38730 Interventional Radiology Multidisciplinary management at high-volume sarcoma centers leads to improved short- and long-term outcomes in sarcoma patients when compared to treatment at low-volume centers. Multidisciplinary management involves experienced teams consisting of oncologists, surgeons, radiation oncologists, pathologists, and interventional radiologists with the goal of treatment being long-term survival while minimizing morbidity and maximizing function. Sarcoma management requires participation by the interventional radiology team to provide adequate biopsies and appropriate venous access to allow for chemotherapy delivery. Moving beyond these established roles offers unique opportunities for local control and treatment. This chapter presents the role of the interventional radiologist in the multidisciplinary team for the management of sarcoma patients. Interventional Radiology, embolization, biopsy, ablation, irreversible electroporation chemotherapy, interventional radiology, morbidity, patients, sarcoma management Disease Management, Drug Therapy, Morbidity, Patients, Radiology, Interventional, Sarcoma INTRODUCTION Multidisciplinary management at high-volume sarcoma centers lead to improved short and long-term outcomes in these patients when compared to treatment at low-volume centers.1,2 Multidisciplinary management involves experienced teams consisting of oncologists, surgeons, radiation oncologists, pathologists, and interventional radiologists with the goal of treatment being long-term survival while minimizing morbidity and maximizing function. This chapter presents the role of the interventional radiologist in the multidisciplinary team for the management of these patients. IMAGE GUIDANCE The use and advances in image guidance are essential in the diagnosis and treatment of these patients. These advances in imaging guidance, percutaneous ablation techniques, and embolotherapy have led interventional radiologists to play a large role in the multidisciplinary management of these patients. Image-guided core-needle percutaneous biopsy is one of the most common techniques and is routinely performed for the diagnosis of the initial tumor.3,4 Here we discuss the different types of imaging guidance used by interventional radiologists in the management of these patients. Ultrasound Ultrasound (US) technology has been used to image the body for over 50 years.5 Benefits of this technology is that it is portable, without radiation exposure, and inexpensive. For image guidance during a procedure, the needle or probe adjustments can be seen in real time rather than requiring intermittent scans. The US pulse (frequency range of 2–15 MHz) is sent from a transducer and echoes are generated by the interaction with structures in the tissue medium. Imaging is difficult for deeper structures, patients with larger body habitus, and through tissues with high acoustic impedance increases, for example, bone. Color Doppler evaluation can help in identifying vessels and avoiding such structures. Magnetic Resonance Imaging As with US, MRI does not use radiation to obtain information. The soft tissue delineation of MRI is exquisite. This technology combines the external magnetic field provided by a magnet with the localized magnetic field provided by gradient and shim coils with the application of radiofrequency (RF) pulse from the RF coils.6 Adjusting the pulse sequences can provide unique information regarding differentiation of pathologic disorders or evaluating treatment response. Given their complexity, scans often take longer than US or CT evaluation, and are more expensive. Computed Tomography CT involves the use of ionizing radiation with the use of x-rays through the patient, creating a three-dimensional (3-D) image data set. CT is best used when high spatial resolution is needed and for rapid imaging of a patient. If malignancy cannot be excluded on plain film in bone sarcomas, MRI should be used to characterize the lesions over CT. MRI is also preferred in the initial diagnosis of soft tissue sarcomas (STS), but CT can be used to characterize calcified lesions along with retroperitoneal tumors. In the workup of STS, a chest-spiral CT is mandatory for staging purposes.3,4 Interventional radiologists use CT to guide many procedures. In the diagnosis and treatment of sarcomas, complex biopsies are often done under CT guidance. Percutaneous radiofrequency ablation (RFA), microwave ablation (MWA), and cryoablation are also performed with CT guidance. 388Positron-Emitting Tomography/Computed Tomography The basis of PET imaging is infusing the patient with proton-rich radioactive nucleotides that emit positrons via B+ decay, which is composed of a positron and a neutrino. This decay leads to a more stable nucleus. The positron travels a very short distance before colliding with an electron in an annihilation event, which produces two photons of equal energy. These photons are detected by the scanner. The F-18 isotope gets incorporated into a glucose analog called 18F-deoxyglucose, which is given to the patient prior to scanning. This molecule gets taken up by highly metabolic tissue, which will often be abnormal oncologic tissue. In sarcoma PET/CT has been studied in diagnosis, grading, staging, biopsy guidance, therapeutic monitoring, and prognostication. However, the cost effectiveness of performing PET/CT versus the standard of care in these areas has not been studied. Interventional radiologists have used PET/CT guidance in biopsies and ablations in these patients. For biopsies, the PET/CT will show the most metabolically active portion of the tumor. In ablations, interventional radiologists can monitor the tumor response to the ablation and areas of necrosis using PET/CT. Future studies in cost effectiveness when compared to the standard CT guidance for complex biopsies and ablations need to be performed before advocating PET/CT guidance as first line.7 Fluoroscopy Fluoroscopy is an imaging technique that uses x-rays, ionizing radiation, to create real-time moving images of a patient. Interventional radiologists use fluoroscopy to guide many different types of procedures in the treatment of these patients. Intra-arterial embolotherapy is guided by fluoroscopy as well as the placement of lines, catheters, and ports. CENTRAL VENOUS ACCESS Obtaining central venous access via placement of a central venous catheter (CVC) is becoming one of the most common procedures done by an interventional radiologist. It is particularly prevalent among oncology patients, as central venous access is required for both diagnostic and therapeutic interventions. Trained interventional radiologists, with the assistance of image guidance, can routinely insert long-term venous access devices, a procedure that has shown to be safe, cost-effective, and efficacious. By using real-time imaging (fluoroscopy and/or sonography) CVCs are placed with their tip in the right atrium or at the cavo-atrial junction. While it is possible to place CVCs without image guidance, radiologically guided percutaneous insertion has higher technical success rates, shorter procedure times, lower cost, and fewer complications, making it the preferred approach to gain central access.8 Types of Devices Peripherally inserted central catheters (PICCs), tunneled central catheter, and implanted ports are the most common devices used for central venous access. Benefits and indications for each type are discussed next. Most devices are made of silicone or polyurethane, which have been associated with fewer infections when compared to previously used materials.8 Catheters can have one, two, or three lumens, allowing for multiple therapies to be administered simultaneously. Terminal end holes or side valves are designed to allow fluid injection and blood aspiration, while preventing blood or air from entering the catheter when it is not in use. Peripherally Inserted Central Catheter PICCs are nontunneled central catheters that are inserted through a vein in the arm. They are generally used in patients who require less than 3 months of central venous access for antibiotic administration or peripheral nutrition. PICCs can be used in the outpatient or inpatient setting among patients requiring long-term continuous or intermittent infusions. PICC placement is less invasive than placement of other CVCs, but they have a higher rate of infection and malfunction (fourfold increase when compared to ports); thus, risks and benefits must be considered before PICC placement.8 Tunneled Catheters Tunneled catheters are recommended in patients requiring more than 1 month of central access. They can be used for chemotherapy administration, dialysis, plasmapheresis, or total parenteral nutrition. The catheter travels through an 8- to 15-cm subcutaneous tract, usually 5 to 10 mm beneath the surface of the skin, before entering the venous system.9 The proximal end of the catheter contains a cuff, which induces an inflammatory reaction within the subcutaneous tissue. Three to 4 weeks after placement, 389the inflammatory reaction leads to fibrosis and catheter fixation. This inhibits migration of organisms down the catheter, thus reducing the incidence of catheter-related infections. Some chemotherapies, particularly trabectedin for STS, have shown to have a local sterile inflammation along the catheter trajectory.10 Placing the tunnel deeper in the subcutaneous tissue has been shown to be the best way to prevent this complication.10 When central venous access is no longer required, infusion of local anesthetic and blunt instrument dissection are necessary to pull the cuff free. Ports Ports consist of a tunneled catheter that terminates in a subcutaneous, self-sealing reservoir. The reservoir has a silicon window that can be accessed through the skin with a noncoring needle. The reservoir is normally placed in the subcutaneous tissue of the chest (preferred) or arm (usually near the antecubital fossa). Ports are generally indicated in patients who require >3 months of intermittent central venous access, and are often used for chemotherapy, chronic transfusion, or chronic blood tests.9 Ports have the lowest rate of infection when compared to tunneled catheters and PICCs.11 Indications The indications for central venous access are numerous and are notably prevalent in oncology patients who often require venous access for the duration of their disease. Therapeutic indications for central venous access include administration of chemotherapy, total parenteral nutrition, blood products, intravenous medications, fluids, plasmapheresis, and/or hemodialysis. Diagnostic indications include establishing diagnosis/prognosis, monitoring response to treatment, and/or repeated blood sampling.11 Central venous access is recommended for boluses of vesicant drugs and is essential for continuous infusions of those agents.8 Guidelines exist to aid in deciding which type of device to use and are summarized in Table 30.1. Devices with multiple lumens are indicated in patients who receive multiple simultaneous therapies or require peripheral nutrition in addition to chemotherapy. Although many factors are considered when choosing a device, patient involvement in the decision has been shown to result in greater patient satisfaction, fewer delays in therapy related to loss of vascular access, fewer device complications, shorter hospital stays, fewer ED visits, and decreased costs.8 Thus, it is important to remember that patients should always be involved in decision-making. Contraindications Owing to the safety of the procedure and the benefits it offers to patients, absolute contraindications to central venous access are rare. Relative contraindications include coagulopathy, thrombocytopenia, and sepsis. It is preferred that a patient with bacteremia or sepsis be afebrile for 48 hours prior to the procedure, though it can still be done in emergent situations. International normalized ratio (INR) <2 and platelet count <25,000 are considered contraindications, but infusion of platelets or fresh frozen plasma can be used to circumvent this issue in emergent cases.12 Additionally, studies show that experienced physicians can safely perform CVC placement in the presence of abnormal coagulation parameters.13 Cannulation is generally avoided in sites with anatomic distortion or vascular injury, but a different site of entry can generally be selected in order to gain central access. Patient Workup and Preparation In most patients, the right internal jugular vein is the preferred access site because of its large size and proximity to the skin. It also provides a short, straight route to the superior vena cava and right atrium. Patients receiving a PICC are generally accessed via the basilic vein for similar reasons: it is closer to the surface and larger than surrounding veins, thus resulting in decreased vasospasm and fewer complications.8 These sites should be examined in all patients to rule out any anatomic variations or deformity.9 Coagulation parameters should be checked and corrected before the procedure. With the exception of PICCs, placement of a CVC requires the same aseptic environment found in the operating room. TABLE 30.1 Guidelines for Central Venous Access Device Selection PICC, peripherally inserted central catheter; TPN, total parenteral nutrition. Source: Reproduced with permission from Valji K. Vascular access placement and foreign body retrieval. In: Valji K, ed. The Practice of Interventional Radiology. Philadelphia, PA: Elsevier; 2012:559–580. 390Follow-Up Postprocedure imaging to confirm successful placement may include fluoroscopy, a chest radiograph, or contrast injection through the catheter.14 Standard of practice is to position the catheter tip in the upper to mid-right atrium with the patient supine, allowing for migration of the tip when the patient switches from the supine to standing position.12 Technical success is defined as catheter introduction into the desired location, with adequate catheter function. Catheter function is defined as withdrawal of blood and infusion of saline without significant resistance. Nursing staff should be well trained in venous access care and able to recognize device failures and identify and treat potential complications.14 Complications and Troubleshooting Complications are rare, with success rates >96%. Complications can be classified as early (<24 hours after placement) and late (>30 days after placement).14 Selected complication rates when using image guidance can be seen in Table 30.2. Some of the more common and serious complications are discussed individually. Infection Chemotherapy and radiotherapy can cause significant neutropenia, and over 60% of catheter-related sepsis (CRS) occurs during periods of neutropenia. Oncology patients with central venous catheters are therefore at an increased risk of catheter-related bloodstream infection (CRBSI.) CRBSI is defined as at least two blood cultures positive with the same organism, obtained from two different sites at two separate times, in association with evidence of colonization of the catheter with the same organism. This differs from central line–associated bloodstream infection (CLABSI), which is defined as recovery of a pathogen from a blood culture (single if not related to the skin or two or more cultures for an organism commonly present on the skin) in a patient who had a central line at the time of infection or within 48 hours developing an infection.15 The most common symptoms associated with infection are fever or chills followed by erythema/induration at the insertion site.15 Measures can be taken to reduce the incidence of CRBSI. Insertion should occur with appropriate hand hygiene, maximum sterile barrier precautions, and proper skin preparation. Dressings at CVC sites should be changed weekly until the insertion site has healed, and then dressings should no longer be used. Site selection can also decrease infections, with internal jugular placement showing lower rates of infection compared to femoral. Devices impregnated with a combination of minocycline and rifampin have been shown to reduce infection rates, and current evidence suggests that such catheters are cost effective for high-risk patients compared with standard CVCs. Use of prophylactic antibiotics before central venous access is controversial, and there are no current recommendations for their use. Catheters should be removed promptly when no longer needed. TABLE 30.2 Central Venous Access Complication Rates PICC, peripherally inserted central catheter. Source: Data from Dariushnia SR, Wallace MJ, Siddiqi NH, et al. Quality improvement guidelines for central venous access. J Vasc Interv Radiol. 2010;21(7):976–981. doi:10.1016/j.jvir.2010.03.006. 391Management centers around the isolated organism and its antibiotic sensitivities. Antibiotics should be administered, and then a decision to remove the catheter or to keep it in place must be made. Patients with limited access may benefit from keeping the catheter in place, even in the setting of infection. The catheter should be removed if the patient has unexplained sepsis, erythema, or purulence at the catheter insertion site, or CRBSI associated with supportive thrombophlebitis, endocarditis, or osteomyelitis. Likewise, it should be removed if the organism isolated from blood cultures is Staphylococcus aureus.8,16 Thrombosis Thrombotic complications are relatively common and are generally marked by catheter malfunction or symptoms of deep vein thrombosis (DVT). The buildup of fibrin around the catheter can cause a partial or complete occlusion of the lumen. Thrombosis occurs as intraluminal thrombosis, fibrin sheaths, and mural thrombosis. Complications include pulmonary embolism, DVT recurrence, and postphlebitic syndrome. Prevention can be accomplished by selecting the proper device and access point, and ensuring proper tip placement. Silicone and polyurethane are now the most common materials used because of their decreased risk of thrombosis. A greater vessel length increases the risk of thrombosis; therefore, the shortest possible route should be selected. Device placement against the wall of the right atrium has been shown to increase risk of thrombosis; therefore, the correct anatomic placement is crucial. Image guidance reduces endothelial damage and decreases the risk of catheter-associated thrombosis. Catheters should be flushed routinely with saline or unfractionated heparin. Tissue plasminogen activator (tPA) can be used weekly as a locking solution. There is inadequate evidence to support the use of routine heparin or warfarin for prevention of thrombosis. Management of partial thrombotic occlusion includes unfractionated heparin 5,000 to 25,000 units over 6 to 24 hours. Complete occlusion requires one to two doses of urokinase or recombinant tPA. Fibrin sheaths can be treated with a catheter exchange, angioplasty balloon, or fibrin sheath stripping, all of which are reserved for instances when pharmacologic therapy fails to reestablish patency. Patients with catheter-related DVT should be placed on anticoagulation for a minimum of 3 months. Catheters affected by thrombosis should be removed whenever possible. Some studies show utility in thrombolysis. Catheter-directed thrombolysis has been shown to be safer than systemic thrombolysis, and can be considered in patients with extensive thrombosis.8 Catheter Malfunction Catheter malfunction manifests as increased resistance on infusion or the inability to aspirate blood. Patients may also complain of pain during injection. Such patients should be evaluated promptly for evidence of device malfunction. Troubleshooting consists of physical exam, chest radiograph, or injection of contrast to assess device position and integrity. The most common cause is fibrin deposition and venous thrombosis, as mentioned previously. Other causes include catheter malposition, kinking, and catheter fracture.9 Occlusion can also be due to precipitation of drugs with low or high pH or lipid-rich parenteral infusions. Such occlusions can be treated with sodium bicarbonate, hydrochloric acid, or ethanol, respectively. These agents should fill the catheter for up to 20 minutes.8 Nonfunctioning catheters with no signs of infection can usually be replaced over a guidewire through the original tunnel. BIOPSY Biopsy of suspected sarcoma is the diagnostic standard and is necessary prior to the development and application of treatment strategies.17 In an age when personalized oncology and targeted therapies provide the best outcomes, determining relevant cellular and molecular signatures of tissue via a histologic sample is critical. Different approaches exist, including open biopsy, fine-needle aspiration, and percutaneous core-needle biopsy. Image-guided percutaneous biopsy has been shown to be safe, effective, and considerably less invasive than open biopsy. Positive and negative predictive values, 392sensitivity, and specificity of tissue samples obtained via image-guided percutaneous biopsy have been reported to be similar to those found in open incisional biopsies.18,19 Biopsies of suspected sarcomas are technically challenging, high-risk procedures. They should only be done at institutions with specialty sarcoma centers made up of an interdisciplinary team. Indications Percutaneous biopsy of bone or soft tissue is performed whenever histologic confirmation is required for definitive diagnosis and/or treatment. Soft tissue tumors are often heterogeneous and it can be difficult to determine if they are benign or malignant from imaging alone. Benign soft tissue masses are 100 times more common than malignant sarcomas, and it can therefore be challenging to determine which soft tissue masses require further workup, evaluation, and biopsy. Published criteria for referral to sarcoma center for potential biopsy of a soft tissue lesion include any lesion that is >5 cm, painful, rapidly increasing in size, deep to the muscle fascia, or recurrent after previous excision.20 Other common indications are to assist in staging or to determine if a mass is secondary to metastasis or primary sarcoma when PET/CT is equivocal.21 Contraindications There are no absolute contraindications; however, there are a handful of relative contraindications in which risk versus benefit should be carefully considered. Biopsy is not indicated in a patient if the results will not change management or if surgery is indicated irrespective of the outcome of the biopsy.22 Biopsy may be contraindicated in patients that exhibit uncorrectable coagulation abnormalities. Severely compromised cardiopulmonary function or hemodynamic instability could potentially incur too much risk to safely complete the procedure.23 Lesions that are located in a position where there is no safe percutaneous approach should be avoided. Additionally, caution should be taken when performing an image-guided percutaneous biopsy in a patient who is unable or unwilling to limit movement during the procedure. If repeat core-needle biopsy is unable to obtain adequate tissue for diagnosis, an incisional biopsy may need to be performed.21 Approach Patients should undergo INR and activated partial thromboplastin time (aPTT) (if receiving heparin) prior to the procedure. If INR is less than 1.5 it should be corrected. It is recommended that platelets be transfused if platelet count is less than 50,000. Low-molecular-weight heparin (LMWH) (1 day) and clopidogrel (5 days) should be withheld before the procedure. There is no need to withhold aspirin.13 Generally, the shortest path to the lesion is considered when planning the imaging approach. Choice of imaging modality for biopsy is based on lesion characteristics and operator preference. The most common modalities are fluoroscopy, sonography, and CT, each having its own advantages and disadvantages. Fluoroscopy is commonly used for biopsy of lung and pleural masses and is useful for its ability to give real-time images. Disadvantages include increased radiation exposure and inability to visualize adjacent structures. Ultrasound is used for biopsy of intra-abdominal lesions, masses in the retroperitoneum, and other peripheral lesions. Advantages include the lack of radiation, real-time imaging, and multiplanar imaging. Disadvantages include impaired visualization of deep lesions and obscuration by overlying structures. CT is reserved for biopsy of smaller or deeper lesions not well visualized by sonography or fluoroscopy. Advantages include high-quality images and the ability to clearly visualize all surrounding structures. Disadvantages include increased radiation, lack of real-time feedback, and patient movement after capturing the CT image. CT fluoroscopy can be used to obtain near real-time imaging when necessary. Single-needle and two-needle techniques can be used in obtaining the biopsy. In the single-needle technique, a needle is advanced into position and imaging is obtained. If the needle is not positioned in the desired location, it is left in place to guide the placement of a second needle. Each biopsy then requires a new needle to be placed in the lesion, allowing for sampling of different regions of the mass. In the two-needle technique, the initial needle is inserted into the desired location within the mass and is used as the landmark for subsequent needles. With the coaxial method, a large needle is inserted just superficial to the mass. The biopsy is then performed with longer, smaller-gauge needles, allowing for a single puncture of viscera or pleura.22 A multidisciplinary team should be assembled to treat all patients with sarcoma. Interventional radiologists should work closely with the medical and radiation oncologists and pathologists to 393determine the best treatment and biopsy approach for the patient. Additionally, they should be in close communication with the surgeon to determine the best route for biopsy in the event that the biopsy tract needs to be resected (see Table 30.3). TABLE 30.3 Percutaneous Needle Biopsy Complication Rates Source: Data from Gupta S, Wallace MJ, Cardella JF, et al. Quality improvement guidelines for percutaneous needle biopsy. J Vasc Interv Radiol. 2010;21(7):969–975. doi:10.1016/j.jvir.2010.01.011. Multiple passes may be needed owing to the heterogeneity of many sarcomas. After the biopsy is completed, imaging can be performed to exclude potential complications. The patient should be monitored for up to 4 hours, with frequent monitoring of vital signs. The patient may be discharged home if vital signs remain stable.22 Complications Complications are rare, with a rate of 1.1% in image-guided percutaneous needle biopsy (PNB) of musculoskeletal (MSK) lesions.19 Complication rates of PNB in general are shown in Table 30.3. Compartmental anatomic guidelines for biopsy of MSK tumors have been published and used with the hopes of decreasing complication rates. Recommendations are extensive and include avoidance of critical structures and breaching more than one anatomic compartment. However, recent studies have shown that nonadherence to these recommendations does not result in increased complications or tumor recurrence.24 Tract Seeding and Tumor Spillage In theory, disruption of the tumor pseudocapsule with the biopsy needle could result in tumor cells spilling into adjacent tissue and seeding of the biopsy tract. Rates of needle tract seeding (NTS) vary for different tumors, with the incidence in hepatocellular carcinoma (HCC) as high as 3%. In such cases, pretreatment biopsy is rarely used. However, in suspected sarcomas, NTS is obscure and a diagnosis without biopsy is frequently unreliable. Correct treatment cannot be safely initiated without diagnosis; therefore, biopsy is routinely performed. Studies are inconclusive, but recent reports indicate that there is no significant increased risk of NTS in soft tissue biopsies. One study showed that 13% of needle tracts had evidence of sarcoma cells, but those tracts did not have an increased incidence of tumor recurrence. Case reports of tumor recurrence along the biopsy tract seem to be decreasing, perhaps due to the widespread use of coaxial sheathed biopsy needles. However, because of existing case reports and the paucity of quality evidence regarding NTS in sarcoma, it is generally recommended that the biopsy trajectory be planned in conjunction with a team of surgeons so that the tract is resected with the tumor in order to avoid any potential complications.25 Pneumothorax Pneumothoraces occur commonly in transthoracic biopsies and can also occur (although not as commonly) in nontransthoracic biopsies. Complications can be divided into major and minor complications. Chest tube placement is required if a pneumothorax becomes symptomatic or continually enlarges. Pneumothorax requiring thoracotomy tube placement is considered a minor complication if it only requires an overnight hospital stay. It is considered a major complication if hospitalization is longer than 48 hours for management of a persistent leak, or if the pneumothorax results in delay of chest tube removal for more than 3 days. Measures can be taken to reduce the incidence of pneumothorax. Patients should be instructed not to move, talk, cough or breathe deeply during and immediately after the procedure. If possible, the pleura should only be punctured once and a coaxial technique should be used.26 Pneumothorax rates range between 17% and 26%; however, only 1% to 14% of patients require a chest tube.26 Risk factors include chronic obstructive pulmonary disease, depth of lesion, size of lesion, and number of pleural punctures.26 394TRANSARTERIAL APPROACH With the introduction of the Seldinger technique of catheterization, arterial access evolved in the 1970s and continues to do so. Access sites have evolved to less minimally invasive approaches from translumbar, to transaxillary to transfemoral and now transradial.27,28 Angiography offers a diagnostic evaluation paired with a unique minimally invasive access to tumor vasculature that can help decrease perioperative hemorrhage, permit targeted chemotherapy delivery, or perform focused brachytherapy. These procedures can last anywhere from less than 2 hours to over 4 hours. While patients only require a combination of local anesthesia and conscious sedation, general anesthesia may be provided. Postprocedure care is centered around decreasing access site complications and supportive care. For the former, this may include lying flat for up to 6 hours if femoral access is used. This is not required with radial artery access.28 Contraindications Angiographic intervention is contraindicated in a medically unstable patient with multisystem organ failure.29 Relative contraindications include cardiac abnormalities, such as a recent myocardial infarction, serum electrolyte imbalances, severe contrast agent reactions, impaired renal status, inability to lay flat (agitation or impaired respiratory status), pregnancy, and Ehlers–Danlos syndrome.27,29 Severe contrast reactions are marked by angioedema or bronchospasm. Dermal reactions can be managed with premedication. Impaired renal status may be addressed with prehydration or limiting the use of iodinated contrast agent by replacing it with carbon dioxide (CO2.) Patients with Ehlers–Danlos syndrome have a high risk for arterial injury, such as arterial dissection.29 Complications Puncture Site–Related Complications Common arterial injuries caused by femoral artery catheterization include hemorrhage, postcatheterization pseudoaneurysm formation, arterial obstruction, arteriovenous fistula, and limb amputation.27,30 Incidence rates are reported between 0.05% and 14%.30 Risk factors include obesity, use of anticoagulation, use of large-caliber sheaths in access (greater than 7F [French]), puncture above the inguinal ligament, brief manual compression, hypertension, calcified atherosclerotic arteries, and history of hemodialysis, to name a few.30 Pseudoaneurysm formation presents with pain, swelling, and dramatic bruising around the puncture site after recent arterial puncture. Diagnosis is made with US, which would reveal a mass with to-and-fro flow pattern on color Doppler mode.30 While spontaneous thrombosis has been seen in pseuodoaneurysms less than 3 cm in size, management by US-guided compression, US-guided thrombin injection, coil embolization, stent placement, or surgical repair is recommended, given risk of rupture.30 Contrast-Induced Nephropathy Contrast-induced nephropathy (CIN) is defined as a 0.5 mg/dL or 25% to 50% increase in serum creatinine or a 25% or greater decrease in estimated glomerular filtration rate (eGFR). This change can be seen as early as 24 hours or up 7 days after contrast administration.29,31 Risk is low if renal function is normal.29 If the patient has a eGFR <30 indicating stage 4 or 5 chronic kidney disease, incidence has been reported as high as 40%.29 Other risk factors include dehydration, diabetes mellitus, congestive heart failure, and the use of angiotensin-converting enzyme inhibitors.29,31 Prevention with hydration with 0.9% saline prior to the procedure has been effective.32 Behavior modification of the operator has also been shown to help, examples of which include diluting contrast agent or using alternative agents, such as CO2.31 Postembolization Syndrome Postembolization syndrome (PES) presents as fatigue, nausea, vomiting, abdominal pain, and/or fever.29,33 Incidence ranges between 60% and 90% with chemoembolization, and is also seen in bland embolization of the liver.29 Possible causes include a systemic inflammatory response caused by the ischemia or necrosis of hepatocytes. The incidence can be reduced with the administration of dexamethasone.33 The remainder of care is supportive with antiemetics, pain control, and hydration, and can lead to prolonged hospitalization.33–35 With radioembolization, the symptoms are milder; therefore, it is classified as postradioembolization syndrome (PRS), which includes fatigue, nausea, anorexia, fever, abdominal discomfort, and cachexia.29,34,35 Incidence ranges between 20% and 55% and can be minimized with postprocedure course of steroids and antiemetics.34 395Radiation Safety Intra-arterial procedures require fluoroscopic guidance; therefore delivering significant doses of radiation to the patient and the operator.29,36 Tissue reactions are deterministic in that there is a threshold dose below which no injury is seen (2 Gy). Cancer risks from unrepaired or incorrectly repaired DNA are stochastic, indicating a linear, no threshold model. In other words, as exposure increases, so does risk. Given that sarcoma patients are often young, the risk of induction of cancers, such as leukemia or thyroid, skin, breast, or brain cancer, is greater.29,37 Strategies to decrease dose include decreasing procedure time, effective shielding, using personal protective equipment, and improved equipment, which allows low dose rates without compromising on imaging quality.29,36,38 Indications Liver Metastases Locoregional therapy that has been used to manage primary liver cancer and metastatic disease, particularly colorectal cancer, includes transcatheter arterial embolization (TAE), transcatheter arterial chemoembolization (TACE), and transcatheter arterial radioembolization (TARE.) This has led to transarterial approach to treat sarcoma metastases in the liver, particularly the mesenchymal tumor, gastrointestinal stromal tumor (GIST.)39 Incidence of liver metastases is 55% to 72% of the time with GIST and control of metastases is a determinant of survival.40,41 Special considerations when evaluating intra-arterial liver metastases include performance status and liver function.40 It is preferred that liver function is Child-Pugh class A or B, as nontarget embolization or treatment can potentiate liver failure.40 Radioembolization requires a closer attention to bilirubin levels; they should be less than 2 mg/dL given risk of radiation-induced liver disease (RILD.)34,35 RILD results from radiation exposure to normal liver parenchyma and rates are reported between 0% and 4%.34 Centers use a bilirubin of less than 3 mg/dL as a cutoff for TAE or TACE.40,41 This technique exploits the dual supply to the liver; therefore, the portal vein is preferred to be used for patients with hepatopedal flow.41 Risk of liver injury may be mitigated by decreasing the amount of liver parenchyma exposed, which can be performed by favoring a subselective technique over a lobar or segmental approach. In this approach, the liver lesions are targeted through their third and fourth order arterial branches with a microcatheter, decreasing exposure of embolic to uninvolved segments. A sequential lobar approach could be used if there are diffuse metastases with interventions spaced out between 2 and 4 weeks, targeting the lobe with the most extensive involvement first.41 Procedure time is determined by anatomy and approach; for example, subselective embolization requires more time. TAE and TACE can be repeated in regular intervals, with imaging follow-up obtained 1 month after the procedure.41,42 TARE requires a mapping angiogram to evaluate tumor supply, embolize branches to bowel, and evaluate lung shunt function.43 Lung shunts greater than 20% can limit ability to give a dose that is effective at treating the tumor, without damaging lung parenchyma.34,43 Treatment is then delivered at most centers 10 to 14 days after mapping procedure.43,44 Imaging follow-up is performed 3 months after delivery of the radioembolic therapy as radiation changes can be mistaken for progression.43,44 With TAE and TACE, different embolics include gelfoam reabsorbable sponges to particles, such as polyvinyl alcohol (PVA) or trisacyl microspheres. There are two types of radioembolic devices with yttrium embedded in glass spheres or on resin microspheres. With chemoembolization, different agents include cisplatin, mytomycin C, doxorubicin, and vinblastine. Some series mix GIST with leiomyosarcoma (LMS). An example of bland embolization for GIST and LMS in shown in Figures 30.1 and 30.2, respectively. Patients with GIST tend to do better than those with LMS.45 The addition of a targeted agent, such as imatinib, improved overall survival (OS).41 There is evidence to support embolization in patients with GIST who are imatinib resistant; however, patient performance status drives survival benefit.40,42,46 There is also evidence to support embolization in LMS resistant to chemotherapy.47,48 Extrahepatic disease, poor imaging response, and progression after embolization were associated with poor OS.41 Initial data with TARE are promising; one multicenter study between four institutions with the majority of patients with LMS (GIST excluded) showed an OS of 30 months treated with resin and glass microspheres, depending on institution protocol.49 A summary of these studies is included in Table 30.4. Given that patients often have had prior surgery, attention to prior biliary interventions is needed.40,52 This can result in an increased risk of infection or biloma formation, for example, in the series by Maluccio. Two patients developed abscess requiring intervention and one bactermia.34,45,52 One patient in the series also developed a duodenal stricture from nontarget embolization, requiring 396surgical bypass.45 In the TARE series with 39 patients by Miller et al, one patient with a hepatojejeunostomy developed a liver abscess.49 The other grade 3 adverse events included one gastroduodenal ulcer and one patient developed radiation pneumonitis.49 In a second series of TARE with 11 patients, one developed a gastric ulcer.51 A strategy to mitigate risks when a patient with an incompetent ampulla of Vater is to begin preprocedure antibiotics days prior to the procedure and follow an extended course of postprocedure antibiotics.34,52 FIGURE 30.1 A 40-year-old man diagnosed with GIST after a small bowel tumor was seen during workup for abdominal pain. His original tumor was an over 9 cm mass. He was maintained on imatinib until 4 years later when he was found to have progression of liver lesions. The dose of imatinib was increased; he underwent bland embolization. His liver function was preserved (Child-Pugh class A) and his performance status was excellent (ECOG grade 0). Bland embolization was performed and showed dramatic response likely related to the adjustment of the imatanib dosing and embolization. Over the next 5 years, he underwent eight different bland embolizations to control the liver lesions. He was given different targeted agents including sunitinib, regorafanib, pazopanib, and ponatinib. Just before succumbing to the disease in the liver, omentum, and mesentery, his liver function deteriorated with elevation of his total bilirubin to 1.8 mg/dL. (A) Contrast-enhanced axial image showed multiple bilateral hypoattenuating lesions. (B) After embolization, lesions have decreased in number and enhancement. (C) Angiogram showing common femoral artery access over the midfemoral head away from the bifurcation. (D) Angiogram showing conventional anatomy of the celiac axis. ECOG, Eastern Cooperative Oncology Group; GIST, gastrointestinal stromal tumor.
Device
Length of Use
Common indications
PICC
<3 months
Antibiotics, TPN
Tunneled catheter
>1 month
Chemotherapy, TPN, dialysis, plasmapheresis
Implantable port
>3 months
Chemotherapy, chronic transfusion, chronic blood tests
Complication
Rate (%)
Tunneled catheter and port placement
Pneumothorax
1–3
Hematoma
1–3
Perforation
0.5–1
Air embolism
1
Thrombosis
4
Procedure-induced sepsis
1–3
PICC placement
Pneumothorax
0
Hematoma
1
Phlebitis
4
Thrombosis
3
Procedure-induced sepsis
1
Complication
Rate (%)
Bleeding requiring intervention
0.1–8.3
Tract seeding
0.3–4.0
Pneumothorax
0.5
Pneumothorax in transthoracic percutaneous needle biopsy
12–45
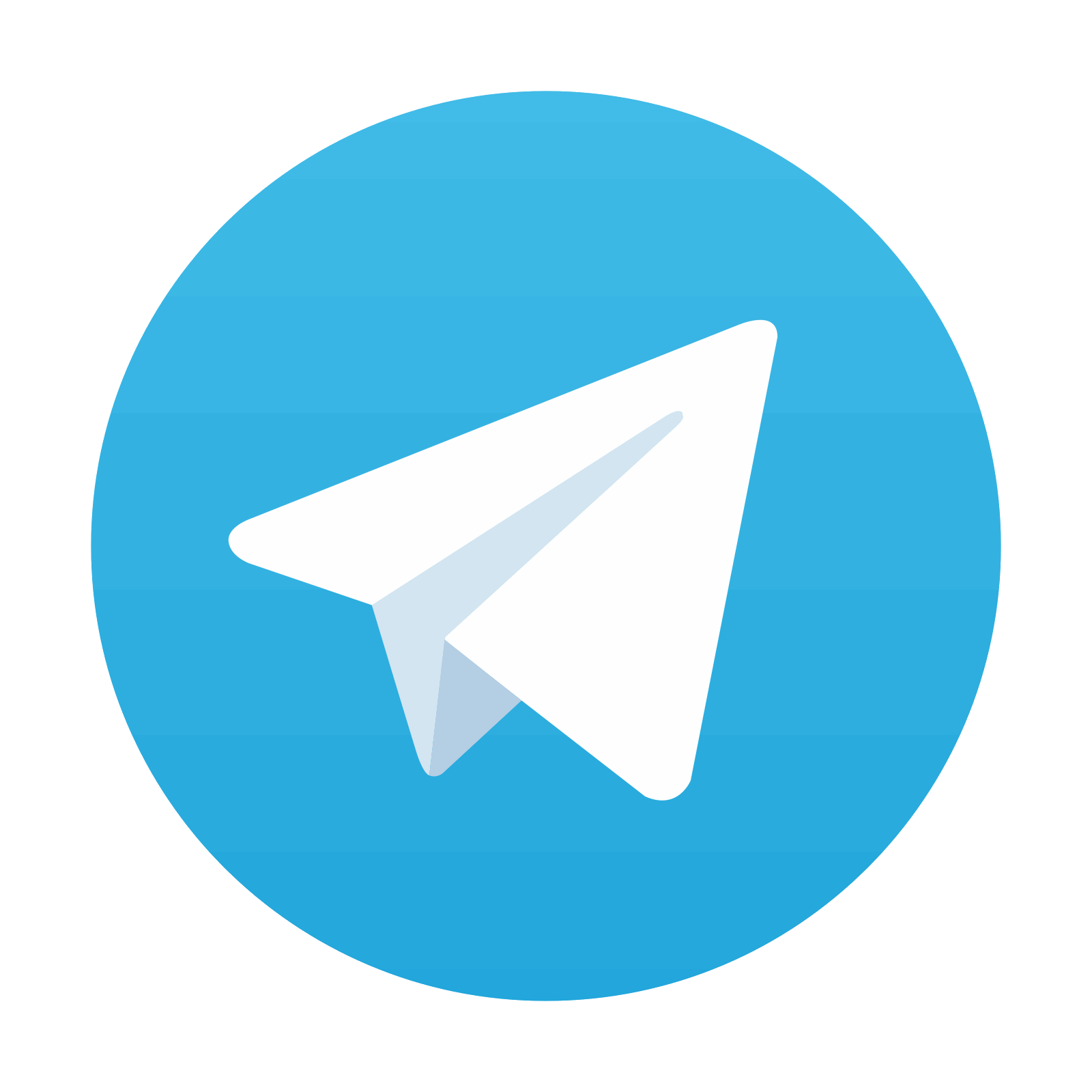
Stay updated, free articles. Join our Telegram channel

Full access? Get Clinical Tree
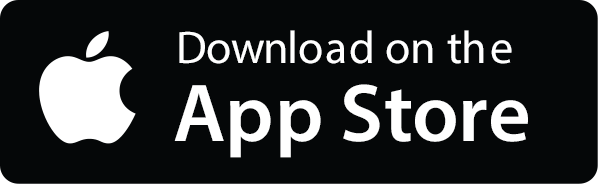
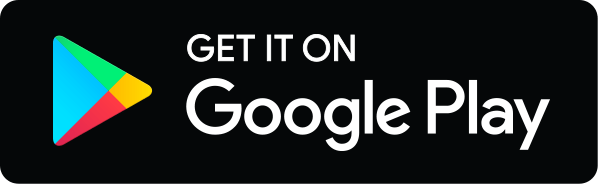