Intermediate- and High-Risk Prostate Cancer
In 2010, an estimated 218,000 new cases of prostate cancer were diagnosed in the United States, representing approximately 30% of all nondermatologic cancers.1 Prostate cancer was the second leading cause of cancer death in men, responsible for more than 30,000 deaths.1 Most of the patients destined to die from prostate cancer initially presented with unfavorable intermediate- or high-risk disease. This chapter focuses on the management of such patients. Since the previous edition of this book, our understanding of prostate cancer has expanded substantially. With several prospective studies now demonstrating a survival advantage, hormonal therapy has become widely accepted as an integral part of treatment. Interest in adjuvant and salvage radiotherapy has been brought to the forefront with recently released prospective studies. With more mature datasets, our ability to prognosticate extends beyond merely biochemical control, but now includes distant metastases, prostate cancer-specific survival, and overall survival. High–dose-rate brachytherapy is being increasingly adopted by centers as a means to further dose escalate. Details on the management of low-risk prostate cancer and the background of prostate cancer in general can be found in Chapter 65.
EPIDEMIOLOGY
With increasing prostate-specific antigen (PSA) screening since the early 1990s, the clinical presentation of prostate cancer has changed dramatically, shifting from locally advanced or metastatic disease to clinically nonpalpable disease. Data from two national prostate cancer registries, the Cancer of the Prostate Strategic Urologic Research Endeavor (CaPSURE) and the Department of Defense Center for Prostate Disease Research (CPDR), highlight this stage migration.2–4 According to CaPSURE, the diagnosis of high-risk disease has seen a precipitous drop from 27.4% in 1990 to 1994 to 13.7% in 2004 to 2007.3 Similarly, the proportion of patients presenting with a pretreatment PSA >20 ng/mL has dropped from 27.0% to 8.1% in the same time periods. Conversely, clinically nonpalpable disease (stage T1) has surged from 16.9% to 49.4%, respectively. Data from CPDR mirror these findings. From 1988 to 1998, clinical stage T3 to T4 disease contracted from 19.2% to 4.4%, whereas T1c disease went from 0% to 47.8%.4
CLINICAL PRESENTATION
Patients with intermediate- or high-risk prostate cancer may present with locoregional symptoms, but this is not common. Lower urinary tract symptoms that may be seen include nocturia, urinary frequency, urgency, decreased flow, incomplete voiding, intermittent flow, or hesitancy. More bulky primary disease may present with difficulty in passing stool or even bloody stool. With increasing PSA and Gleason score, the risk of metastases increases. Metastatic spread is generally sequential, proceeding from the prostate to the pelvic lymph nodes then bone. Thus patients with metastatic disease may present with renal failure, lymphedema of the lower extremities, and bone pain.
PROGNOSTIC FACTORS AND RISK CLASSIFICATION SCHEMES
The traditional prognostic factors have been clinical T stage, presenting PSA, and Gleason score. To simplify treatment recommendations and prognostication, several risk classification schemes have been proposed by grouping patients with different clinical features, but similar biochemical outcomes.5,6 The risk classification scheme proposed by D’Amico et al.5 appears to be the most widely used, stratifies patients with clinically localized prostate cancer to three risk groups, and has since been adopted by the National Comprehensive Cancer Network (NCCN) guidelines.7 Low risk is defined as meeting all the criteria: T1c to T2a, PSA <10 ng/mL, and Gleason score ≤6. Intermediate risk is defined as T2b or T2c, PSA 10 to 20 ng/mL, or Gleason score 7. High risk is any of the following: T3a, PSA >20 ng/mL, or Gleason score 8 to 10. Locally advanced is defined as T3b or T4 disease. It has recently been validated using a study cohort of 7,316 men from two large cancer registries (CaPSURE and CPDR) and has also been found to predict for prostate cancer–specific mortality (PCSM) after radiotherapy.8 Of note, in the seventh edition of the American Joint Committee on Cancer (AJCC) prostate cancer staging, Gleason score and pretreatment PSA are now incorporated into the staging classification.9
Other pretreatment predictive factors have since been identified, including percentage of positive biopsy cores (PPC) in radiotherapy and surgery-treated patients.10–11,12 D’Amico et al.10 reported on the independent prognostic capabilities of PPC, calculated as the number of PPCs containing cancer divided by the total number of cores, of PSA control. Interestingly, 76% of patients with intermediate-risk disease could be reclassified into either low- or high-risk disease. Specifically, those patients with <34% PPC had similar outcomes as low-risk patients (5-year PSA control 85% vs. 91%, respectively), whereas patients with more than 50% PPC had similar outcomes as high-risk patients (5-year PSA control 30% vs. 43%, respectively). The prognostic significance of PPC has also been reported to extend to PCSM in intermediate-risk patients.13 When stratified by ≤50% and >50% PPC, 7-year prostate cancer-specific survival was 100% versus 57% (P = .004), respectively. In patients treated with radical prostatectomy, high PPC is associated with adverse pathologic features like extracapsular extension, seminal vesicle invasion, positive surgical margins, lymphovascular invasion, perineural invasion, and pelvic lymph node involvement.14–16
A recent meta-analysis of four Radiation Therapy Oncology Group (RTOG) trials demonstrated that older age (>70) patients were associated with a reduced risk of PCSM and metastases, even after adjusting for the expected increased risk of non–prostate cancer–specific mortality in this subgroup.17
In summary, PPC (>50%) have further refined our prognostic precision in addition to the traditional predictive factors (pretreatment PSA, Gleason score, clinical T stage). At the University of California–San Francisco (UCSF), the presence of either in intermediate-risk patients, as defined by the NCCN classification, is considered as unfavorable intermediate- or high-risk disease. Single modality local therapy is likely not enough, but rather more aggressive treatment, such as hormonal therapy and pelvic irradiation, is indicated.
GENERAL MANAGEMENT
Radiation Therapy Techniques
For details on prostate only radiotherapy, please refer to Chapter 65. This section will focus on the implementation and toxicities of whole pelvic radiotherapy.
Whole-Pelvic Irradiation
Since the previous edition, intensity-modulated radiation therapy (IMRT) has been increasingly adopted by many centers in pelvic nodal irradiation. For historical purposes, with conventional technique, pelvic irradiation is usually treated with a four-field box. The target volume usually includes the prostate, seminal vesicles, obturator, and proximal internal and external iliac nodal regions. Occasionally common iliac, para-aortic, and even perirectal nodes are included in the initial target. The traditional field borders of the anteroposterior portals are as follows: superior at the L5-S1 interspace, inferior at 2 cm distal to the membranous urethra (defined by the apex of the urethrogram peak), and 1.5 to 2 cm lateral of the pelvic brim. Corner blocks are usually placed at all four corners to limit dose to the small bowel and femoral heads. In the lateral portals, the superior and inferior borders are placed at the same point as the anteroposterior portals; the anterior border is placed at the anterior most aspect of the pubic symphysis, and the posterior border is placed at the S2–3 interspace. With CT planning, the prostate, seminal vesicles, rectum, small bowel, bladder, pelvic vessels, and penile bulb may be contoured to facilitate shielding of the rectum and small bowel.
The dosimetric advantages of IMRT have led to its investigation in delivering pelvic radiotherapy. Compared to conventional technique, IMRT allows unprecedented sparing of nearby critical structures like the rectum, small bowel, bladder, and femoral heads. In a study from UCSF, Wang-Chesebro et al.18 compared the nodal target coverage by conventional field borders with IMRT (Fig. 66.1). The nodes that were contoured included the obturator, internal or external iliac, common iliac, and presacral regions. In the conventional four-field plan, only 70.3% of the nodal target volume received the prescription dose of 45 Gy, whereas 96.2% was covered in the IMRT plan (P = .002). Even worse, conventional field placement led to 20.2% of the nodal target volume to receive <80% of the prescription dose. The rectal and bladder volume receiving 95% of the prescribed dose was significantly reduced with IMRT, with an absolute reduction of 23% and 80%, respectively.
Whereas conventional technique involved simply placing field borders based on bony anatomy, IMRT requires the delineation of a nodal target volume based on vasculature. Investigators have used lymphotropic nanoparticle-enhanced magnetic resonance imaging (MRI) and ultrasmall superparamagnetic iron oxide lymph node contrast agent, ferumoxtran-10, to develop nodal clinical target volume (CTV) models.19,20 These MRI studies have formed the foundation for the RTOG Genitourinary Radiation Oncology Consensus on pelvic nodal CTV delineation, as shown in Figure 66.2.21 However, the RTOG guidelines did not use sentinel lymph node imaging, which in a study by Chen et al.22 demonstrated that the RTOG pelvic nodal CTV included all identified sentinel lymph nodes in only 30% of patients.
In the same consensus, Lawton et al.21 recommended dose constraints to the rectum (V50 Gy ≤50%, V70 Gy ≤20%), bladder (V55 Gy ≤50%, V70 Gy ≤30%), femoral heads (V50 Gy <5%), small bowel (maximum dose <52 Gy). Based on an extensive literature search, Chan et al.23 proposed guidelines on rectal dose constraints for patients receiving pelvic radiotherapy.
FIGURE 66.1. Comparison of dose–volume histograms for rectum and bladder for whole-pelvic only phase using intensity-modulated radiotherapy (IMRT), whole-pelvis (WP) three-dimensional conformal radiotherapy (3DCRT), and extended-field (EF) 3DCRT plans. For both bladder and rectum, IMRT significantly reduced V45, V42.75, V36, and V22.5 (P <.01 for all comparisons) compared to both WP and EF 3DCRT plans. (From Wang-Chesebro A, Xia P, Coleman J, et al. Intensity-modulated radiotherapy improves lymph node coverage and dose to critical structures compared with three-dimensional conformal radiation therapy in clinically localized prostate cancer. Int J Radiat Oncol Biol Phys 2006;66:654–662, with permission from Elsevier.)
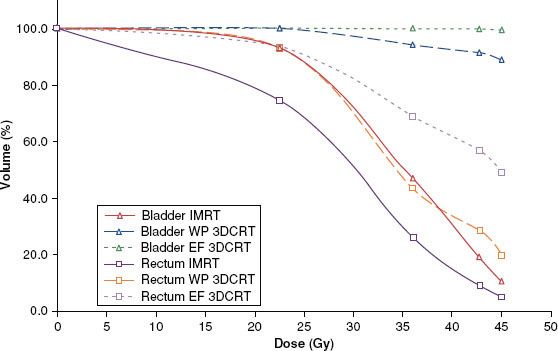
Prostate Motion
With the shift to dose escalation and IMRT and its attendant high conformality around target structures, minimization of setup errors becomes critical. The previous technique of verification of the isocenter relied on obtaining weekly port films, which only allowed comparison of bony anatomy without any consideration of the prostate and did not account for prostate motion. Questions that have since emerged are:
1. How much does the prostate move on a daily basis (i.e., interfractional) and during each treatment (i.e., intrafractional)?
2. Is the positional relationship between the prostate and bony anatomy static (i.e., can bony anatomy be used as a surrogate of the prostate location)?
The use of fiducial gold seed markers and daily electronic portal imaging (EPI) with online correction is one such strategy. In a comprehensive study from the Mayo Clinic, the inter- and intrafractional motion of the prostate and pelvic bony anatomy in 20 prostate patients, each with three or four intraprostatic gold fiducial markers, and daily pretherapy and through-treatment EPI were reported.24 With more than 22,000 data points, Schallenkamp et al.24 determined that the prostate moved as much as 9.1 mm (mean 2.5 mm) in the superior-inferior (SI), 16.3 mm (mean 3.7 mm) in the anterior-posterior (AP), and 15.2 mm (mean 1.9 mm) in the right-left (RL) axes, prior to any efforts to localize the fields to the fiducial markers. In recommending a margin for setup and organ motion (i.e., CTV to planning target volumes [PTV] margin), without fiducial marker localization, SI, AP, and RL margins of 5.1, 7.3, 5.0 mm, respectively, were required to cover 95% of the CTV with the prescribed dose with a 95% probability. With a daily fiducial marker localization protocol, the margins can be reduced to 2.7, 2.9, and 2.8 mm, respectively. The interfractional three-dimensional (3D) displacements of the prostate and bony anatomy were 5.6 and 4.4 mm prior to localization and 2.8 and 4.4 mm after postlocalization adjustments, respectively, suggesting that bony anatomy does not accurately reflect the position of the prostate. The average intrafractional displacements of the prostate and bony anatomy were 0.1 and –0.5, 0.4 and 0.4, and 0.1 and 0.3 mm in the AP, SI, and RL axes, respectively. Marker migration was found to be minimal, with 79% within 1 mm and 96% within 1.5 mm. The latter results have been corroborated by Kupelian et al.,25 who demonstrated that the average absolute variation in intermarker distance was 1.01 ± 1.03 mm. Only 1% of the markers exhibited frequent movement, and it was found to be due to prostate deformation secondary to rectal filling.
Recently integrated into all contemporary linear accelerators, cone-beam computed tomography (CBCT) for online correction is gaining popularity as it allows for soft tissue matching, and does not require an invasive procedure to implant fiducial markers. Moseley et al.26 compared kilovoltage CBCT with fiducial markers and EPI and found no significant difference as a means for image guidance.
With the development of electromagnetic transponders (Calypso Medical Technologies, Seattle WA) that can be implanted into the prostate, real-time tracking at a rate of 10 times per second is now possible. Langen et al.27 described broadly two different types of intrafraction motions. One is by a slow, small drift, usually posteriorly and inferiorly, that is thought to be associated with pelvic muscle relaxation or the gradual movement of rectal contents away from the prostate. Second is a sudden and transient motion, usually anteriorly and superiorly, that can be significant in extent and is thought to be due to peristalsis. In the cohort of 17 patients (550 fractions), the average proportion of the total treatment time that the prostate was displaced by >3 mm and >5 mm was 13.6% and 3.3%, respectively. However, when analyzed by patient-to-patient basis, the prostate was displaced by 36.2% and 10.9%, respectively.
If the nodal target volumes are assumed to correlate with bony anatomy, pelvic IMRT that is set up to the prostate gland by intraprostatic fiducial markers could lead to significant underdosing given the lack of correlation between the prostate and bony anatomy. In a preliminary analysis of patients treated at UCSF, Chen et al.28 demonstrated that an isocenter shift of 5 or 10 mm in the superior direction could reduce nodal target coverage by 11% and 26%, respectively, with whole pelvic IMRT. Xia et al.29 compared multiple adaptive plans, isoshifting, and multileaf collimator (MLC) shifting and concluded that MLC shifting was the most effective in addressing the independent movement of the prostate and pelvic nodes during radiotherapy. Rossi et al.30 performed a retrospective dosimetric review of 10 high-risk patients, who had daily onboard imaging and correction of gold intraprostatic fiducial markers, and reported that overall, there was little difference (2% to 5%) between the planned dose and the delivered dose to the pelvic nodes by IMRT. However, two patients had a significant underdosing of 9% and 29% to the pelvic nodes that was thought to be due to a difference between the planning CT simulation scan and the daily treatments rather than random prostate motion. In a study by Chung et al.,31 patients treated with image-guided IMRT to the pelvic nodes had significantly lower dose to the rectum and bladder and fewer toxicities than those who received IMRT only.
FIGURE 66.2. Representative pelvic lymph node clinical target volume contours of: (A) common iliac and presacral at L5-S1; (B) external, internal, and presacral at S1-S3; (C) external and internal iliac below S3; (D) end of external iliac at top of femoral heads (bony landmark for the inguinal ligament); (E) obturator at the top of the pubic symphysis. (Adapted from Lawton CA, Michalski J, El-Naga I, et al. RTOG GU radiation oncology specialists reach consensus on pelvic lymph node volumes for high-risk prostate cancer. Int J Radiat Oncol Biol Phys 2009;74:383–387, with permission from Elsevier.)
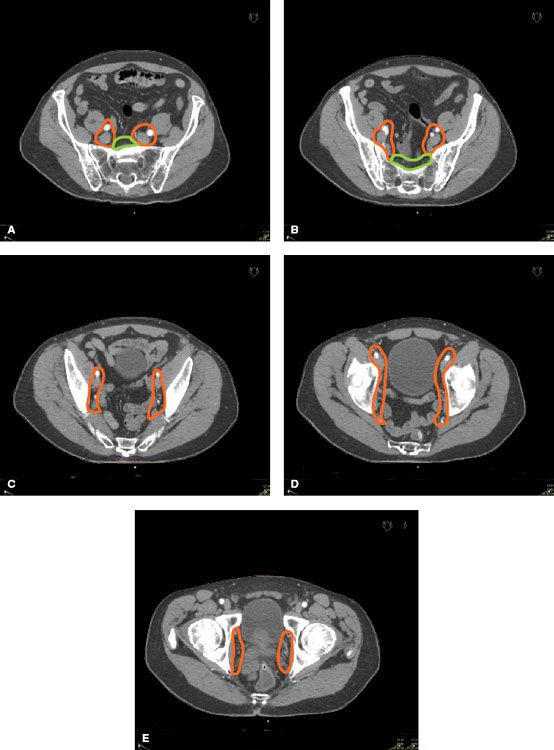
BIOLOGIC RATIONALE FOR PROPHYLACTIC PELVIC IRRADIATION
Incidence of Occult Lymph Node Involvement
The ability of current imaging techniques to detect involved nodes is hampered by their sole reliance on size criteria. As we will see, the incidence of clinically occult disease is unexpectedly high. This observation comes from several fronts, including more sophisticated assays, different imaging agents that have increased affinity to lymph nodes, and more extensive node dissection.
From the Baylor College of Medicine, Shariat et al.32 studied reverse-transcriptase polymerase chain reaction (RT-PCR) assay for human glandular kallikrein 2 (hK2) mRNA expression in histopathologically normal pelvic nodes in patients with pT3N0 prostate cancer. Of the 199 evaluable men, 20% and 40% had positive and equivocal results, respectively. In multivariate analysis, a positive RT-PCR/hK2 result was associated with PSA progression, development of distant metastases, and PCSM.
Surgical Results of Extended Pelvic Lymph Node Dissection
One of the current controversies erupting in the surgical literature is the role of an extended pelvic lymph node dissection. The traditional and most common approach is to do a limited dissection. Both procedures excise the fibrofatty and lymphatic tissues between the bifurcation of the common iliac artery superiorly to the femoral canal inferiorly and to the pelvic sidewall laterally. The critical difference between the two procedures is that in the limited dissection, the posterior extent is carried to the obturator nerve, whereas in the extended dissection, it is extended to include the obturator vessels and internal iliac vein. A second important consideration, when reviewing surgical studies, is whether only hematoxylin and eosin (H&E) staining was performed or more sensitive analyses were included, such as immunohistochemistry (IHC) or RT-PCR.
Bader et al.33 conducted a prospective study of the anatomic extent of pelvic nodal involvement in a cohort of 365 men who underwent an extended lymph node dissection and radical prostatectomy. The median number of nodes retrieved was 21. Despite using only H&E stains to evaluate the extracted nodes, 24% of patients had node-positive disease, of which 49% of them had a PSA >20 ng/mL. The internal iliac nodes, which are not usually dissected in a limited dissection, were involved in 58% of men with node-positive disease. Of note, 19% of node-positive men had involved nodes that were exclusively found in the internal iliac region, suggesting that the lymphatic drainage of prostate cancer is variable. The rate of problematic lymphocele was only 2%.
Heidenreich et al.34 reported on the incidence of lymph node involvement between standard and extended pelvic lymphadenectomy in 203 patients. IHC staining was performed if the H&E findings were negative. There were more dissected nodes in the extended dissection group (28 vs. 11; P <.01), at the expense of longer operative time (179 vs. 125 minutes; P <.03). There were more than twice the number of patients with positive nodes (26% and 12%; P <.03) in the extended dissection group, with 42% of all metastases lying outside the planes of a limited dissection. In patients deemed as high risk (Gleason score 7 to 10 and PSA ≥10.6 ng/mL) for lymph node metastasis, 60.9% of patients had histologically positive nodes. There was no difference in pelvic lymphocele or postoperative complications between the two groups.
In a large retrospective study from Johns Hopkins, the pathologic findings and biochemical outcomes were compared between two high-volume surgeons, each of whom exclusively performed either limited (n = 1,865) or extended (n = 2,135) node dissections during radical prostatectomy.35 As expected, the extended dissection group had more lymph nodes retrieved than the limited group (11.6 vs. 8.9 nodes; P <.0001). Yet the proportion of patients with involved nodes was also significantly higher in the extended dissection group (3.3% vs. 1.2%; P <.0001). When only patients with Gleason score 7 or 8 to 10 were considered, the difference was even more striking (8.2% vs. 2.4% and 23.2% vs. 8.9%, respectively). The relative risks of detecting a patient with involved nodes were remarkably similar, varying between 2.5 to 3 when adjusted by Gleason score, organ-confined status, seminal vesicle invasion, and surgical margin status. There was a trend in favor of the extended dissection group for 5-year biochemical recurrence-free survival (34.4% vs. 16.5%; P = .07). Among patients with <15% positive lymph nodes, the difference was more remarkable (42.9% vs. 10%; P = .01), suggesting a therapeutic benefit of an extended dissection in low volume disease. Clinically significant lymphoceles occurred in only 0.3% in the extended dissection group.
Using an intraoperative gamma probe and dynamic lymphoscintigraphy, Wawroschek et al.36 found that about a third of sentinel lymph nodes were in areas outside of a limited node dissection, such as the presacral, hypogastric, and pararectal regions. Holl et al.37 demonstrated the feasibility of using sentinel lymph node dissection via injection of radio-labeled nanocolloid into the prostate.
On the basis of these studies, the NCCN guidelines now recommend an extended lymph node dissection for all patients who have a predicted probability of lymph node metastases of >2% for both diagnostic and potentially therapeutic reasons.7 Although there have been numerous nomograms to predict nodal involvement, most are derived from surgical data where only limited node dissections were performed.38,39 Recently published nomograms by Briganti et al.39,40 help to address these limitations by using surgical data from patients who had an extended lymph node dissection to predict pelvic nodal involvement, exclusive nonobturator nodal metastases, and ideal nodal yield to accurately determine nodal involvement. Furthermore, it was recently demonstrated that the Roach et al.41 formula still held relevance when validated with a cohort of over 3,000 patients treated with radical prostatectomy and extended lymph node dissections.42 Although the accuracy of the Roach formula was found to be 80% in this cohort, Abdollah et al.42 found that applying the recommended cutoff of ≥15% to decided on whether to treat the pelvic nodes led to missing about a third of patients with lymph node involvement, and therefore they recommended that the threshold be lowered to 6%.
Novel Imaging Techniques
The role of lymphotropic superparamagnetic nanoparticles, given with MRI, has been recently reported.19,43 The nanoparticles are transported by lymphatic vessels, where they are filtered by lymph nodes. Lymph nodes that have been infiltrated by metastases will have distorted lymphatic flow and thus will accumulate the nanoparticles. Of the histologically involved nodes, 71.4% did not meet the MRI size criteria for malignancy.43 The sensitivity (90.5% vs. 35.4%; P <.001) and specificity (97.8% vs. 90.4%; P <.001) of MRI with superparamagnetic nanoparticles was significantly better than conventional MRI. When only nodes that were between 5 to 10 mm in diameter on the short axis were considered, the sensitivity increased from 28.5% to 96.4% (P <.001). Dinniwell et al.19 proposed a pelvic nodal modal based on nanoparticles and recommended CTV margins to the distal para-aortic (12 mm), common iliac (10 mm), external iliac (9 mm), and internal iliac (10 mm), drawn in continuity with a 12-mm expansion anterior to the sacrum and 22-mm expansion medial to the pelvic sidewall.
De Jong et al.44 reported on C-choline positron emission tomography (PET) for preoperative nodal staging of prostate cancer. In contrast to 18-fluorine-fluorodeoxyglucose (18F-FDG) PET, this radiolabeled agent does not suffer from radioactivity in the bladder, which can obscure nearby sites of metastases. The sensitivity, specificity, and accuracy were 80%, 96%, and 93%, respectively. In the 15 patients with histology-confirmed nodal disease, five patients had nodal disease in the common iliac region but otherwise had no involved nodes in the obturator region. In all five cases, 11C-choline PET correctly detected them.
Implications for Radiotherapy
The surgical series suggests that an extended node dissection, not surprisingly, yields more dissected nodes. Perhaps more surprising is that more involved nodes are detected—despite negative preoperative imaging studies—suggesting that the harder one looks, the more one finds. To further put this into perspective, these nodes are often found in areas (i.e., internal iliac and presacral nodes) that are traditionally not excavated by the more common limited node dissection, meaning that a significant proportion of higher-risk patients may have residual micrometastatic disease after surgery. The location of sentinel nodes have also been shown to be remarkably variable.36 Thus, some urologists have endorsed a more thorough node dissection in patients at higher risk of node involvement.34,45
The second important concept to draw from the surgical studies is that taken together, these studies suggest that minimal lymph node involvement detected by an extended lymph node dissection does not necessarily lead to inevitable relapse. It is not difficult to surmise that a patient with occult nodal disease who has been completely excised in an extended dissection would probably have a better outcome than the same patient with residual micrometastatic nodal disease after a limited dissection. Bader et al.33,45 prospectively studied the outcomes of 92 patients with node-positive disease after radical prostatectomy and extended node dissection that was initially staged as clinically organ-confined prostate cancer. After 45 months, 39% of patients with only one positive node as compared to only 12% patients with two or more positive nodes were free of biochemical recurrence (P = .008). Although follow-up was short, PCSM was significantly different (8% vs. 33%, respectively; P = .004). In the prospective study of node-positive patients after radical prostatectomy by Messing et al.,46 in the observation arm, 18% had no evidence of disease at a median follow-up of 7.1 years. Of note, these patients had a limited node dissection (median number of nodes retrieved was 12). The main criticism of this argument of improved outcomes with extended node dissection is that the effects of stage migration and lead time bias should not be discounted.
Collectively, these studies argue that an extended node dissection may yield a therapeutic benefit in the subgroup of patients with higher risk of nodal involvement yet have minimal nodal disease. This may in part be due to eradication of all tumor, but may also reflect the large individual variability of lymphatic drainage from the prostate gland.36 Can we extrapolate these results to pelvic radiotherapy? Is there a volume effect for pelvic radiotherapy as seen in the extent of lymph node dissection? The results from the RTOG-9413 study would suggest so.47
Although RTOG-9413 affirmed the role of whole-pelvic radiotherapy and neoadjuvant androgen suppression therapy (AST) in high-risk prostate cancer, there has been some lingering reluctance among radiation oncologists to deliver whole-pelvic radiotherapy, instead opting for “minipelvic” fields or omitting the pelvic field entirely.47 To reinforce this notion, secondary subgroup analysis of RTOG-9413 was recently presented, studying the volume dependence of outcome.48 Because of the disparity in the time point at which the neoadjuvant and adjuvant AST arms became susceptible to relapse (i.e., end of both radiotherapy and AST), only patients (n = 649) who received neoadjuvant AST were included in this analysis. After stratifying by volume irradiated, the 7-year progression-free survival was 40%, 31%, and 27% in the groups that received whole-pelvic, minipelvic, and prostate-only radiotherapy, respectively (P = .02; Fig. 66.3). There was no difference in acute grade ≥3 genitourinary or gastrointestinal toxicities among the three volumes. No difference was seen in the incidence of late grade ≥3 genitourinary toxicities between the whole-pelvic, minipelvic, and prostate-only radiotherapy groups at 48 months (3.0%, 2.4%, and 0%, respectively; P = .24). There was a small but significant increase in the incidence of late grade ≥3 gastrointestinal toxicities with larger fields at 48 months (4.3%, 1.2%, and 0%, respectively; P = .006). Of note, 3D conformal radiation therapy (3DCRT), let alone IMRT, was not routinely performed in this study. It is conceivable that with either modality, late toxicities may be further decreased.
Although sentinel lymph node dissections are standard in various disease sites like breast and melanoma, its application in prostate cancer is still under study. Holl et al.37 demonstrated that sentinel lymph node dissections using radiolabeled nanocolloid was reliable in detecting positive nodes in over 2,000 patients. Krengli et al.49 recently reported on the application of sentinel lymph node imaging (single-photon emission computed tomography [SPECT]) in pelvic radiotherapy for prostate cancer and found that 25% of patients had nodes outside their pelvic CTV. Similarly, Chen et al.22 reported on the feasibility of sentinel lymph node image-guided IMRT for prostate cancer and compared it to RTOG-based IMRT plans. Patients were injected with 99mTc-sulfur colloid into six prostate locations, then imaged with SPECT to generate a lymphatic drainage map. Although all patients had sentinel nodes identified in the internal or external iliac nodal basins, a startling 50% had sentinel nodes in the para-aortics, and RTOG consensus guidelines on pelvic delineation included all identified sentinel nodes in only 30% of cases. In comparative radiotherapy plans, there was no significant difference in dose to organs at risk.
FIGURE 66.3. Progression-free survival of subgroup analysis of Radiation Therapy Oncology Group RTOG-9413 comparing whole-pelvic, minipelvic. and prostate-only radiotherapy.(From Roach M III, DeSilvio M, Valicenti R, et al. Whole pelvic, “mini-pelvic” or prostate-only external beam radiotherapy after neoadjuvant and concurrent hormonal therapy in patients treated in the Radiation Therapy Oncology Group 9413 Trial. Int J Radiat Oncol Biol Phys 2006;66:647–653, with permission from Elsevier.)
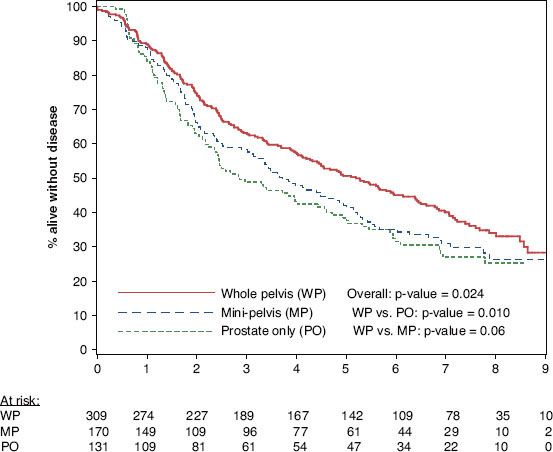
Radiation Oncology Trials
RTOG-9413 is a landmark trial that featured a two-by-two factorial randomization scheme to either prostate-only radiotherapy (PORT) or whole-pelvic radiotherapy (WPRT) followed by a prostate boost, and either 4 months of neoadjuvant and concurrent hormones or 4 months of adjuvant hormones.47 Patients with an estimated nodal risk of >15% or T2c to T4 with a Gleason score of ≥6 were eligible for the study. The 4-year progression-free survival was 54.2% and 47% in the combined WPRT arms and PORT arms (P = .02), respectively. When the four arms were analyzed separately, the neoadjuvant hormone and WPRT arm (59.6%) had a significantly better 4-year progression-free survival than the other three arms (44.3% to 49.8%; P = .008). Although there was no difference in acute (3% vs. 4%; P = .39) and late (2% vs. 2%; P = .85) grade 3 to 5 genitourinary (GU) morbidity, a trend toward increased acute (2% vs. 1%; P = .06) and late (1.7% vs. 0.6%; P = .09) grade 3 to 5 gastrointestinal (GI) morbidity was seen in the combined WPRT and prostate only arms, respectively.
Updated in 2007, there has been widespread confusion as to how to interpret the updated results, with some vocal pundits declaring that RTOG-9413 was now a “negative” study.50,51 Contributing to this confusion was the fact that when the four arms were combined into two groups based on volume irradiated (WPRT vs. PORT), the previously significant difference in progression-free survival was no longer seen (P = .99). When the four arms were analyzed separately, there was a trend toward significance for progression-free survival in favor of the WPRT and neoadjuvant hormone arm (NHT) as compared to PORT + NHT (P = .066), WPRT and adjuvant hormonal therapy (AHT) (P = .022), but not different than PORT + AHT (P = .75). Of note, the progression-free survival end point included death from any cause, and therefore with longer follow-up non–prostate cancer deaths may overwhelm cancer-related events. When analyzed for biochemical failure using only the Phoenix definition (discussed below), there was a significant difference among the four arms (overall P = .047) and a significant difference in pair-wise comparisons in favor of WPRT + NHT versus PORT + NHT (P = .0098). Finally, RTOG-9413 was designed well before the results of dose-escalation trials (see Section on dose-escalation later in this chapter) that demonstrated the superiority of increased radiation doses in terms of local control and biochemical control. Indeed, its prostate dose of 70 Gy would be considered woefully inadequate in the current era. Thus, these patients would be at significant risk of local relapse, which would presumably present as later recurrences relative to distant or regional recurrences. These distinct waves of recurrences may help to explain why the initial results of RTOG-9413 were positive, but then deemed negative in the updated results.
Late toxicities were reported in the updated results of RTOG-9413.50 Although there was no difference in grades 3 to 5 GU toxicities among the four arms (P = .16), there was a significantly greater incidence of grades 3 to 5 GI toxicities in the WPRT + NHT arm (5% vs. 1% to 2% in the other three arms; P = .002).
Preliminary results from GETUG-01 (Groupe d’Etude des Tumeurs Uro-Génitales), a French multicenter phase III trial, were recently published.52 It randomized 444 patients with T1b-T3N0M0 prostate cancer to WPRT or PORT. Four to 8 months of neoadjuvant and concurrent hormones was not part of the protocol treatment for all patients. Instead, patients were stratified based on estimated node involvement, and only those deemed to be high risk, defined as ≥T3, Gleason score ≥7, or PSA ≥3 times normal, for nodal involvement received hormones. The total prostate dose in the study was 66 to 70 Gy. With a median follow-up of 42 months, no significant difference was seen in progression-free survival between the WPRT and PORT arms for high risk (59.8% vs. 63.4%; P = .2) and low risk (83.9% vs. 75.1%; P = .21), respectively. Yet in multivariate analyses, risk of nodal involvement was the most significant predictor of progression-free survival. WPRT slightly increased acute mild GI toxicities and late grade ≥2 GI toxicities. Although the results of GETUG-01 seem to contradict that of RTOG-9413, there are significant differences in the methodology of the two studies that warrant mention. First, more than half of the patients (~54%) had an estimated nodal risk of <15% using the Roach formula,41 which meant that these patients had more favorable disease than those in RTOG-9413 and therefore would likely not benefit from WPRT. Second, the protocol-defined pelvic fields were smaller than those used in RTOG-9413. Specifically, the superior border was taken at the level of S1–2 interspace, rather than L5-S1 interspace, which meant that a significant portion of the presacral and common iliac nodes were not treated. Third, approximately 60% of patients received <70 Gy (RTOG-9413 mandated 70.2 Gy) to the prostate gland, which is considered suboptimal and may lead to increased risk of local recurrence.
One of the most common comments regarding RTOG-9413 is whether the beneficial finding of neoadjuvant hormones and WPRT still holds true in the dose-escalation era where doses of more than 80 Gy to the prostate alone can be given safely with IMRT. Retrospective data from Fox Chase Cancer Center were recently reported, investigating the effects of dose escalation, WPRT, and short-term hormones in the subgroup of patients who would otherwise be eligible for RTOG-9413 based on the inclusion criteria.53 Multivariate results suggest that radiation dose (70 to 72.9 vs. 73 to 76.9 vs. ≥77 Gy), PSA, clinical T stage, and Gleason score were significant predictors of biochemical failure, whereas short-term hormones and radiation field size were not. Although provocative, there are some shortcomings of this study, as pointed out by the authors, which merit closer attention. Although the inclusion criteria was identical to RTOG-9413, 42.4% and 31.4% of patients had a PSA <10 and 10 to 20 ng/mL (median PSA 10.95 ng/mL), respectively, whereas the median PSA in RTOG-9413 was 22.6 ng/mL. In addition, only 67 patients received neoadjuvant hormones. Radiation fields were prostate only, partial pelvic, and whole-pelvis fields in 11.4%, 17.6%, and 71%, respectively. In the latter group, the whole-pelvic field extended superiorly to the inferior sacroiliac joints, which would not be considered adequate pelvic irradiation according to RTOG-9413, where a minimum unblocked field size of 16-by-16 cm was used. Total radiation doses of up to 82 Gy were given in the cohort, although the group that received at least 77 Gy had a median follow-up of only 30 months (vs. 62 and 54 months in the <73 Gy and 73 to 76.9 Gy groups, respectively). Thus, because only 16% patients received NHT and none received WPRT with the potential for selection bias (patients treated with NHT may have had worse disease than those not receiving NHT), these data do not make a compelling argument against WPRT and NHT acquired from a large phase III trial.
Vargas et al.54 reported on the role of WPRT in a large cohort of patients treated at three institutions with external-beam radiotherapy and high–dose-rate brachytherapy. At the two German institutions, the policy was to give WPRT, whereas the American institution chose to irradiate the periprostatic area only (PORT). Of the 1,492 patients treated with this technique, 596 had an estimated nodal involvement of more than 15% based on the Roach formula,41 and they formed the study cohort. Fifty-one percent received neoadjuvant or adjuvant hormones for ≤6 months, and 53.3% received WPRT. As opposed to the PORT group, the median PSA was higher (15.6 vs. 11.5 ng/mL), although both groups had a Gleason score of 7 and clinical T stage was slightly worse (T2b vs. T2a) in the WPRT group. Rather than show no difference, the 5-year actuarial PSA failure was significantly worse in the WPRT group (31.2% vs. 16.8%; P <.001) as was clinical failure (17.6% vs. 7.9%; P = .01), suggesting an imbalance in predictors between the two groups. In multivariate analysis, Gleason score and clinical T stage were the only predictors of clinical failure, whereas PSA, use of hormones, WPRT, and estimated risk of nodal involvement were not. Thus, the authors concluded that WPRT may not be beneficial in those with >15% risk of nodal involvement. Yet there are elements of the results and interpretation that are problematic. First, the authors chose to report only the median clinical T stage, PSA, and Gleason score (without P values) rather than provide a more detailed breakdown between the WPRT and PORT groups. Second, the findings of RTOG-9413 suggest a synergistic effect between neoadjuvant and concurrent hormones and WPRT. But they did not suggest that WPRT without hormones was beneficial. Rather than compare a subset of the study cohort that exclusively received WPRT and neoadjuvant hormones, this retrospective study included patients who did not receive hormones in their WPRT group. Finally, the multivariate analysis included WPRT and hormones as separate covariates.
In a retrospective study from Yale, Aizer et al.55 analyzed the outcomes of 277 patients with an estimated nodal involvement of ≥15% who received either WPRT or PORT. The median prostate radiation dose was 75.6 Gy. Over 90% of patients in both groups received neoadjuvant and concurrent hormonal therapy. Median follow-up was only 30 months. Despite more advanced T stage, Gleason score, and pretreatment PSA, 4-year biochemical control was improved in the WPRT over the PORT group (86.3% vs. 69.4%). On multivariate analyses, WPRT was a significant predictor of biochemical control, along with Gleason score, pretreatment PSA, and the use of hormones.
Given the clinical equipoise stemming from the controversies of WPRT, in July 2011, RTOG activated its RTOG-0924 study that includes patients with unfavorable intermediate- and favorable high-risk disease, and sought to study whether the addition of WPRT could improve overall survival in patients receiving dose-escalated radiotherapy and hormones. Eschewing the simplistic NCCN risk groupings,7 RTOG-0924 identified a subset of patients from RTOG-9413 who benefited from WPRT and incorporated the percentage of positive biopsy cores into the eligibility criteria. Patients eligible for this study should have an estimated nodal risk of at least 15% and have either:
• Gleason score 7 to 10 and T1c-T2b and PSA <50 ng/mL
• Gleason score 6 and (T2c-T4 or >50% biopsies) and PSA <50 ng/mL
• Gleason score 6 and T1c-T2b and PSA >20 ng/mL.
The phase I dose is 45 Gy in both arms and can be delivered with 3DCRT or IMRT. The phase II prostate boost can be achieved by IMRT (34.2 Gy; total dose 79.2 Gy), low–dose-rate brachytherapy (110 Gy for iodine-125 [125I] and 100 Gy for palladium-103 [103Pd]) or high–dose-rate brachytherapy (15 Gy in one fraction with iridium-192 [192Ir]) were permitted. Patients will be stratified into 6- or 32-months of androgen suppression therapy.
Summary of University of California–San Francisco Recommendations
Whole-Pelvic Radiotherapy
At UCSF, the authors recommend prophylactic whole-pelvic IMRT to patients with an estimated nodal involvement of >15% and unfavorable intermediate- and high-risk prostate cancer. The authors recommend adherence to the RTOG GU Radiation Oncology consensus statement on pelvic nodal volumes.21 The prostate boost is delivered with either IMRT, 125I permanent seed or high–dose-rate (HDR) brachytherapy, although the authors prefer the latter approaches because of their ability to deliver maximal dose to the prostate. Brachytherapy is typically performed 1 to 2 weeks following pelvic radiotherapy. For T3 disease, the authors use an IMRT or HDR boost, which allows better coverage of extranodal disease.
Androgen Suppression Therapy
The authors give 2 months of neoadjuvant, then concurrent total androgen blockade using an luteinizing hormone-releasing hormone (LHRH) agonist and an antiandrogen. Two to 3 years of adjuvant hormones (only an LHRH agonist) is recommended for high-risk disease, but on occasion lifelong androgen deprivation is used in patients with very high-risk disease (Table 66.1).
TABLE 66.1 RECOMMENDATIONS OF PELVIC RADIOTHERAPY AND HORMONES
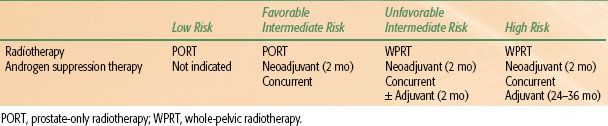
Volumes and Setup Variation
The authors routinely place three fiducial gold seed markers into the prostate under transrectal ultrasound guidance—two in the base and one in the apex. Each marker is approximately 1.1 mm in diameter and 3 mm in length. Using an amorphous silicon flat-panel detector, the authors perform daily EPI and make any necessary adjustments prior to each treatment. As such, the authors apply a 3-mm margin around the prostate gland and seminal vesicles in defining the PTV. For patients with hip replacements, the authors use cone-beam CT for image guidance. At institutions without daily EPI to control for intra- and interfraction prostate motion, larger margins such as 0.5 to 1 cm should be considered.
Patients are instructed to empty their rectum with an enema prior to simulation. Patients are told to maintain a full bladder during simulation and treatment. A retrograde urethrogram is performed to assist in identifying the base of the prostate. Three-millimeter slice thickness is used for the CT simulation. The critical organs that are contoured include the penile bulb, small bowel, rectum, bladder, and femoral heads. The entire rectum is contoured from the anus to the rectosigmoid junction.
Intensity-Modulated Radiotherapy Treatment Planning
For patients opting for definitive external-beam radiotherapy to the pelvis and prostate, the authors use a two-phase plan. The first phase delivers 25 to 30 fractions of 1.8 Gy per fraction (total 45 to 54 Gy) to the nodes, and 2 Gy per fraction (total 54 Gy) to the seminal vesicle and prostate PTV. The second phase is a cone-down boost to the prostate PTV alone with 11 fractions of 1.8 Gy per fraction. Therefore, the minimum total dose to the prostate (prescribed to the PTV) is 73.8 Gy. The usual isodose line that the authors prescribe to is approximately 90%, meaning that the maximum dose in the prostate is approximately 82 to 84 Gy. Both phases use a seven-field isocentric technique with 18-MV photons.
For pelvic radiotherapy followed by a brachytherapy boost, the authors deliver 25 fractions of 1.8 Gy per fraction to the nodes and prostate or seminal vesicle PTV (total dose 45 Gy). In postoperative patients opting for pelvic radiotherapy, the authors prescribe 25 fractions of 1.8 Gy to the nodes (total dose 45 Gy) and 2 Gy per fraction to the tumor bed PTV (total dose 50 Gy) then 9 fractions of 1.8 Gy per fraction to the prostate bed (total minimal PTV dose 66.2 Gy, maximum dose 78 Gy).
Toxicity of Pelvic Intensity Modulated Radiotherapy
In a study from Memorial Sloan-Kettering Cancer Center, the dosimetric outcomes of pelvic radiotherapy using two-dimensional (2D), 3DCRT, and IMRT were compared in 13 patients.56 The mean bowel dose was reduced by approximately 20% with 3DCRT and IMRT as compared to 2D planning (P = .001). Similarly, the bowel V45 was 41.7%, 22.6%, and 9.1% with 2D, 3DCRT, and IMRT. Rectal sparing was also evident with IMRT and 3DCRT. The mean dose was 40.4, 37.3, and 27.3 Gy, respectively. The V25 was significantly better with IMRT (50.5%) as compared to 2D (91.7%) and 3DCRT (86.3%). Bladder V45 was significantly better with IMRT (87.2, 56.8, and 25.6%, respectively; P <.001). Toxicity was minimal with no cases of acute grade 3 to 5 toxicities. Only one patient required medication for proctitis and none for diarrhea.
Jani et al.57 retrospectively compared 15 patients who received pelvic IMRT versus 34 patients who received pelvic 3DCRT. The bladder (60.8% vs. 24.8%; P = .04) and rectal (65% vs. 25.1%; P = .01) V60 were significantly lower in the IMRT plans. The IMRT patients had significantly less acute GU but no difference in GI toxicities. Grade 3 (3% vs. 0%) and grade 2 GU (59% vs. 20%; P <.001) toxicities were less with IMRT.
Chung et al.31 analyzed the dosimetry and acute toxicities of 25 high-risk patients who received pelvic radiotherapy and received either IMRT (weekly portal images) or image-guided (IG) IMRT using intraprostatic fiducial markers. Planning target volume margins differed significantly between the two groups (0.5 to 1.0 cm for IMRT vs. 0.2 to 0.3 cm for IG-IMRT). As expected, bladder and rectal doses were significantly less with IG-IMRT, which translated to significantly less RTOG grade 2 rectal (80% vs. 13%; P = .004) and bladder (60% vs. 13%; P = .014) toxicities. No grade 3 to 5 toxicities were observed.
DOSE ESCALATION
Perhaps the most polarized topic in prostate radiotherapy management is whether pelvic radiotherapy or hormonal therapy is needed in the setting of dose escalation. The crux of the argument for dose escalation in intermediate- and high-risk patients is that the relatively poor outcome observed in such patients may be due to inadequate doses of radiotherapy to the prostate itself, as suggested by postradiotherapy biopsy data, rather than occult metastatic disease. Therefore, some contend that dose escalation to the prostate obviates the need for pelvic radiation or hormonal therapy. Dose escalation can be achieved by either external-beam radiotherapy alone, or in combination with prostate brachytherapy, with the latter capable of delivering a much higher radiation dose.
External-Beam Radiotherapy Alone
To date, there have been five phase III trials comparing conventional-dose with high-dose radiotherapy. Although all have demonstrated an improvement in biochemical control, no survival advantage has been observed despite long-term follow-up. As expected, grade 3 GI toxicities appear to be worse with dose escalation.
The MD Anderson Cancer Center conducted a single-institution dose-escalation phase III trial comparing 70 with 78 Gy to the prostate.58 Radiotherapy was conventionally planned in the 70-Gy arm and in phase I of the 78-Gy arm; a conformal boost plan was used in the 78 Gy arm. No hormonal therapy was used. With a median follow-up of 9 years, recently updated results continue to demonstrate a significant improvement with 78 Gy in 10-year PSA control of 74% versus 43% (P = .013) in patients with a presenting PSA >10 ng/mL.59 Moreover, with longer follow-up, the high-dose arm now conferred a reduction in 10-year nodal (P = .03), distant failures (P = .016) and death from prostate cancer (3% vs. 15%; P = .03) but not overall survival. The 10-year incidence of grade ≥2 GI toxicities was 13% versus 26% (P = .013), and grade 3 GI toxicities was 1% versus 7% (P = .018) in favor of the conventional-dose arm. There was no difference in GU toxicities.60
The Proton Radiation Oncology Group (PROG-9509 is a phase III trial of 393 men, comparing 19.8 gray-equivalent (GyE) photon with 28.8 GyE proton boost to the prostate.61 All patients then received 50.4 Gy using photons to the prostate and seminal vesicles with 3DCRT. In the cohort, 32% and 8% had intermediate- and high-risk disease, respectively. Updated results were recently reported with a median follow-up of 8.9 years.62 The 10-year PSA control rates were 68% versus 82.6% for conventional- and high-dose radiotherapy, respectively (P <.001). Dose escalation clearly benefited patients with low-risk disease (hazard ratio [HR] 0.22; P <.0001) and was of borderline significance among intermediate-risk patients (HR 0.58; P = .06). The lack of dose response seen in the high-risk group may have been due to the trial limiting the accrual to a PSA <15 ng/mL and T1b to T2b stage disease. To date, there is no survival benefit from high-dose radiotherapy. Acute and late GU and GI toxicities were not significantly increased with dose escalation.
The UK Medical Research Council (MRC) recently presented the initial results of RT01, a multicenter phase III trial that randomized 843 patients to conventional-dose (64 Gy) or dose-escalated (74 Gy) 3DCRT to the prostate.63 Both arms received neoadjuvant androgen therapy (3 to 6 months). With a median follow-up of 63 months, the 5-year biochemical control rates were superior in the dose-escalated arm.
The results of GETUG-06, a French multicenter randomized trial comparing 70 Gy with 80 Gy, were recently reported.64 Hormonal therapy was not given. All patients received 3DCRT to the prostate and seminal vesicles (46 Gy) then a prostate-only boost to either 24 or 34 Gy. Median follow-up was 61 months. Among the 306 patients accrued, the 5-year freedom from relapse rates were 67.9% versus 76.5% (P = .09). In subgroup analyses, a significant biochemical control difference was primarily noted to be among those with an initial PSA level >15 ng/mL (HR 0.52). A trend toward increased acute rectal and urinary toxicities was seen in the 80-Gy arm. Overall, late urinary toxicities were significantly increased in the 80-Gy arm. Although there was no significant difference in overall late rectal toxicities, grade 3 toxicities were significantly >80 Gy (1.5% vs. 6.5%; P = .047).
In the Dutch multicenter CKVO96–10 study by Peeters et al.,65 669 men were randomized to 68 Gy versus 78 Gy to the prostate only using 3DCRT. Hormonal therapy was permitted and left to the discretion of the treating physician. Overall, 22% of patients received neoadjuvant or adjuvant hormones. Updated results were recently published after a median follow-up of 70 months.66 The 7-year freedom from failure was significantly improved in the 78-Gy arm (45% vs. 56%; P = .03), but no survival difference was seen. Although not powered to do so, subgroup analyses suggested a significant benefit among intermediate-risk patients (odds ratio 0.6; P = .01) and borderline significance among high-risk patients, but not in the low-risk group. The 78-Gy arm had significantly higher rates of rectal bleeding, requiring laser or transfusion (3% vs. 8%; P = .01) and fecal incontinence (7% vs. 13%; P = .02) at 7-years. There was no difference seen for GU toxicities.
Zelefsky et al.67 updated the results a single-institution phase I and II study of dose escalation from 66.0 to 86.4 Gy to the prostate alone. Of note, radiotherapy was delivered by 3DCRT or IMRT. Of a total cohort of 2,047 patients, there were 849 and 752 patients with intermediate- and high-risk disease, respectively. Approximately half of the patients received 3 months of neoadjuvant hormones with the aim of cytoreduction or for those with high-grade, unfavorable-risk disease. Median follow-up was 6.6 years. In the intermediate-risk group, doses of ≥75.6 Gy yielded significantly better PSA control rates than doses ≤70.2 Gy (P <.0001; HR 0.707). In the high-risk group, 86.4 Gy improved 5-year biochemical control as compared with 75.6 Gy (P = .05). Among the intermediate- and high-risk patients, higher doses were significant predictors of distant metastases-free survival. Postradiation biopsies taken at 2.5 years seemed to support the contention that dose escalation was efficacious, even among intermediate- and unfavorable-risk patients. Whereas only 10% and 23% of patients who received 81 and 75.6 Gy, respectively, had positive biopsies, 34% and 54% of patients who received 70.2 Gy and 64.8 Gy, respectively, had positive biopsies. The overall grade 3 late rectal toxicity was 1%. In the cohort who received 75.6 Gy or greater using 3DCRT, grade 2 late rectal toxicity was 14%. Grade 3 urethral stricture or hematuria was seen in 1.5% of patients. The 5-year rate of grade 2 late urinary toxicity was 13%.
In a separate provocative study by Zelefsky et al.68 distant metastasis (DM)-free survival and cause-specific survival (CSS) were compared between radical prostatectomy (RP) and IMRT while controlling for differences in pretreatment characteristics by including the Kattan biochemical control nomogram69 and NCCN risk group classification.7 The 8-year freedom from metastatic progression was 97% for RP and 93% for IMRT (P <.001). Among intermediate- and high-risk patients, the adjusted absolute difference in DM-free survival was 3.3% and 7.8%, respectively. CSS was similarly better for RP than IMRT (P = .15). Despite euphoria from our surgical colleagues, it must be tempered by the notable limitations of this study. First, the IMRT group was severely handicapped by the lack of elective nodal irradiation, whereas extended node dissection was routinely performed in the RP arm. Second, none of the IMRT high-risk patients received adjuvant hormones, which has been shown in all phase III trials (albeit lower radiotherapy doses) to date to confer a clear survival advantage. Third, salvage therapies were given far less in the IMRT group than the RP group (43% vs. 76%) and far later (69 vs. 13 months), respectively. Salvage treatment for the IMRT group was overwhelmingly with hormones, which offers not even a glimmer of cure, whereas most in the RP group received salvage radiotherapy. Salvage brachytherapy after IMRT failure was not an option in this study. Finally, the nomogram chosen in this study found that PSA was far more predictive than clinical stage and Gleason score, whereas another nomogram specifically for the development of bone metastases70 concluded the opposite.
Long-term toxicities of RTOG-9406, a phase I and II trial (n = 1,084) of sequential dose levels (68.4, 73.8, 79.2, 74, and 78 Gy) using 3DCRT, were recently reported. Michalski et al.71 concluded that 79.2 Gy given in 1.8 Gy per day was the maximally well-tolerated dose. Toxicities were significantly higher with 78 Gy given in 2.0 Gy per day than the 79.2 Gy dose level. The follow-up study is RTOG-0126, a phase III multi-institutional trial that is comparing 70.2 Gy with 79.2 Gy using 3DCRT or IMRT in intermediate-risk disease, which recently completed accrual.
Of the five discussed randomized controlled trials, only the MRC RT01 and Dutch CKVO96–10 permitted hormones. In the case of the MRC study, both arms received hormones, and yet dose escalation was still beneficial. This suggests that dose escalation alone cannot replace hormones as a strategy to improve cure, and that both may be necessary in patients with intermediate- and high-risk disease. Recently, RTOG activated its RTOG-0815, which is a phase III multicenter trial evaluating the addition of 6-months of androgen blockade with dose-escalated radiotherapy, achieved by either 3DCRT or IMRT (79.2 Gy), combined low–dose-rate (110 Gy with 125I or 100 Gy with 103Pd) brachytherapy boost with 3DCRT or IMRT (45 Gy to the prostate and seminal vesicles), or combined high–dose-rate (2 fractions of 10.5 Gy per fractions) 3DCRT or IMRT (45 Gy to the prostate and seminal vesicles).
External-Beam Radiotherapy with Brachytherapy Boost
According to the 1999 Patterns of Care Study of radiotherapy and brachytherapy, 46% of prostate cancer patients who received brachytherapy also received supplemental external-beam radiotherapy (XRT).72 Rather than using XRT alone, where prostate doses are limited by nearby critical organs, many have combined the advantages of dose delivery by brachytherapy with the ability to irradiate a larger volume (e.g., periprostatic or whole pelvis) with XRT that would otherwise be beyond the reach of brachytherapy. Various centers have reported using low–dose-rate (LDR) brachytherapy, whereas others have used high–dose-rate brachytherapy.
Notwithstanding the downsides that brachytherapy is an invasive procedure that requires anesthesia, additional resources, and expertise, the advantages of incorporating prostate brachytherapy as a means of boosting the dose to the prostate gland are compelling. First, the achievable biologically effective dose (BED) to the prostate far exceeds what can be achieved by IMRT, which translates into higher cure rates. Second, brachytherapy presents as a superior method of minimizing radiation to nearby critical structures, and therefore toxicities have not been significantly greater than for IMRT. Third, the overall treatment time is usually less than the usual 8 weeks of conventionally fractionated XRT and therefore more convenient for patients. Fourth, the individual unpredictable nature of prostate motion, which continues to be vexing to radiation oncologists, is not an issue with brachytherapy.
As compared to LDR brachytherapy, HDR yields the following advantages: flexibility in source positioning, accurate source positioning, immobilized target, stable geometry, adaption of dose to target and healthy organs, target volume–dose optimization, high-quality planning and dose distribution, no radiation exposition to health care personnel or public, no source preparation needed, reduced cost, and possibly more effective prostate cancer cell kill. The disadvantages of HDR are that because the dose rate is higher it is often fractionated and therefore requires more workload and careful coordination from the health care personnel.
The indications of combined XRT and brachytherapy boost are those with intermediate- or high-risk prostate cancer, in particularly patients who are relatively young and have high-volume disease. Patients not eligible for brachytherapy boost include those with a large prostate (>60 cc), prior transurethral resection of the prostate and significant urinary symptoms.
Outcomes
The Seattle Prostate Institute reported on the biochemical outcome of 232 consecutively treated patients with pelvic radiotherapy (45 Gy) followed by an 125I (108 Gy) or 103Pd (100 Gy) brachytherapy boost.73 No hormones were given in this cohort. Overall, the 15-year biochemical relapse-free survival was 74%. Fifteen-year biochemical control was 86%, 80%, and 68% in the low-, intermediate-, and high-risk groups, respectively.
From McMaster University, 104 patients with T2 and T3N0 disease were randomly allocated to brachytherapy plus XRT or XRT alone.74 The XRT-only arm received 66 Gy, whereas the brachytherapy arm received 35 Gy using 192Ir seeds followed by XRT of 40 Gy (total dose 75 Gy). No adjuvant hormones were given. After a median follow-up of 8.2 years, the 5-year biochemical failure rate was significantly less in the brachytherapy arm. At 2 years, positive biopsies were significantly less in the brachytherapy arm (24% vs. 51%; P = .015). There was no difference in acute and late GI, GU, or sexual morbidity between the two arms.
Guix et al.75 recently reported on early results of a randomized trial comparing dose-escalated external-beam radiotherapy (76 Gy) with combined external-beam radiotherapy (46 Gy) and 2 fractions (8 Gy each) of HDR brachytherapy with 192Ir in patients with intermediate- or high-risk prostate cancer. After a median follow-up of 77 months, 5-year PSA relapse-free survival for intermediate- and high-risk patients in the external-beam radiotherapy only arm was 90% and 89%, respectively, whereas it was 97% and 96%, respectively, in the combined arm (P <.05). There were no grade 3 or 4 rectal or urinary toxicities observed in either arm. This study is of particular interest as it uses contemporary external-beam radiotherapy doses, unlike other studies, and is a prospective randomized trial.
In a sequential prospective study by Morton et al.,76 HDR brachytherapy boost (10 Gy é 2 fractions, given 1 week apart) with conventional fractionation (45 Gy in 25 fractions) was compared to single-fraction HDR brachytherapy boost (15 Gy; Fig. 66.4) with hypofractionated external-beam radiotherapy (37.5 Gy in 15 fractions) in patients with intermediate-risk prostate cancer. The hypothesis was that late effects, assuming an αβ ratio of 3, were equivalent between the two fractionations, and that disease control rates were comparable. With increasing reports that prostate cancer may have an αβ ratio of <3, the hypofractionated arm would theoretically confer improved tumoricidal doses.77–79 Preliminary data show no difference in 5-year PSA control rates between the single-fraction (95.1%) and two-fraction boost (97.9%; P = 0.35), although median follow-up differed at 45 versus 72 months, respectively. Two-year prostate biopsy was positive in 4% and 8%, respectively. Although acute grade 3 GU toxicity (i.e., acute urinary retention requiring catheterization) was significantly greater in the two-fraction boost group (1.6% vs. 20%; P = .0005), there was no difference in late grade 3 to 5 GU or GI toxicities as measured by the Common Terminology Criteria for Adverse Events (version 3.0), serial International Prostate Symptom Score, or Expanded Prostate Index Composite.
From UCSF, Kaprealian et al.80 compared the outcomes of 165 patients treated with HDR brachytherapy boost using two different HDR fractionation schemes (18 Gy in 3 fractions and 19 Gy in 2 fractions). All patients received an initial 45 Gy to the prostate and seminal vesicles for those with an estimated risk of nodal involvement of ≤15% or the same dose to the pelvic nodes for those with >15% risk of nodal involvement. Patients received 4 months of neoadjuvant and concurrent hormones, except those with high-risk disease who received an additional 24 months of adjuvant hormones. There was no significant difference in overall 5-year PSA control rates between the 3- and 2-fractions HDR groups (93.5% vs. 87.3%; P = .19), despite the 2-fraction group comprising more patients with Gleason scores of 8 to 10. In the entire cohort, there were only three grade 3 GU toxicities and no grade 4 or 5 toxicities. Only one patient developed a stricture.
Hoskin et al.81 presented preliminary results of their phase III trial comparing external-beam radiotherapy alone (55 Gy in 20 fractions) with combined external-beam radiotherapy (35.75 Gy in 13 fractions) followed by HDR prostate brachytherapy (17 Gy in 2 fractions over 24 hours) among 220 patients with clinically localized prostate cancer. With a median follow-up of 30 months, the combined arm had significantly improved PSA relapse-free survival (P = .03). There was no difference in acute GU and GI toxicity scores, except for rectal discharge, which was more frequent in the external-beam radiotherapy arm (P = .025). No difference was observed in late GU and GI grade 2+ toxicity scores.
At William Beaumont Hospital, Martinez et al.82 published the long-term results of their phase II study of hypofractionated dose-escalated radiotherapy in 472 patients with intermediate- and high-risk disease. All patients received 46 Gy in 23 fractions to the pelvis with external-beam radiotherapy. The HDR brachytherapy procedure was delivered over 2 or 3 separate fractions during weeks 1 and 3 of the external-beam radiotherapy, for a total overall treatment time of 5 weeks. The HDR brachytherapy dose fractionation was increased in successive dose bins of 3 implants of 5.5 Gy, 6.0 Gy, and 6.5 Gy, and 2 implants of 8.25 Gy, 8.75 Gy, 9.5 Gy, 10.5 Gy, and 11.5 Gy. Assuming an αβ ratio of 1.2, the HDR BED ranged from 92.13 to 243.42 Gy, and the total BED including the external-beam radiotherapy ranged from 215 to 366 Gy. For the purposes of the study, patients were separated into a low-dose-level (BED ≤268 Gy) and a high-dose-level (BED >268 Gy). The use of hormones was based on the treating physician’s discretion, and in the study, 51.3% received neoadjuvant or concurrent hormones. Median follow-up was 8.2 years. At 10-years, the biochemical control rate (56.9% vs. 81.1%; P <.001) and distant metastasis-free rate (87.6% vs. 94.3%; P = .028) were superior in the high-dose-level group.
FIGURE 66.4. Isodose lines and dose–volume histogram from a representative patient treated with single-fraction 15 Gy high–dose-rate prostate brachytherapy with ultrasound-based planning. Twelve catheters were implanted. On dose–volume histogram, the prostate (red line), urethra (blue line), and rectum (brown line) are represented. (Courtesy of Gerard Morton, MD, Sunnybrook Odette Cancer Centre, University of Toronto.)
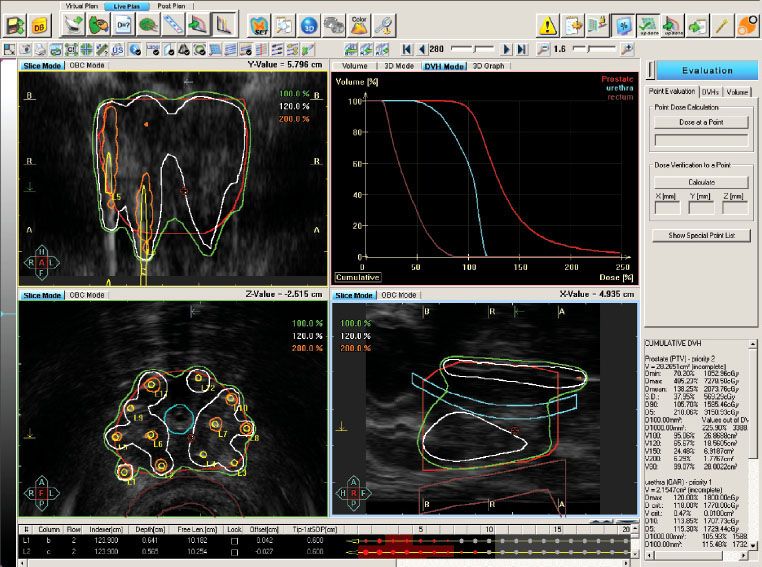
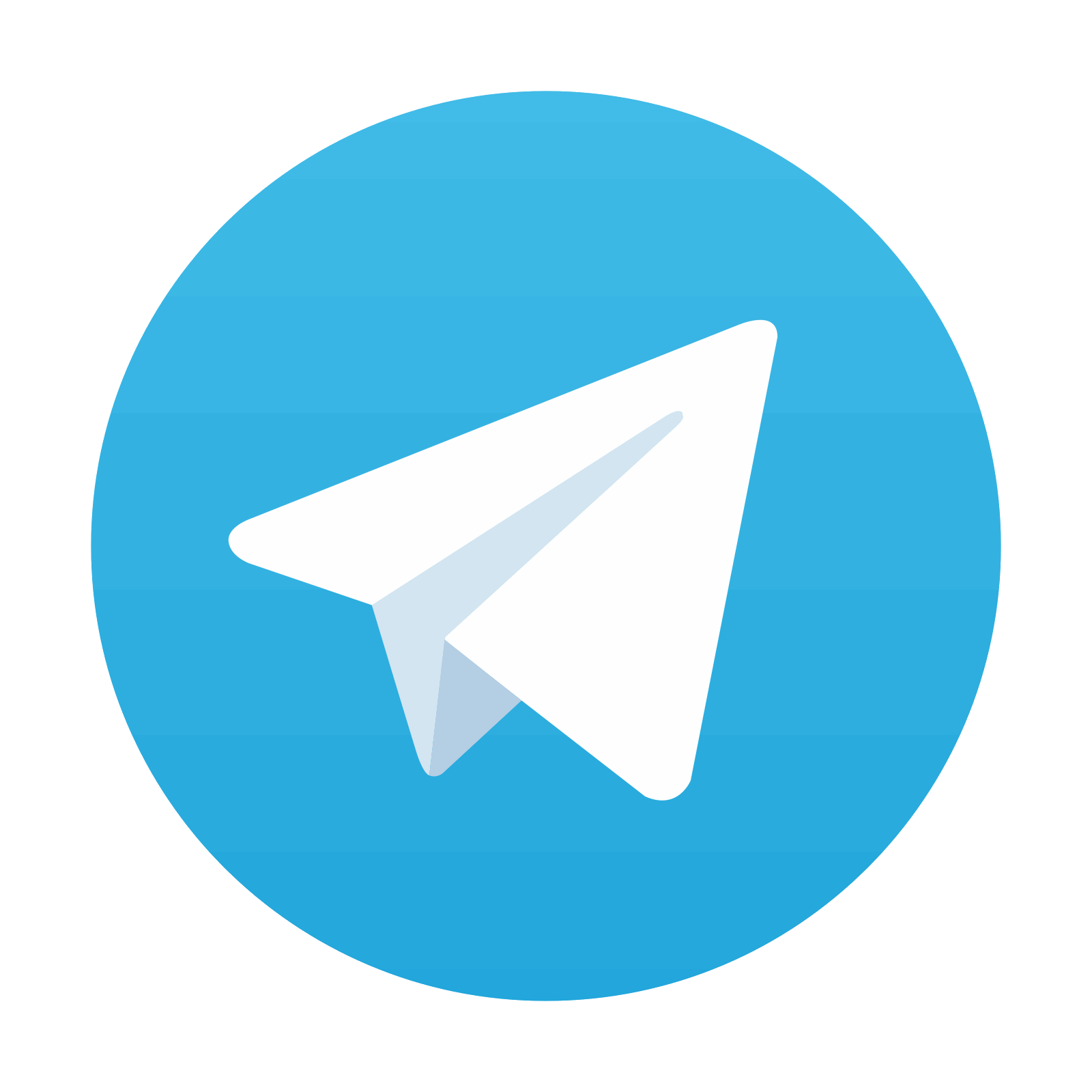