meta-analysis of physical activity and mammographic density showed no significant effect, and vitamin D supplementation in the Women’s Health Initiative similarly had a null effect. Aspirin use, which appears to reduce breast cancer risk, shows no relationship to breast density. Therefore, although modification of breast density is possible, not all pathways of breast cancer risk reduction are reflected as reductions in breast density.
postmenopausal hormone therapy (PMHT) increases percent breast density whereas tamoxifen therapy not only reduces it, but a decrease in mammographic density appears to predict preventive benefit. In a post-hoc sub-study of the International Breast Cancer Intervention Study (IBIS) trial, women who demonstrated a 10% or greater reduction in density following 12 months of tamoxifen use demonstrated a two-thirds reduction in breast cancer risk, whereas no significant risk reduction was seen in women whose mammographic density did not change (9). Small studies of ovarian suppression with a gonadotrophin-releasing hormone agonist in premenopausal women, and in BRCA1 mutation carriers, suggest another avenue of breast density reduction. Other data are more mixed; a meta-analysis of physical activity and mammographic density showed no significant effect, and vitamin D supplementation in the Women’s Health Initiative similarly had a null effect. Aspirin use, which appears to reduce breast cancer risk, shows no relationship to breast density. Therefore, although modification of breast density is possible, not all pathways of breast cancer risk reduction are reflected as reductions in breast density.
Breast Epithelial Hyperplasia and Involution
The present paradigm for the development of breast cancer suggests that in the breast, as in other epithelial organs, the etiologic pathways of malignancy converge in the occurrence of breast epithelial hyperplasia or intraepithelial neoplasia (IEN). This concept is supported by data going back several decades, relating benign breast disease (specifically epithelial proliferation) to an increased risk of subsequent breast cancer; several studies have shown that the breast cancer risk of women with hyperplasia without atypia is roughly twofold greater than women without; and the risk associated with atypical hyperplasia is increased approximately fourfold (10, 11). The specific lesions included in the category of hyperplasia without atypia consist of moderate or florid duct hyperplasia, intraductal papilloma, sclerosing adenosis, and radial scar. The lesions usually included in the category of atypical proliferation include atypical duct hyperplasia (ADH), atypical lobular hyperplasia (ALH), atypical papilloma, and flat epithelial atypia (see Chapter 9 for more detail). Although data from the large retrospective cohort from Nashville, TN, showed a strong interaction between atypical hyperplasia and a positive family history for breast cancer (the risk associated with a history of first degree relative with breast cancer and atypical hyperplasia was increased almost 10-fold), two recent studies do not substantiate these findings and show no added increase in risk for women with atypical hyperplasia and a positive family history (11, 12). Although there was a suggestion of a more marked risk increase with ALH than ADH in the Nashville and Nurses cohorts, recent results from the Mayo Clinic cohort do not show a difference in risk between ALH and ADH. However, an increasing number of atypical foci, the presence of calcifications in the histological material, and the combination of ADH and ALH, all raise risk significantly over that observed in the absence of these findings (Fig. 19-2).
![]() FIGURE 19-2 (A) Cumulative risk of breast cancer over time. Observed cumulative breast cancer incidence among women with atypical hyperplasia, with 95% represented by stippled lines. Expected breast cancer events were calculated by applying age- and calendar period-stratified person-years of observation to corresponding lows Surveillance, Epidemiology, and End Results breast cancer incidence rates. Observed and expected events cumulated after accounting for death as a competing. (B) Observed and expected cumulative breast cancer incidence among women with atypical hyperplasia, stratified by number of foci of atypia and histologic presence of calcifications. (From Degnim AC, Visscher DW, Berman HK, et al., Stratification of breast cancer risk in women with atypia: a Mayo cohort. J Clin Oncol 2007;1;25(19):2671-2677. Epub 2007Jun 11. (A is Fig 2; B is Fig 3, both on page 2675.)) |
Despite the consistently increased risk seen with typical and atypical epithelial proliferation in the breast, most women with these findings do not, in fact, develop breast cancer (e.g., 80% of the 330 women with atypical hyperplasia in the Mayo cohort remained free of breast cancer over a mean follow-up period of almost 14 years). A number of authors have attempted to identify molecular factors which might improve the specificity of risk assessment, so that preventive interventions can be targeted to a more purified high risk group. Despite a number of potential risk biomarkers, there has been little progress in prospective validation of these. The more obvious immunohistochemical markers estrogen receptor, p53, and HER-2/neu demonstrate risk increases of two- to threefold but have not been validated and are not in clinical use. Expression of cyclooxygenase-2 (COX-2) has been examined in atypical lesions from women in the Mayo cohort, with an observed increase in breast cancer risk with increasing COX-2 expression. Thus, if expression was weak or absent, RR was 2.63 (95% CI 3.0,7.4); with
moderate COX-2 expression, RR was 3.56 (95% CI, 1.94, 5.97) and with strong expression, RR was 5.66 (95% CI, 2.59, 10.75). Although these findings have not been replicated, the fact that COX-2 expression is also a marker of increased recurrence risk in DCIS lesions strengthens the possibility that the findings are biologically significant. Nevertheless, at the moment there are no validated risk biomarkers in breast epithelial lesions, and the morphologic diagnosis of atypical hyperplasia and lobular carcinoma in situ remains the strongest tissue-based marker of breast cancer risk.
moderate COX-2 expression, RR was 3.56 (95% CI, 1.94, 5.97) and with strong expression, RR was 5.66 (95% CI, 2.59, 10.75). Although these findings have not been replicated, the fact that COX-2 expression is also a marker of increased recurrence risk in DCIS lesions strengthens the possibility that the findings are biologically significant. Nevertheless, at the moment there are no validated risk biomarkers in breast epithelial lesions, and the morphologic diagnosis of atypical hyperplasia and lobular carcinoma in situ remains the strongest tissue-based marker of breast cancer risk.
An additional aspect of breast histology that has been investigated recently by Hartmann and colleagues in the Mayo Clinic cohort is the appearance of age-related lobular involution, described as physiological atrophy of the breast, with a decrease in the number and size of acini per lobule. These investigators hypothesized that absence of involution may be a risk factor for breast cancer, and classified benign biopsy sections into three categories: no involution where 0% lobules were involuted, partial involution with 1% to 74% lobules involuted, or complete involution with ≥75% lobules involuted. Involuted terminal duct lobular units (TDLUs) contain only a few to several small acini and flattened, inconspicuous acinar epithelium with fibrosis or fatty replacement of specialized intralobular stroma. They found a significant increase in relative risk in women displaying complete absence of involution. On further analysis, in a study that included mammographic density data, it appears that lobular involution is inversely associated with breast density (61% of women with extremely dense breasts displayed no involution); it remains independently associated with breast cancer risk following adjustment for mammographic density. Thus, the highest risk was observed in women with no involution and dense breasts (adjusted HR = 4.08, 95% CI = 1.72-9.68) (13). The mechanism of increased risk related to failure of lobular involution remains to be defined, but it is clearly plausible that involution is related to attrition of cytokine and hormonal signals that both maintain parenchymal volume, and support tumorigenesis in breast epithelium.
BREAST EPITHELIAL SAMPLING IN ASYMPTOMATIC, HIGH RISK WOMEN
Until recently, atypical hyperplasia has been identified only in women who developed breast lumps or mammographic findings that required biopsy. In such populations, the prevalence of atypical hyperplasia in an older series of open surgical biopsies was 3.5% (10) but more recent estimates in series of core needle biopsies of image-detected lesions range up to 9%. Autopsy studies suggest that the prevalence of occult atypical IEN could be as high 26%, depending on the detail of the sampling. There is good evidence, therefore, that the prevalence of occult breast IEN is significantly higher than that suggested by surgical biopsy data.
The recent interest in techniques for the sampling of epithelium from clinically normal breasts is motivated by the expectation that an improved ability to identify occult IEN would lead to improvements in our ability to assess individual risk. Noninvasive methods of breast epithelial sampling have another important application: the potential for the development of surrogate endpoints for the occurrence of malignancy, which are notably lacking in the field of breast cancer prevention at the moment. Although there has been significant progress in this area over the last decade or so, breast epithelial sampling of asymptomatic women remains a research tool, with no specific application in the clinical management of high risk women; available methods are briefly summarized below.
Methods of Non-Invasive, Minimal Sampling of the Healthy High Risk Breast
The techniques available include two, which obtain fluid and cells from ductal lumina (nipple aspiration fluid and ductal lavage) and two, which obtain epithelial and stromal cells via random fine or core needle biopsy.
Nipple Aspiration Fluid (NAF): Nipple fluid contains cells that are exfoliated into the ductal lumen and can be collected at the duct orifice by suction-aspiration. It is well tolerated, inexpensive, and produces samples that are paucicellular (or acellular) but rich in proteins, hormones, and possibly nucleic acids. It was first evaluated by Papanicolaou in the 1950s, with the goal of breast cancer detection, and was subsequently furthered by Sartorius, who developed a breast pump device designed to improve nipple fluid yield from asymptomatic women. The method in use today involves breast massage, dekeratinization of the nipple, and suctionaspiration with commercially available devices (Atossa Genetics, Halo Healthcare). Analyses of cells or protein in NAF samples obtained with these devices are also commercially available. However, the validation of protein assays is lacking, and reproducibility of cytologic analyses has long been a problem. A significant limitation of NAF as a biosample is the great variability in the fraction of women who yield of nipple fluid in various studies; a recent report from the Netherlands by van Diest and colleagues describes the successful use of oxytocin nasal spray to induce NAF production but the absence of a comparison group, or a within-person comparison before and after oxytocin use renders it difficult to interpret these results. Oxytocin nasal spray currently has no FDA approved uses in the United States, is difficult to obtain, and expensive. An added concern in the interpretation of studies of NAF-based biomarkers is the inability to extend these to women who do not yield NAF, and recent data suggest that non-yielders of NAF have significantly lower serum prolactin levels than yielders (Khan et al., manuscript under review).
Ductal Lavage (DL): DL is an extension of NAF, designed to overcome the problems of scant cellularity in NAF samples, and to sample the entire length of the ductal tree (14). The procedure involves application of a topical anesthetic or periareolar infiltration with lidocaine; the elicitation of nipple fluid as described above, cannulation of each fluidyielding duct (non-fluid yielding ducts can also be cannulated) with a single lumen catheter and lavage with normal saline. The lavage effluent is fixed, centrifuged to recover a cell pellet, and cytological smears prepared. Ductal lavage was introduced with the expectation that it would perform better than NAF in the identification of occult IEN. When compared to NAF, DL samples consistently provide a higher cell yield, but at significantly greater cost in supplies, time, and patient discomfort. Although some investigations continue, this technique has not proven useful for early diagnosis of breast cancer, risk evaluation, or for monitoring of biomarkers in prevention trials (15).
Random Fine-Needle Aspiration (rFNA): The rRNA method is performed in the clinically and radiologically normal breast in healthy, high risk women. It is based on the concept of field change in the high risk breast and does not provide site-specific sampling. First tested by Marshall in Utah in the late 1980s, major work in this area has subsequently been reported from University of Kansas by Fabian and colleagues, who have modified the original technique (16). After local anesthesia with buffered lidocaine with
epinephrine, eight to ten passes are made per breast with a 21 gauge needle introduced at two locations (periareolar, upper outer and upper inner quadrants). Samples from both breasts are pooled. With the prophylactic use of Vitamin K, use of buffered lidocaine, post-procedure cold packs, and tight-fitting sports bras, hematomas are rare, and the procedure is generally well tolerated.
epinephrine, eight to ten passes are made per breast with a 21 gauge needle introduced at two locations (periareolar, upper outer and upper inner quadrants). Samples from both breasts are pooled. With the prophylactic use of Vitamin K, use of buffered lidocaine, post-procedure cold packs, and tight-fitting sports bras, hematomas are rare, and the procedure is generally well tolerated.
Random Core Needle Biopsy (rCNB): With the availability of spring-loaded core needle biopsy devices, rCNB has become a possible approach to breast epithelial sampling. Some data exist in terms of the utility of this approach for tissue acquisition for biomarker studies (17), but there is no published information as to its use in risk assessment. In one biomarker study, up to seven cores were obtained from each subject, through the same skin incision. On average, 3 of the 6-7 cores per subject contained epithelium, the rest being fatty. The investigators were able to count 3,000 cells per case after Ki-67 labeling, by combining a mean of 11 corecut sections per subject. They did not find any significant difference in pre- and posttreatment Ki-67 labeling indices (17).
OCCULT IEN AND BREAST CANCER RISK
The concept of epithelial sampling to define breast cancer risk was pioneered by Petrakis and colleagues, who published a series of reports through the 1970s to the present, characterizing nipple fluid yield and cytologic findings in healthy women. In two cohorts studied by this group, NAF yield varied considerably (85% for the first cohort and 40% for the second) (18). In the first cohort, women who produced NAF and had cytological evidence of hyperplasia developed breast cancer at a 2.5-fold higher rate (95% CI, 1.1-5.5), which increased to 4.9-fold (95% CI, 1.7-13.9) when cytologic atypia was present (Fig. 19-3). Women who did not produce NAF were the reference group. In the second cohort, accrued between 1981 and 1991, the relative risk was 2.0 (95% CI, 1.3-3.3) for the hyperplasia and atypical hyperplasia groups combined, as no cancers occurred in the 22 women with atypical hyperplasia. There are no long-term follow-up studies of women who have undergone ductal lavage.
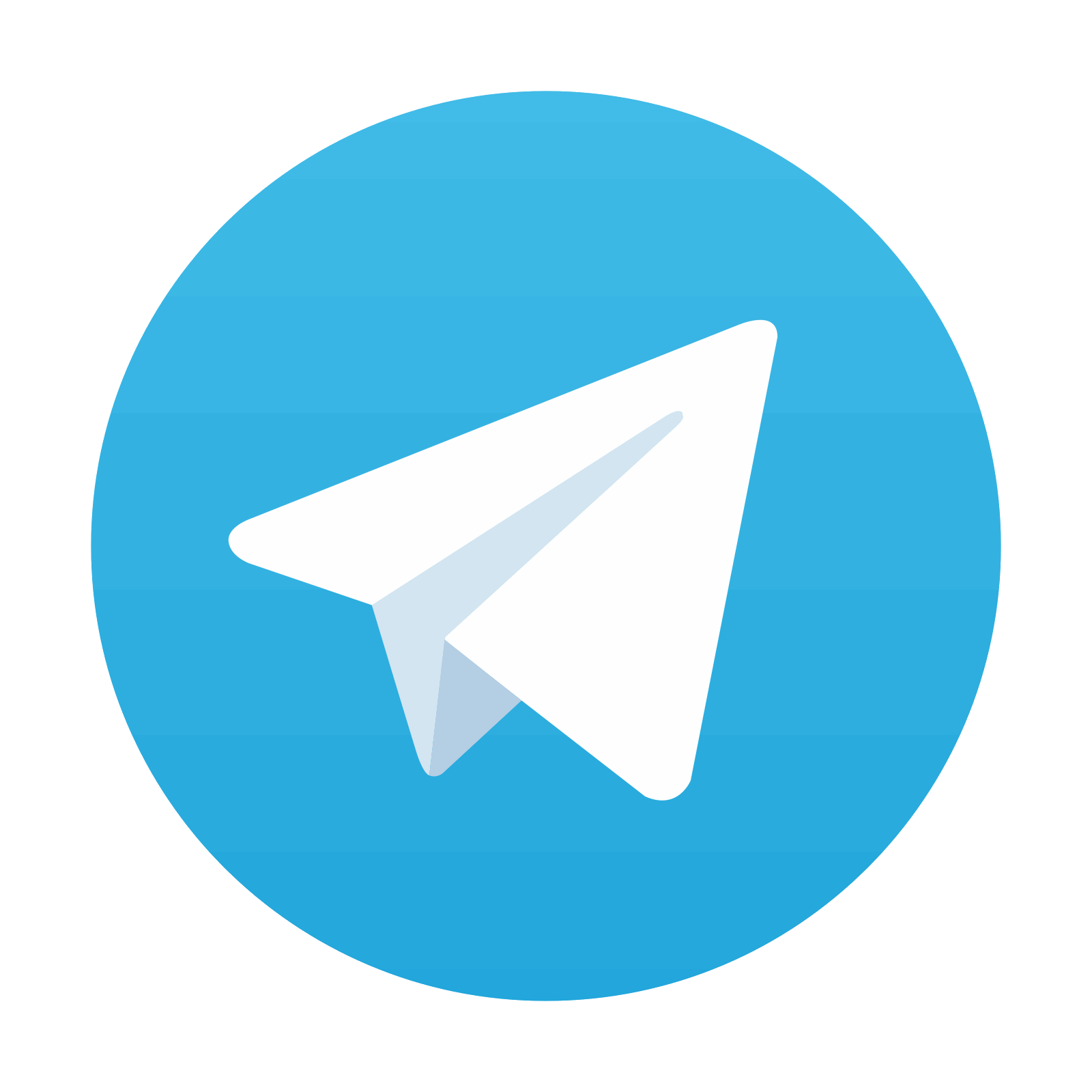
Stay updated, free articles. Join our Telegram channel

Full access? Get Clinical Tree
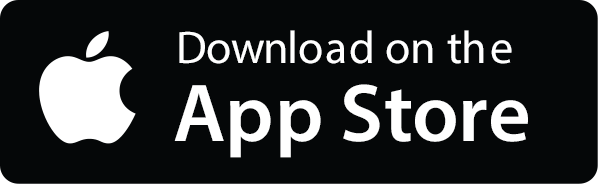
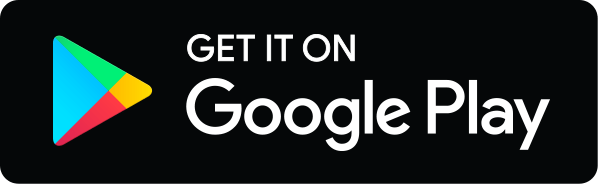