Lipoprotein (A): A Pleotropic Risk Factor for Cardiovascular Disease
Ernst J. Schaefer
Mary E. Herman
Jennifer J. Jenner
Leo J. Seman
In this chapter we review the current state of knowledge about the composition, genetics, functions, and metabolism of lipoprotein (a) or Lp(a). We also review the current status of the measurement of plasma or serum Lp(a), the relationship of Lp(a) elevation with atherosclerosis and cardiovascular disease (CVD), as well as treatment strategies to lower Lp(a).
Important milestones in research on Lp(a) include the following: its description in 1963 by Berg (1) as an antigenic variant of low-density lipoprotein (LDL) and its subsequent linkage with risk for CVD (2,3,4); characterization of Lp(a) lipoprotein and the development of a radioimmunoassay (5); the finding of apolipoprotein(a) (apo(a)) isoforms and their clear relationship with Lp(a) levels, underlining the importance of genetics in determining Lp(a) values (6,7); publication of a partial sequence for apo(a) (5); determination of the sequence of the apo(a) gene and its homology with plasminogen (8); documentation of the potential of Lp(a) to interfere in clot lysis activated by plasminogen (9); confirmation by many groups that elevated Lp(a) is an independent risk factor for CVD, a conclusion supported by an important meta-analysis (3); demonstration that Lp(a) can be lowered by niacin (10); the finding that Lp(a) serves as an acceptor of oxidized phospholipids in human plasma and that oxidized phospholipids can be reduced by treatment with a statin (11,12); the recent finding that inhibitors of cholesteryl ester transfer protein (CETP) can markedly lower Lp(a) (unpublished observation).
Some investigators believe that there is no residual risk associated with elevated Lp(a) if LDL cholesterol (LDL-C) levels are optimized to <100 mg/dL; however, recent data indicate that there is a continuous association of increasing risk with progressively higher levels of Lp(a) (13). In our view Lp(a) is a significant, independent, genetically determined risk factor, and when controlled clinical trials currently under way are completed in the year 2012, we predict that it will be clear that both the lowering of Lp(a) and the increasing of high-density lipoprotein cholesterol (HDL-C) levels with either niacin or a CETP inhibitor will significantly lower CVD risk. Firm guidelines for the treatment of elevated Lp(a) and low HDL-C await the completion of these studies.
It is clear that top tertile Lp(a) values (above 30 mg/dL or 74 nmol/L) are associated with a hazards ratio of about 1.6 for CVD as compared to bottom tertile values. In this regard elevated Lp(a) carries about the same risk as other common CVD risk factors including elevated LDL-C, decreased HDL-C, hypertension, and elevated C-reactive protein.
THEORETICAL CONSIDERATIONS
Composition of Lp(a)
When Lp(a) is exposed to reducing agents such as dithiolthreitol or β-mercaptoethanol, two subunits can be isolated: (1) an LDL particle containing apoB-100, and (2) apo(a), a carbohydrate-rich, highly hydrophilic protein, characterized by marked size heterogeneity due to genetic polymorphisms in its polypeptide. Lp(a) is thus an apoB-100-containing lipoprotein, with one molecule of apoB-100 attached to one molecule of apo(a) through a cysteine residue by a disulfide bond (14,15) (Fig. 18.1). As an apoB-100-containing lipoprotein, Lp(a) can be found in plasma at density <1.006 g/mL with very low density lipoproteins (VLDL), at density 1.006 to 1.0019 g/mL with intermediate-density lipoproteins (IDL), at density 1.019 to 1.063 g/mL with LDL, and also at density 1.063 to 1.125 g/mL with large HDL or HDL2. However, usually most of apo(a) in plasma is found in the density range of 1.050 to 1.100 g/mL and thus overlaps both the LDL and HDL2 density ranges. Lp(a) generally contains by weight about 40% protein, 35% cholesterol (mainly cholesteryl ester), 20% phospholipids, and 5% triglyceride.
The lipid composition of Lp(a) can vary based on the composition of the apoB-100-containing lipoprotein to which apo(a) is attached. Lp(a) in the VLDL, IDL, LDL, or HDL density range has prebeta mobility on lipoprotein electrophoresis, which distinguishes this lipoprotein from other apoB-100-containing lipoproteins which have beta mobility, except for VLDL (prebeta mobility). For this reason, Lp(a) was originally known as “sinking prebeta lipoprotein” because it is the only lipoprotein in the 1.006 g/mL infranant (or “bottom fraction” after ultracentrifugation) that has prebeta mobility (5) (see also Chapter 2).
Overall about one-third of Lp(a) is composed of cholesterol, mainly cholesteryl ester, found in the core of the particle. Therefore, elevated Lp(a) can contribute substantially to estimated LDL-C (see also Chapter 2). The division of total Lp(a) reported in mg/dL by 3 or in nmol/L by 7.4 provides a reasonable estimate of Lp(a) cholesterol. In our view Lp(a) may ultimately be measured by its cholesterol content as previously reported (16). One advantage of this approach is that cholesterol has been well standardized, and knowing the level of Lp(a) cholesterol allows one to subtract this value from LDL-C to calculate the true LDL-C level. This knowledge has important therapeutic implications because statins substantially lower LDL-C, without having a significant effect on Lp(a) values.
Genetics of Lp(a)
Multiple isoforms of apo(a) were first discovered by Utermann et al. (6,7), and low molecular forms of apo(a) were associated with elevated Lp(a) levels (6). The earliest classification of apo(a) isoforms denoted six apo(a) isoforms (F, B, S1, S2, S3, and S4), based on their apparent molecular weight on polyacrylamide gel electrophoresis (PAGE) ranging from approximately 400 to 700 kDa. Several isoforms had apparent molecular weights that were lower than that of apoB-100 (molecular weight 550 kDa). Subsequently, 11 isoforms were separated by PAGE, with molecular weights ranging from 419 to 838 kDa. Kamboh et al. (17) then found 23 apo(a) isoforms with further modifications of PAGE. Sandholzer et al. (18) examined apo(a) isoforms in seven different ethnic groups and documented that apo(a) isoform variation accounts for a substantial variation in plasma Lp(a) levels.
Homology of the Apo(a) and Plasminogen Genes
One of the most important breakthroughs in Lp(a) research occurred with the publication of a partial sequence for apo(a) (5). Those findings led to the sequencing of the apo(a) gene by the group at Genentech headed by Lawn et al., and the documentation of homology of apo(a) with plasminogen (8). These investigators documented that the genes encoding apo(a) and plasminogen are 50 kb apart on chromosome 6. The Genentech group also recognized that plasminogen had just one copy each of kringles I through V and that apo(a) consisted of kringles 4 and 5. However, there were multiple and variable number of kringle 4-like repeats (with 75% to 85% homology to kringle IV of plasminogen), but one copy of a kringle 5 (with 91% homology to kringle V of plasminogen) (Fig. 18.1). The name kringle is given to protein domains that have the shape of a Danish pastry. The 5′ untranslated region and the signal sequence region of the apo(a) gene and the plasminogen gene are 98% and 100% homologous, respectively (8). An unpaired cysteine residue at position 4,057 of apo(a) is necessary for the binding of apo(a) to apoB-100 through a disulfide bond (14) (Fig. 18.1). The apo(a) gene contains an albeit inactive protease domain with 94% homology to the protease domain of plasminogen. The apo(a) gene was derived from the plasminogen gene through a series of mutations, deletions, and recombinations (8). Lp(a) is found only in humans and Old World monkeys, indicating that it developed relative recently in mammalian evolution.
Dr. Utermann et al. in Innsbruck made a number of seminal contributions to the Lp(a) field. His group documented markedly elevated levels of Lp(a) in patients with renal failure, which could be reversed by kidney transplantation (19) (see also Chapter 11). They also noted that apo(a) isoform size determined Lp(a) levels both in normals as well as in patients with renal failure (19). The formation of Lp(a) was believed to be a complex process with apo(a) having multiple noncovalent interaction with apoB-100, followed by a covalent disulfide linkage of two free cysteine residues on both proteins. In the case of apoB-100, this residue always forms the surface of an apoB-100-containing lipoprotein, in either the VLDL, IDL, or LDL density region (20). These tenets were confirmed and extended by the mutagenic and physical-chemical studies of Becker and Koschinsky (21,22).
The Innsbruck group also examined the role of Lp(a) levels and isoforms in prediction of CVD, and concluded that the Lp(a) levels predicted the presence or absence of carotid atheroma, while apo(a) phenotypes emerged as one of the strongest predictors of the severity of carotid atherosclerosis (23). However, in an Asian Indian population, no relationship between apo(a) isoforms and CVD risk could be detected after controlling for Lp(a) levels (24). Dr. Utermann’s group also reported that a G for A substitution at the +1 donor splice site in the apo(a) kringle 4 type 8 intron occurs in about 6% of Caucasians, but not in Africans, and accounts for about 25% of subjects with undetectable plasma levels of Lp(a) (25). They also examined 14 single-nucleotide polymorphisms (SNPs) within exons, introns, and flanking regions of the portion of the apo(a) gene coding for apo(a) kringles 4, types 6, 8, 9, and 10, and reported in an African population that the S37F substitution in kringle 4 type 6 and the G17R substitution in kringle 4 type 8 resulted in significantly lower Lp(a) levels, while the R18W substitution in kringle 4 type 9 was associated with significant increases in Lp(a) levels (26). Dr. Utermann’s group reported that a nonsense mutation at this site found in 2% of the population causes the formation of a truncated form of apo(a) kringle 4 type 2 and lower Lp(a) levels (27). These investigators also reported that the apo(a) gene may be a susceptible gene for type I diabetes (23).
Utermann’s group also documented that lysine-binding sites in kringle 4 type 10 are the major binding sites for fibrinogen (28) and that developmental arterial and neural crest growth factor (or DANCE) directly interacts and binds to apo(a) (29) (Fig. 18.2). In an Asian Indian population, these investigators could only detect a relationship between Lp(a) levels but not apo(a) isoforms with CVD risk (24). After assay standardization for Lp(a) in a large population of South African families with familial hypercholesterolemia (FH), mutations at the LDL receptor locus causing FH clearly affected Lp(a) levels in a gene-dosage manner, with homozygotes having the highest levels (30). Examining Lp(a) genetics in an African population in Gabon, Dr. Utermann’s group reported that Lp(a) levels, even in this homogeneous population, are highly heritable, due mainly to variation at the apo(a) gene locus on chromosome 6 (31).
Major contributions were also made in Lp(a) research by Hobbs et al. Using pulsed field electrophoresis, this group documented for the first time in 1991 that there were 34 different isoforms of kringle 4 of apo(a), due to 12 to 51 repeats of kringle 4-encoding sequences (32). These investigators also found high levels of apo(a) fragments in the plasma of endstage renal disease patients (33). However, they showed that there were increases in both full-length apo(a) as well as apo(a) fragments in plasma (33). In LDL receptor-negative mice expressing human apoB-100, aortic atherosclerosis was not accentuated by the expression of human apo(a) (34). In contrast, in a more physiologic model of overexpression of apo(a) in transgenic Watanabe rabbits, atherosclerosis increased significantly (35). In contrast to other studies on CVD risk, Hobbs’ group could not detect any significant relationship between Lp(a) levels, apo(a) isoforms, and coronary artery calcification in the Dallas Heart Study in either blacks or whites (36).
Boerwinkle et al. (37) documented that 69% of the variation in Lp(a) levels is due to variation in the number of kringle 4 repeats, with an additional 22% being due to variation in gene expression. They estimated that 91% of the variation in Lp(a) levels is due to variation at the apo(a) gene locus.
Ober et al. (38) recently reported that there are 77 SNPs spanning 12.4 megabases on chromosome 6q26-q27, involving nine genes in this region including the LPA gene. The investigators documented that variation in six genes in this area including LPA was associated with Lp(a) levels independent of each other and of the kringle 4 repeat polymorphism in the LPA
gene. Their conclusion was that there exists “a complex genetic architecture of Lp(a) levels that may involve multiple loci on chromosome 6q26-q27.” A novel SNP in intron 37 of LPA was associated with Lp(a) levels and CVD risk (38).
gene. Their conclusion was that there exists “a complex genetic architecture of Lp(a) levels that may involve multiple loci on chromosome 6q26-q27.” A novel SNP in intron 37 of LPA was associated with Lp(a) levels and CVD risk (38).
Functions of Lp(a)
Pleiotropic Effects of Lp(a)
Interference of Lp(a) with the Function of Plasminogen. Plasminogen is a zymogen that when activated to plasmin potentiates the dissolution of fibrin clots (39,40,41). Mice transgenic for human apo(a) are resistant to tissue-plasminogen activator-mediated thrombolysis (9). These data indicate that Lp(a) interferes with the function of plasminogen. Plasminogen itself is a 93-kDa glycoprotein which has one copy of each of five kringle types (kringles I-V) and a protease domain (39,40,41). Kringles are trilooped structures of approximately 80 amino acids in length and are stabilized by three cysteine bonds. Their name is derived from their similarity in shape to that of a Danish pastry (39,40,41). The kringles are present in several of the factors involved in blood coagulation and clot lysis. Their function is thought to modulated by their binding to various substrates through lysine residues, or “lysine-binding sites (LBS)” (39,40,41). Kringle type I of plasminogen has the highest lysine affinity, while kringle type IV has only moderate affinity. Plasminogen and apo(a) form a complex by which apo(a) can interfere with the action of plasminogen (41). Feric et al. (42) reported that apo(a) inhibits the conversion of Glu-plasminogen to Lys-plasminogen, resulting in an inhibition of its action to break up clots. These data suggest that elevated Lp(a) levels may interfere with thrombolysis in humans and serve as a potent risk factor for atherosclerosis and CVD (43).
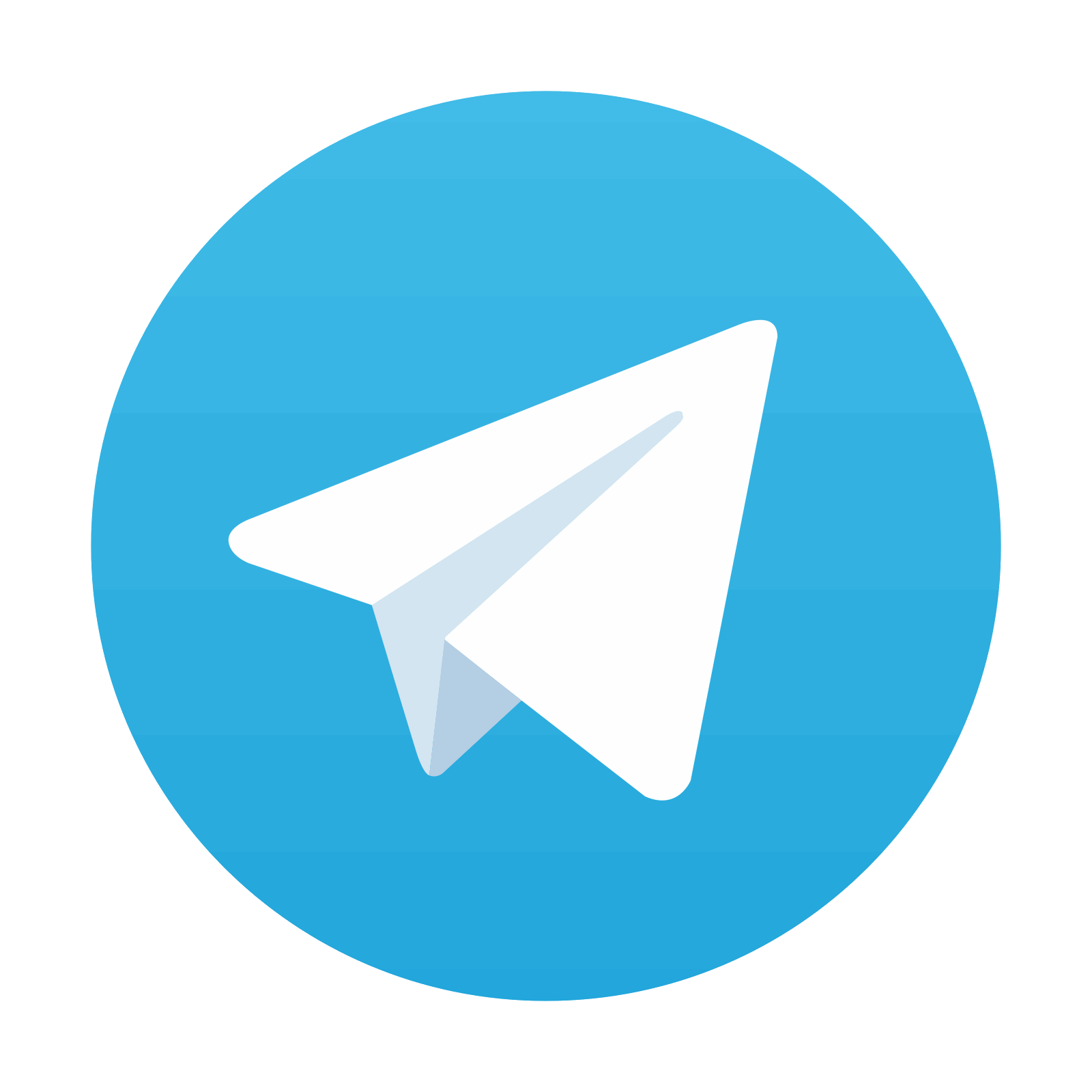
Stay updated, free articles. Join our Telegram channel

Full access? Get Clinical Tree
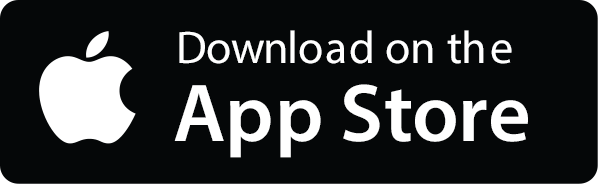
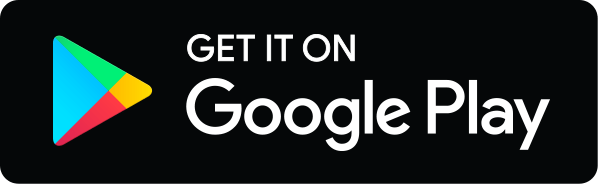
