Kidney Cancer
W. Marston Linehan
Laura S. Schmidt
Kidney cancer or renal cell carcinoma (RCC) affects more than 209,000 people annually worldwide, resulting in 102,000 deaths each year.1 A variety of risk factors, including obesity, hypertension, tobacco smoking, and certain occupational exposures, have been shown to increase one’s risk for developing RCC. Our current understanding of the molecular genetics of kidney cancer has come from studies of families with an inherited predisposition to develop renal tumors. Individuals with a family history of RCC have a 2.5-fold greater chance for developing renal cancer during their lifetimes2 and comprise about 4% of all RCC.
Kidney cancer is not a single disease but is classified into tumor subtypes based on histology.3 Over the past two decades, studies of families with inherited renal carcinoma enabled the identification of five inherited renal cancer syndromes, and their predisposing genes (Table 20.1), which implicate diverse biological pathways in renal cancer tumorigenesis.4 The von Hippel-Lindau (VHL) tumor suppressor gene was discovered in 1993.5 Subsequently, activating mutations were identified in the MET protooncogene in patients with hereditary papillary renal carcinoma (HPRC).6 More recently, the gene for Krebs cycle enzyme fumarate hydratase (FH), responsible for hereditary leiomyomatosis and renal cell carcinoma (HLRCC),7 and FLCN, the gene for Birt-Hogg-Dubé (BHD) syndrome, were identified.8 Germline mutations in the gene encoding another Krebs cycle enzyme, succinate dehydrogenase subunit B (SDHB), have been found in patients with familial renal cancer.9 Discovery of the genes for the inherited forms of renal cancer has enabled the development of diagnostic genetic tests for presymptomatic diagnosis and improved prognosis for at-risk individuals.
VON HIPPEL-LINDAU
VHL is an autosomal dominantly inherited multisystem neoplastic disorder that is characterized by clear cell renal tumors, retinal angiomas, central nervous system hemangioblastomas, tumors of the adrenal gland (pheochromocytoma), endolymphatic sac and pancreatic islet cell, and cysts in the pancreas and kidney. VHL occurs in about 1 in 36,000 and develops during the second to fourth decades of life with nearly 70% penetrance by age 60. Bilateral, multifocal renal tumors with clear cell histology develop in 25% to 45% of VHL patients10 that can have metastatic potential when they reach 3.0 cm.
Genetics of Von Hippel-Lindau
Loss of heterozygosity (LOH) on chromosome 3p in clear cell renal tumors suggested the location of a predisposing gene for RCC.11 Positional cloning in VHL kindreds defined the disease locus to chromosome 3p25-26, leading to the cloning of the VHL gene in 1993.5 VHL is a tumor suppressor gene in which both copies of VHL must be inactivated for tumor initiation. Germline VHL mutations that predispose to VHL encompass the entire mutation spectrum, including large deletions, protein-truncating mutations, and missense mutations that exchange the amino acid in the VHL protein. Over 1,000 different VHL mutations have been identified in more than 945 VHL families worldwide. Mutations are located throughout the entire gene with the exception of the first 35 residues in the acidic domain.10 With the development of new methods for detection of deletions, VHL mutation detection rates are approaching 100%.12,13 VHL subclasses based on the predisposition to develop pheochromocytomas, and high or low risk of RCC are established with interesting genotype-phenotype associations.14
Gene Mutated in Renal Cancer Families with Chromosome 3p Translocations
In 1979 Cohen et al.15 described a family with a constitutional t(3;8)(p14;q24) balanced translocation that cosegregated with bilateral multifocal clear cell renal tumors. Loss of the derivative chromosome carrying the 3p segment and different
somatic mutations in the remaining copy of VHL were identified in the tumors from this translocation family. Based on these data Schmidt et al.16 proposed a three-step tumorigenesis model in 3p translocation families: (1) inheritance of the constitutional translocation, (2) loss of the derivative chromosome bearing 3p25, and (3) mutation of the remaining copy of VHL, resulting in inactivation of both copies of VHL and predisposing to clear cell RCC. A number of chromosome 3 translocation families have been described.17,18 Loss of the derivative chromosome concomitant with somatic mutation of the remaining copy of VHL in these families provides strong evidence for the three-step tumorigenesis model and implicates VHL loss in clear cell RCC that develops in chromosome 3 translocation kindreds.
somatic mutations in the remaining copy of VHL were identified in the tumors from this translocation family. Based on these data Schmidt et al.16 proposed a three-step tumorigenesis model in 3p translocation families: (1) inheritance of the constitutional translocation, (2) loss of the derivative chromosome bearing 3p25, and (3) mutation of the remaining copy of VHL, resulting in inactivation of both copies of VHL and predisposing to clear cell RCC. A number of chromosome 3 translocation families have been described.17,18 Loss of the derivative chromosome concomitant with somatic mutation of the remaining copy of VHL in these families provides strong evidence for the three-step tumorigenesis model and implicates VHL loss in clear cell RCC that develops in chromosome 3 translocation kindreds.
TABLE 20.1 HEREDITARY RENAL CANCER SYNDROMES | ||||||||||||||||||||||||||||||||||||||||||
---|---|---|---|---|---|---|---|---|---|---|---|---|---|---|---|---|---|---|---|---|---|---|---|---|---|---|---|---|---|---|---|---|---|---|---|---|---|---|---|---|---|---|
|
Gene for Clear Cell Kidney Cancer
Mutation of the VHL gene is found in a high percentage of tumors from patients with clear cell kidney cancer.19 Nickerson et al.20 recently identified mutation or methylation of the VHL gene in 92% of clear cell kidney cancers. VHL gene mutation is not found in papillary, chromophobe, collecting duct, medullary, or other types of kidney cancer.
Function of the VHL Protein
The most well-understood function of the VHL protein pVHL is the substrate recognition site for the hypoxia-inducible factor (HIF)-α family of transcription factors targeting them for ubiquitin-mediated proteasomal degradation (Fig. 20.1).14 pVHL binds through its α domain to elongin C and forms an E3 ubiquitin ligase complex with elongin B, cullin-2, and Rbx-1. Under normal oxygen conditions, HIF-α becomes hydroxylated on critical prolines by a family of HIF prolyl hydroxylases (PHD) that require 2-oxoglutarate, molecular oxygen, ascorbic acid, and iron as cofactors. pVHL then binds to hydroxylated HIF-α through its β domain, targeting HIF-α for ubiquitylation by the E3 ligase complex. Under hypoxic conditions when PHDs are unable to function or when pVHL is mutated, altering its binding to HIF-α or elongin C binding, HIF-α cannot be recognized by pVHL. HIF-α accumulates and transcriptionally upregulates a number of genes important in blood vessel development (EPO, VEGF), cell proliferation (PDGFβ, TGFα), and glucose metabolism (GLUT-1).14 HIF-α dependent up-regulation of target genes involved in neovascularization provides an explanation for the increased vascularity of central nervous system (CNS) hemangioblastomas and clear cell renal tumors in VHL. Germline VHL mutations frequently occur in the pVHL binding domains for HIF-α and elongin C.21 HIF-2α, rather than HIF-1α, stabilization appears to be critical for renal tumor development.22,23 Additional HIF-dependent and HIF-independent functions for pVHL have been reported.10,14
![]() FIGURE 20.1 The von Hippel-Lindau (VHL) E3 ubiquitin ligase complex targets hypoxia-inducible factor (HIF)-α for ubiquitin-mediated degradation. A: Under normal oxygen conditions, HIF-α is hydroxylated on critical prolines by HIF prolyl hydroxylase (PHD), requiring molecular oxygen, 2-oxoglutarate, and iron as cosubstrates. The VHL protein (pVHL) can then recognize and bind hydroxylated HIF-α, enabling its ubiquitylation by the VHL E3 ligase complex and degradation by the proteasome. Under hypoxic conditions, PHD is unable to function properly, pVHL cannot recognize HIF-α, and HIF-α accumulates, leading to up-regulation of HIF-target genes (VEGF, GLUT1, PDGF) that support tumor growth and neovascularization. B: When VHL is mutated and pVHL is unable to bind HIF-α, HIF-α stabilization leads to transcriptional up-regulation of HIF target genes. (From ref. 4, with permission.) |
HEREDITARY PAPILLARY RENAL CARCINOMA TYPE 1
HPRC is an autosomal dominant hereditary cancer syndrome in which affected individuals are at risk for the development of multifocal, bilateral papillary type 1 kidney cancer.24 HPRC develops in the fifth and sixth decades with age-dependent penetrance estimated at 67% by 60 years of age25; however, early onset HPRC has been described.26 This rare disorder has been reported in less than 40 kindreds worldwide.24
Genetics of Hereditary Papillary Renal Carcinoma: MET Protooncogene
In 1995 Zbar et al.27 described ten families in which multifocal, bilateral papillary renal tumors were inherited in an autosomal dominant fashion and suggested that these families might represent a hereditary counterpart to sporadic papillary tumors. Schmidt et al.6 localized the HPRC disease locus to chromosome 7q31.1-34 by linkage analysis. Since trisomy of chromosome 7 was described as a hallmark feature of papillary renal tumors,28 a gain-of-function oncogene seemed a likely candidate disease gene; in fact, germline missense mutations were identified in the tyrosine kinase domain of the MET protooncogene located at 7q31 in affected HPRC family members.6 Mutations of the MET gene have been detected in 13% of sporadic papillary renal tumors.6,29 Further studies to determine the role of MET and related genes in papillary type 1 kidney cancer are currently under way.
Hereditary Papillary Renal Carcinoma: Functional Consequences of MET Mutations
The MET protooncogene encodes the hepatocyte growth factor/scatter factor (HGF/SF) receptor tyrosine kinase. Binding of ligand HGF to MET triggers autophosphorylation of critical tyrosines in the intracellular tyrosine kinase domain, subsequent phosphorylation of tyrosines in the multifunctional docking site, and recruitment of a variety of transducers of downstream signaling cascades that regulate cellular programs, leading to cell growth, branching morphogenesis, differentiation, and “invasive growth.”30 Although MET overexpression has been demonstrated in a number of epithelial cancers,31 HPRC was the first cancer syndrome for which germline MET mutations were identified. The missense MET mutations in HPRC are constitutively activating without ligand stimulation, display oncogenic potential in vitro,32,33 and are predicted by molecular modeling to stabilize active MET kinase.34 Nonrandom duplication of the chromosome 7 bearing the mutant MET allele was demonstrated in papillary renal tumors from HPRC patients35 and may represent the second step in HPRC tumor pathogenesis. The presence of two copies of mutant MET may give kidney cells a proliferative growth advantage and lead to tumor progression.
XP11.2 TRANSLOCATION RENAL CELL CANCER
Xp11.2 translocation renal cell carcinomas, typically presenting with papillary architecture and clear or eosinophilic cytoplasm, are uncommon tumors often detected in young children and adolescents. Translocations involving Xp11.2 and 1q 21.2 associated with sporadic papillary renal carcinoma, and first described in a 2-year-old child,36 generate a fusion between a novel gene, PRCC, and the basic helix-loop-helix family transcription factor gene, TFE3.37 The encoded fusion protein, PRCC-TFE3, acts as a stronger transcriptional activator than native TFE3, and loss of the majority of native TFE3 transcripts is observed in these tumors. This deregulation of normal TFE3 transcriptional control caused by the chromosomal translocation may be important to the development of sporadic papillary renal cell carcinoma.38
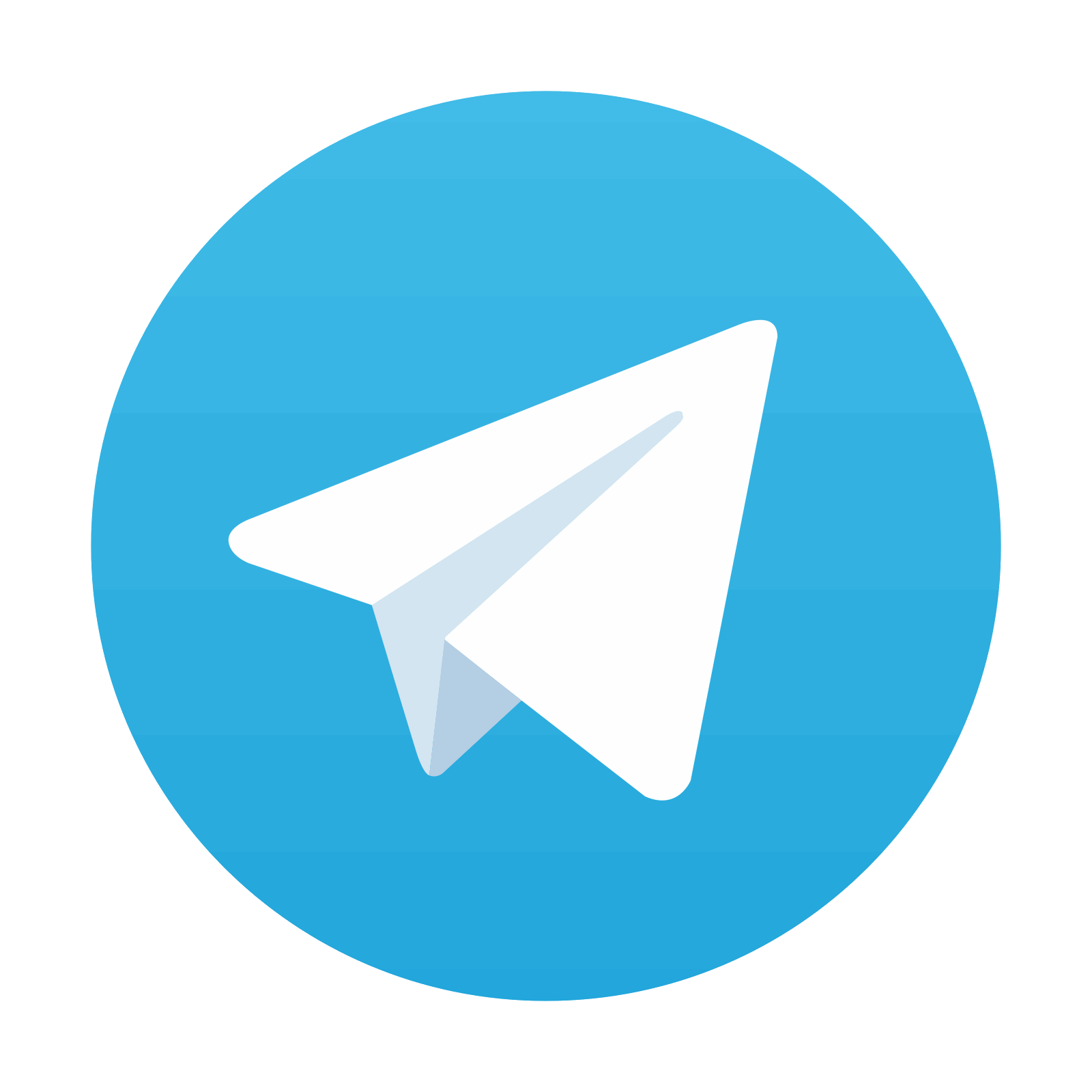
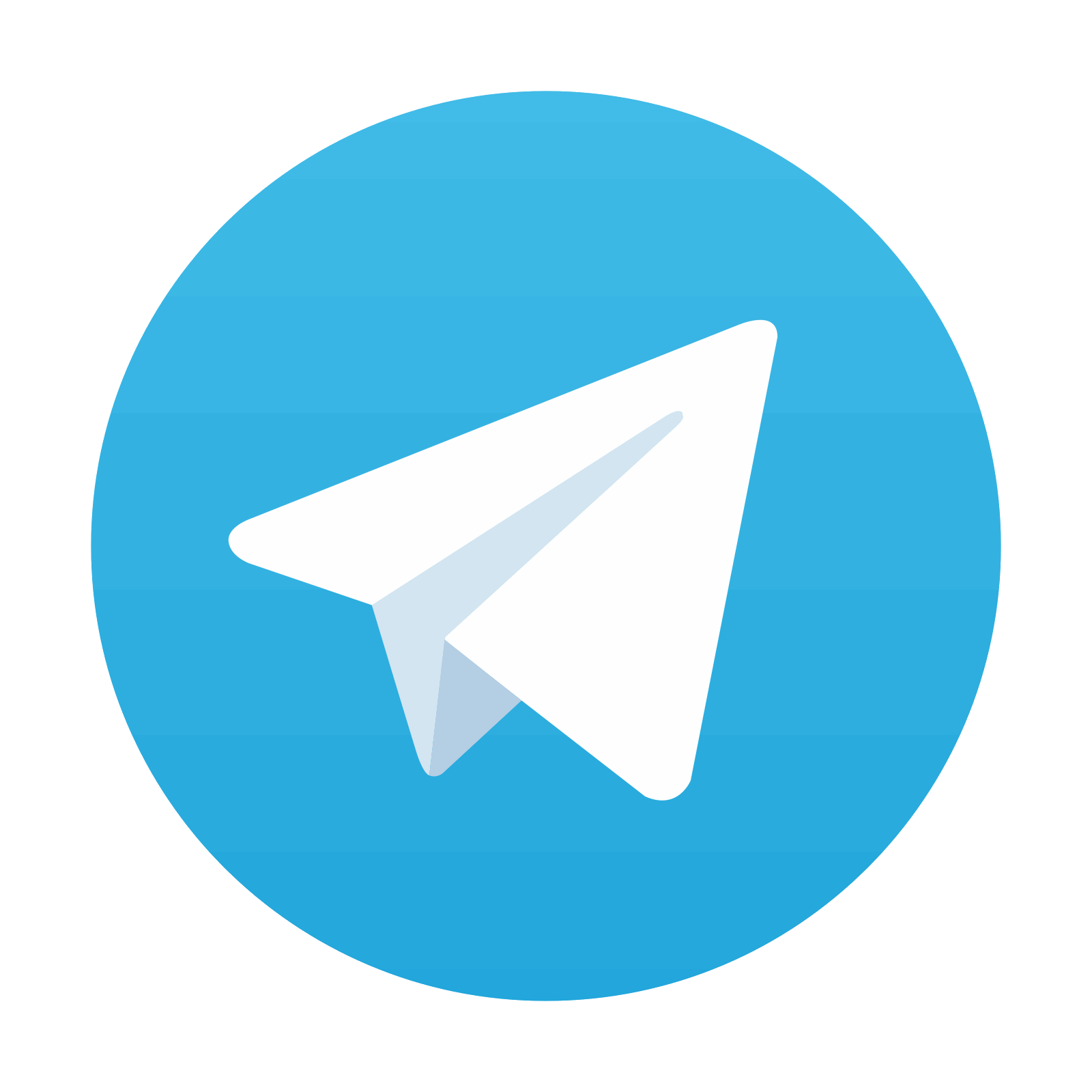
Stay updated, free articles. Join our Telegram channel

Full access? Get Clinical Tree
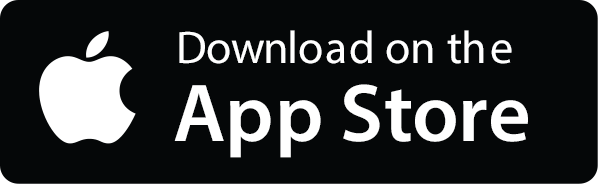
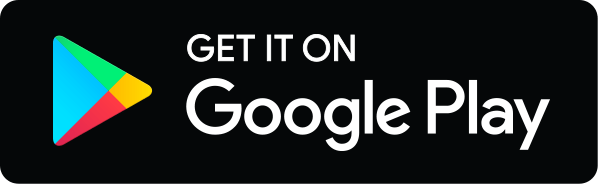