LABORATORY EVALUATION
TESTOSTERONE
Physiologic Aspects of Testosterone Testing.
Testosterone is both a paracrine regulator of spermatogenesis (see Chap. 113) and a hemocrine hormone. The testicular content of testosterone is ˜50 μg (1 μg/g testis), whereas the blood production rate is ˜5000 μg per day, indicating that only a small portion of the testosterone produced each day is stored in the testes. The testosterone precursor steroids, including pregnenolone, 17-OH pregnenolone, dehydroepiandrosterone (DHEA), progesterone, 17α-hydroxyprogesterone, and
androstenedione, are also secreted by the testis, and the relative concentrations of these steroids in spermatic vein blood are proportional to their testicular concentrations.6 The release of precursor steroids into the circulation may indicate that they are unnecessary by-products in the orderly biotransformation of pregnenolone to testosterone, because none is known to have a physiologic action in the male. Androstenedione is of special interest because it is used as a performance-enhancing drug. Androstenedione is bioconverted to testosterone and to estrone, but there is little published information on the endocrine profile following androstenedione administration.
androstenedione, are also secreted by the testis, and the relative concentrations of these steroids in spermatic vein blood are proportional to their testicular concentrations.6 The release of precursor steroids into the circulation may indicate that they are unnecessary by-products in the orderly biotransformation of pregnenolone to testosterone, because none is known to have a physiologic action in the male. Androstenedione is of special interest because it is used as a performance-enhancing drug. Androstenedione is bioconverted to testosterone and to estrone, but there is little published information on the endocrine profile following androstenedione administration.
A single sample of blood, generally drawn in the morning, can be used to measure testosterone. The usual normal range in morning samples is 3 to 10 ng/mL (10–40 nmol/L). There is a diurnal variation in testosterone in adult men, with highest levels in the early morning, followed by a progressive fall throughout the day, reaching the lowest levels in the evening and during the first few hours of sleep. Peak and nadir values differ by ˜15%, although more pronounced differences are sometimes observed.7 The diurnal rhythm is blunted with aging8 and in men with testicular failure.9 The metabolic clearance of testosterone is not thought to vary throughout the day, so that the diurnal testosterone rhythm is presumed to result from a day-night difference in testosterone production. Because there are no parallel changes in serum LH levels, however, the origin as well as physiologic significance of the diurnal testosterone rhythm remains uncertain. It is important to measure testosterone in the morning, because reference ranges are based on morning values. Frequent sampling of spermatic venous blood reveals testosterone secretory pulses at a frequency of ˜1 pulse per hour,6 but because of this rapid frequency and relatively low pulse amplitude only small fluctuations occur in peripheral plasma. Testosterone secretory bursts are more readily defined in the peripheral blood when pulse frequency is low.7 There is a good correlation between the plasma testosterone level at first sampling and the mean of multiple samples taken over 1 year, so that one morning sample is reasonably representative,10 although abnormal and borderline values should be confirmed.
There is a prominent sleep-related increase in serum testosterone in pubertal boys, with abrupt rises from female to adult male levels.11 This difference can be used clinically to evaluate boys with delayed puberty (see Chap. 92), because the rise to a higher testosterone value in the morning may precede pubertal testis growth and indicates that puberty has begun.12 Men with hypogonadism and hyperprolactinemia have an exaggerated diurnal testosterone rhythm, leading to very low levels in the afternoon and evening, which can explain clinical hypogonadism despite a normal morning total testosterone level,9 as sometimes occurs in men with a prolactinoma. The testosterone level tends to rise during intense exercise because of hemoconcentration13 and to decline 12 to 24 hours later because gonadotropin-releasing hormone (GnRH) secretion is reduced.14 Testosterone levels are also reduced in acute and chronic illness15 and with fasting for at least 48 hours. These factors can confound an evaluation of testicular function.
Testosterone Metabolism.
The metabolism of testosterone is shown in Figure 114-1. Testosterone is metabolized into two biologically important products, dihydrotestosterone (DHT) and estradiol, by the enzymes 5α-reductase and aromatase, respectively. Most of the metabolism of testosterone occurs in the liver, however, via 3α- and 3β-hydroxysteroid dehydrogenase, 5α- and 5β-steroid reductase, and oxidation of the D-ring to the 17-keto-steroids androsterone (3α-hydroxy-5α-androstane-17-one) and etiocholanolone (3α-hydroxy-5β-androstane-17-one), which are then excreted in the urine. Most of the 10 to 25 mg per day of keto-steroids in the urine of men is of adrenal origin, however, so that the urinary 17-ketosteroid excretion is not a test of testicular function. Testosterone metabolites are also conjugated to sulfuric and glucuronic acids at the 3- or 17-position and excreted in the urine and bile. A small fraction (2%) of the circulating testosterone is excreted unchanged in the urine.
SEX HORMONE–BINDING GLOBULIN
Of the circulating testosterone in normal men, <4% is free (not protein bound), 1% to 2% is bound to cortisol-binding globulin, ˜50% is loosely bound to albumin, and ˜45% is bound with high affinity to sex hormone–binding globulin (SHBG), a β-globulin produced by the liver16 (Fig. 114-2). (SHBG is also referred to as testosterone-binding globulin [TeBG] or sex steroid–binding protein [SBP]). The level of SHBG in plasma is a strong predictor of the total testosterone level in normal men (Fig. 114-3), accounting for 50% of the variance in total testosterone. SHBG is present in plasma as a 100-kDa heterodimer of variably glycosylated subunits.17,18 Androgen-binding protein is a nearly identical protein that is produced by Sertoli cells of the testis.19 Androgen-binding protein and SHBG differ in carbohydrate content. Although SHBG binds testosterone and other steroids and prolongs their metabolic clearance, its function remains
controversial. The finding of membrane-binding sites for androgen-binding protein in the epididymis and for SHBG in testis, prostate, and other tissues and the activation of the cyclic adenosine monophosphate (cAMP)/protein kinase A (PKA) pathway, which is a coactivator of androgen receptors (ARs), suggest that these binding proteins could play a direct role in androgen action. However, according to the free hormone hypothesis, which states that only free steroid enters target cells, SHBG functions solely as a reservoir for testosterone.
controversial. The finding of membrane-binding sites for androgen-binding protein in the epididymis and for SHBG in testis, prostate, and other tissues and the activation of the cyclic adenosine monophosphate (cAMP)/protein kinase A (PKA) pathway, which is a coactivator of androgen receptors (ARs), suggest that these binding proteins could play a direct role in androgen action. However, according to the free hormone hypothesis, which states that only free steroid enters target cells, SHBG functions solely as a reservoir for testosterone.
FREE OR BIOAVAILABLE TESTOSTERONE
For most clinical applications the measurement of the total testosterone level is entirely satisfactory. However, when circulating SHBG levels are altered (Table 114-2), this change may be reflected as an increase or a decrease in the measured serum total testosterone concentration. For example, a low testosterone level in an obese man may be misinterpreted to suggest androgen deficiency (see Fig. 114-2). Because there is considerable evidence that the non–SHBG-bound portion of circulating testosterone represents the biologically active fraction, many methods for determining non–SHBG-bound or free testosterone have been developed.20
METHODOLOGY
Total Testosterone.
Total testosterone can be measured directly in serum by immunoassay, or after extraction with organic solvents with or without further chromatographic separation. Commercial kits for the direct assay of testosterone in unextracted serum or plasma using an iodine-125 (125I)-labeled tracer are technically easy to use, precise, and sufficiently accurate for most purposes. When SHBG levels are low, however (see later), the result for testosterone may be overestimated, whereas high SHBG levels may lead to underestimation of the actual testosterone value. This artifact appears to result from differences between the SHBG levels in the assay standards and samples, with nonlabeled testosterone binding to both SHBG and to the testosterone antiserum, whereas the 125I-testosterone tracer binds primarily to the antiserum.21 Direct assays also tend to overestimate the true values of testosterone at low concentrations.
To discriminate among low levels more accurately, or to control for the effects of abnormal SHBG concentrations, immunoassays using organic solvent extraction and chromatographic separation may be needed, but are more costly and difficult to perform.
Fully automated immunoassay analyzers have been introduced into clinical laboratories for competitive and two-site assays.22 These electrochemiluminescence immunoassays (ECLIAs) use nonradiolabeled detection methods, and have very short incubation times. Thus, they are attractive for clinical laboratories. The precision and accuracy are quite acceptable for samples from adult men, with results similar to those of radioimmunoassays (RIAs). At low levels, however, ECLIAs may produce values that are 50% to 100% higher than those of RIAs. Lipemia may also produce inaccurate high values.
Equilibrium Dialysis Assay.
The equilibrium dialysis assay is used to calculate the percent free testosterone. The diffusion across a semipermeable membrane of tracer amounts of 3H-testosterone added to the sample is measured. The free testosterone concentration is then calculated from the product of the total testosterone level and the percentage of tracer crossing the dialysis membrane (percent free testosterone). The latter is usually 1% to 4%, with a free testosterone level commonly ranging from 4 to 20 ng/dL. This assay is complex, with potential errors due to temperature, sample dilution, and tracer impurities. (Testosterone can also be measured by RIA in the dialysate, avoiding the use of 3H-testosterone, but a very sensitive immunoassay is needed.) Centrifugal ultrafiltration is a variation of equilibrium
dialysis in which high-speed centrifugation is used to separate bound from free hormone across a dialysis membrane.
dialysis in which high-speed centrifugation is used to separate bound from free hormone across a dialysis membrane.
Calculated Free Testosterone.
Another approach for correcting the total testosterone value for variations in SHBG concentrations is to calculate the free testosterone level from the levels of total testosterone and SHBG, using binding constants for SHBG and albumin.23 This value appears to correlate quite well with the value obtained by equilibrium dialysis.
Free Testosterone Index.
The “free testosterone index” has been calculated as the ratio: total testosterone/SHBG using units of nmol/L. Although the calculation is easy to perform and is believed to be valid in women, it appears to be less useful in men, because most of the SHBG in men is bound to testosterone.24
Analog Kits.
The free testosterone level has been determined directly using solid-phase free testosterone RIA analog kits, which use an 125I-labeled testosterone analog as the tracer. This assay is based on the selective binding of the analog tracer to the testosterone antiserum, but not to SHBG, and is a popular, high-precision, single-step, nonextraction method. Although there is a strong positive correlation between free testosterone levels measured by these analog kits and by equilibrium dialysis assay, the kits produce substantially (75%) lower values.24a Moreover, the level of SHBG is a positive predictor of the free testosterone level as measured by analog methods. The percentage of free testosterone (determined by the analog method) does not decrease as SHBG increases, and this free testosterone level is almost perfectly positively correlated with the total testosterone. Thus, both the total testosterone and the free testosterone (determined by the analog method) appear to provide essentially the same clinical information.25
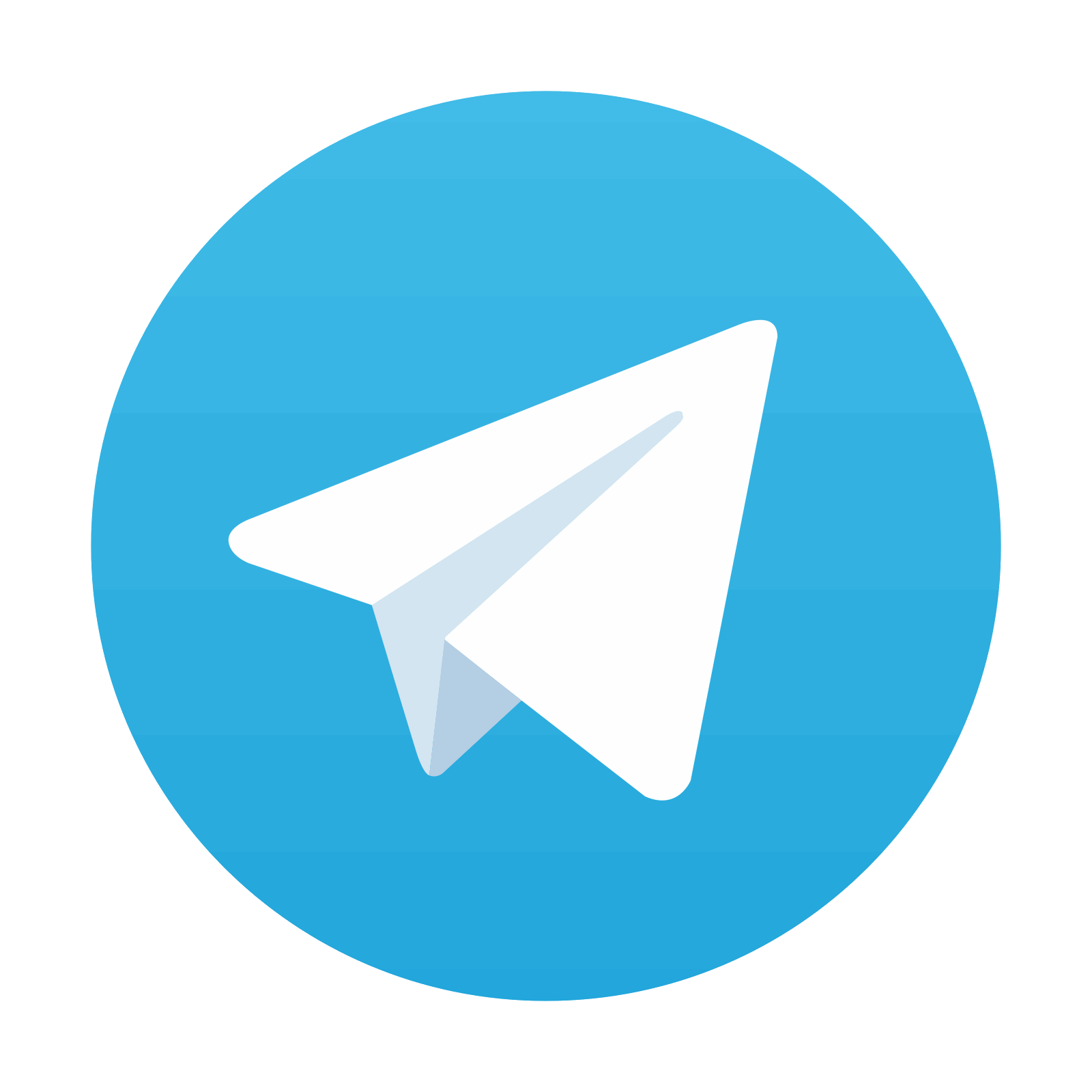
Stay updated, free articles. Join our Telegram channel

Full access? Get Clinical Tree
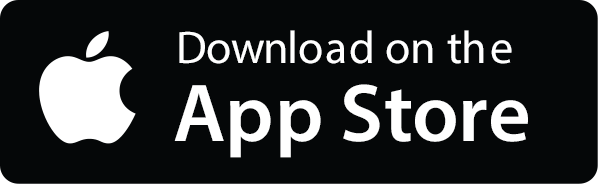
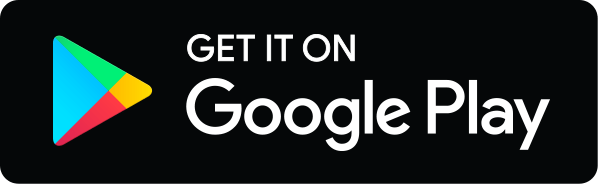
